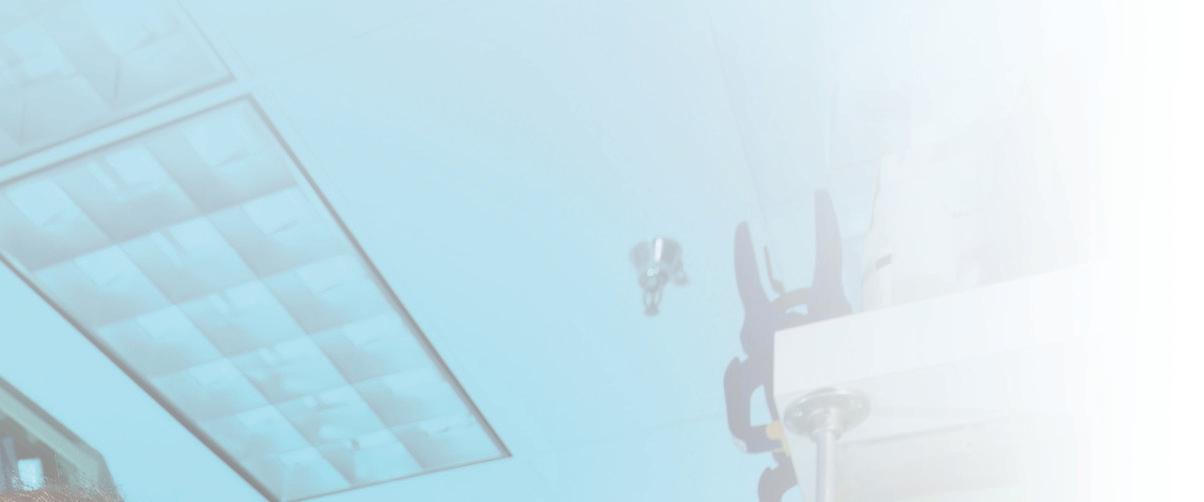
8 minute read
industRializing the pRoduction of steM cells
Industrializing the Production
oF STeM ceLLS
Advertisement
Stem cells have the potential to yield a readily available, consistent source of many differentiated cell types. This unique property can be leveraged for therapeutic purposes and for facilitating and improving a number of drug discovery and development processes such as toxicity screening (Figure 1).
Large-scale “industrialized” production of stem cells is necessary to enable their advancement into human clinical trials and to effectively deliver the quantities needed for drug discovery screening and lead optimization. However, achieving this level of production while meeting rigorous quality standards will depend on further progress in the areas of cell culture and scale-up, characterization, enrichment and purifi cation to deliver a consistent and reproducible supply of cells.
Large-scale cGMP Production of Stem Cells
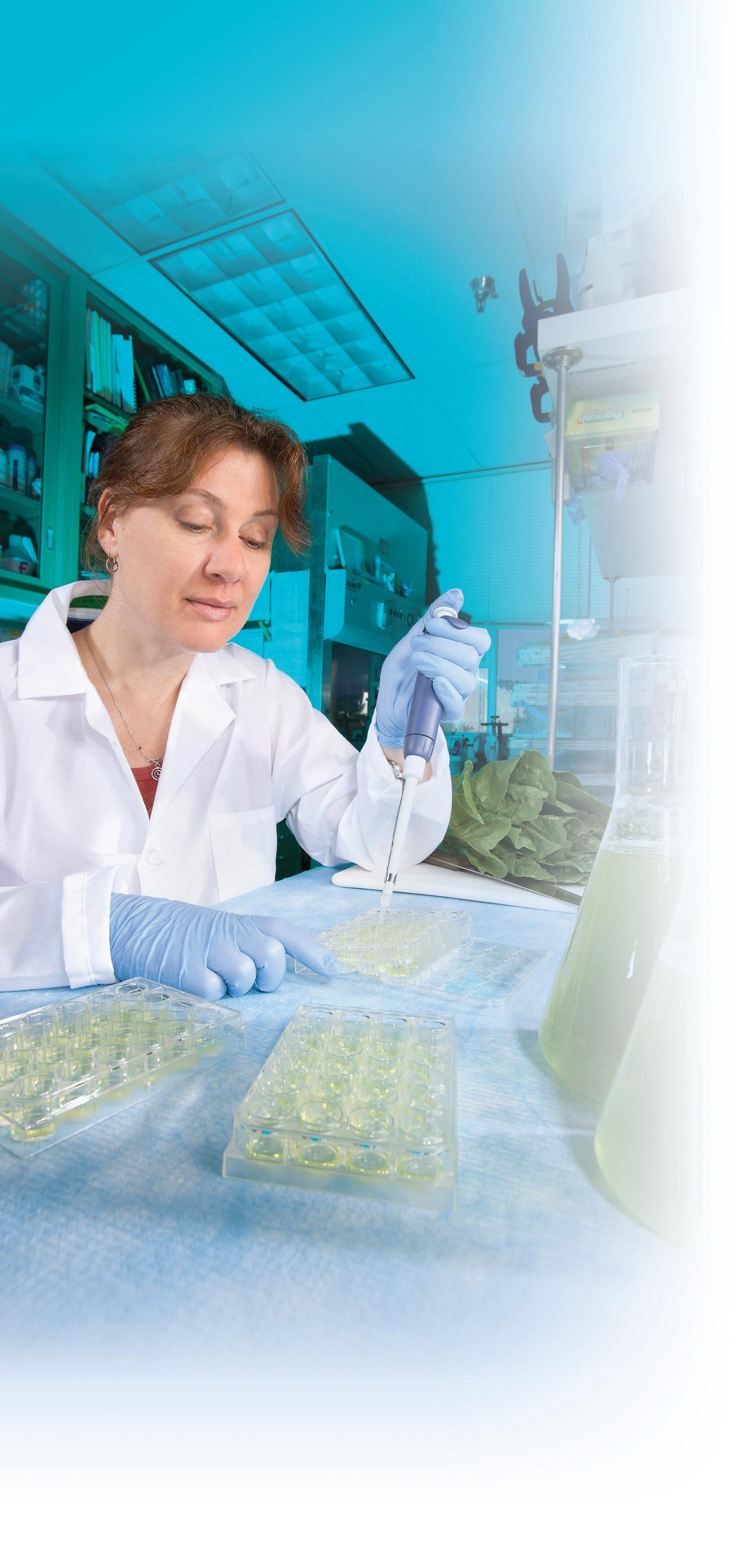
Advancement of stem cell-based therapeutics into clinical trials requires well-characterized cells produced under tightly controlled, consistent, reproducible culture conditions that adhere to Current Good Manufacturing Practice (cGMP) standards (Figure 2). cGMP standards, established by the FDA, cover the manufacture and testing of pharmaceutical products and dictate that processes are clearly defi ned, validated, and controlled to ensure consistency and compliance with specifi cations. cGMP stem cell culture systems will need well-defi ned, optimized media and supplements to support stem cell expansion and differentiation. The use of effi cient, standardized methods
Figure 1: Large-scale production of stem cells is necessary to support development of therapeutic applications and drug discovery and development applications.

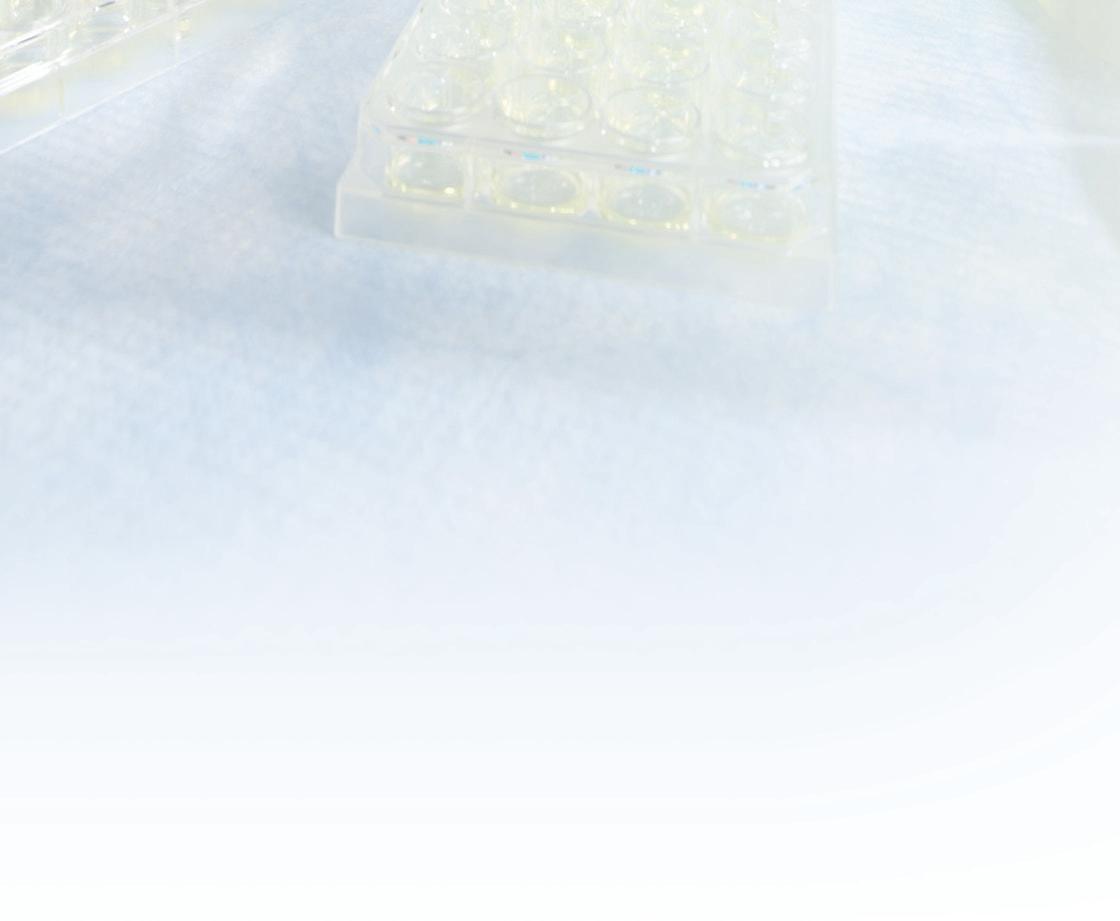
Figure 2: Bridging the gap between basic research and large scale manufacturing of stem cells requires well controlled, reproducible methods.
for growing and harvesting cells will ensure consistent cell populations with uniform properties and predictable behaviors.
cGMP Culture Supplements When used for basic research applications, stem cells are typically grown in small-scale tissue culture flasks using media and culture supplements (e.g. growth factors) that are not always fully defined or characterized and, in some cases, of animal origin.
Human embryonic stem cell cultures were originally grown on “feeder layers” of mouse fibroblast cells. While the soluble factors secreted by the mouse cells help provide the proper environment for stem cell proliferation, use of feeder layers or co-culture systems have significant drawbacks when culturing stem cells for clinical applications. Furthermore, the use of undefined matrices used for adherent cells is also undesirable.
As more stem cell-based therapeutics progress towards clinical testing, the consistency, quality, and reproducibility of largescale culture systems become imperative. When manufactured under cGMP conditions, supplements and cell-binding matrices enabling robust proliferation of stem cells in culture will be required. Use of cGMP supplements contributes to high quality, consistent, reproducible culture conditions.
Integrated Cell “Manufacturing” Systems
A number of challenges exist across the scaleup process for generating large batches of stem cells for therapeutic use (Figure 3). Because the cells themselves are the “product,” culture systems must: minimize variability; effectively control differentiation; enable harvesting and formulation without damaging cells; and incorporate processes to ensure cell viability during storage, transport, and administration to the patient.
Large-scale production of stem cells will require fully integrated, scalable systems that include: • New microcarrier technology or alternative solutions that enable particulate-free culture of stem cells in a bioreactor. When cultured in bioreactors, adherent stem cells must be grown on a solid surface such as small beads (called “microcarriers”). However, small particulates or “fines” are often generated during the microcarrier manufacturing process. The fines can wind up in the culture system. Fines can also result from beads being crushed during the cell harvest process. As stem cell cultures cannot be readily filtered to remove these particulates, any small particles will be co-purified with the cells. The presence of foreign particulate matter such as microcarrier fines is unacceptable for injectable products. EMD Millipore is collaborating with the
Toronto-based Centre for Commercialization of Regenerative Medicine (CCRM) to develop optimized conditions for bioreactor-based cultivation of stem cells. CCRM is a federally incorporated, not-for-profit organization dedicated to supporting the development of foundational technologies that accelerate commercialization of stem cell-based and biomaterials-based technologies and therapies. The joint project will focus on exclusive development of a monitoring and control methodology enabling robust growth of microcarrier-adherent human pluripotent stem cells in EMD Millipore’s CellReady® stirred tank bioreactor. Ultimately, the project is expected to result in a commercially available kit containing reagents and associated methodologies for bioreactor culture of stem cells on microcarriers.
• Bioreactors optimized for stem cell culture.
Existing bioreactor technology is designed primarily to support the production of proteins expressed in the supernatant of cell cultures. Supernatant samples are easily extracted from the reactor for analysis.
In the case of stem cell cultures, however, bioreactors must allow rapid sampling of small volumes containing the actual cells.
Stem cell cultures need to be well mixed in the bioreactor prior to sampling as they tend to settle quickly. Because the cells are the actual product (in contrast to protein therapeutics produced by cells) the sample size must be small to avoid wasting valuable product and must be processed rapidly while the cells are still viable.
• Technology for harvesting and packaging of live cells. Existing centrifugation and filtration technologies are not optimized for the harvest and recovery of live cells. While centrifugation is often used to collect cells for research applications, it is not always practical for collection of large numbers of stem cells. Centrifugation is typically not a closed system, and shear forces can damage cells. Once cells are harvested, they must be rapidly concentrated, the media washed away with buffer solution, and packaged into containers for freezing or administration to patients (a process known as “fill/finish”).
No systems currently exist to efficiently manage the fill/finish process for stem cells.
Figure 3: Challenges across the stem cell scale-up process.
Investigative toxicity testing
In addition to their direct use in the area of regenerative medicine, stem cells offer unique advantages when incorporated into the small molecule drug discovery and development process. Pharmaceutical companies are now using stem cells to elucidate disease mechanisms and pathways, facilitate novel target discovery, assess and optimize lead compounds, and improve metabolic profiling and toxicity evaluation. One area that is receiving a great deal of attention is the use of stem cell-derived human hepatocytes in investigative toxicity studies.
The liver plays a central role in processing and metabolizing drugs and other substances in the bloodstream. Because hepatocytes are responsible for metabolizing most compounds that enter the body, these cells are used during the drug discovery process to predict how drugs will be metabolized and to what extent a drug may be toxic to the liver.
Drug-induced liver injury is one of the principal reasons clinical trials are suspended and approved drugs withdrawn from market. In fact, drug-induced liver injury has been the most frequent single cause of safety-related withdrawals of marketed drugs in the United States over the past 50 years.1
Investigative in vitro liver toxicity studies are typically conducted using primary human hepatocytes or an immortalized (genetically transformed) liver-derived cell line such as HepG2. Despite routine use for investigative toxicity, both of these options present significant drawbacks: • Primary human hepatocytes are derived from fresh liver tissue, typically sourced from cadavers or cancer resections. Supply of these cells can be limited and the tissue can vary widely among donors. • Primary hepatocytes cannot be sustained for more than a few days in culture without losing function. Securing a consistent supply of cells requires repetitive sourcing, which further contributes to variability. • Immortalized hepatocyte cell lines can be cultured indefinitely, which addresses the supply and variability issues associated with use of primary human hepatocytes.
However, these cells display distinct differences from normal liver cells and may not exhibit normal cell behavior or response.
For example, most cytochrome P450 enzymes (responsible for drug metabolism) are expressed only weakly in HepG2 cells compared to normal human hepatocytes.
The challenges of hepatocyte-based in vitro toxicity testing have led pharmaceutical companies to rely heavily on animal models for preclinical metabolism and toxicity testing. But animal models also have limitations. Animal models may not be fully and reliably predictive of human toxicity, are low throughput, expensive, and raise ethical concerns for some.
Cost and throughput often relegate use of animal models to the later stages of preclinical development, after a company has invested significant resources and time in a lead compound. This delayed evaluation of toxicity contributes to the high failure rate of compounds in late stage preclinical testing, which is extremely costly.
Earlier, more effective assessment of drug candidate toxicity has the potential to reduce the attrition rate of drugs in later stages of development.
Differentiation and expansion of human stem cells into hepatocytes for use in investigative toxicity studies could overcome the shortcomings of primary hepatocytes and immortalized cell lines. Use of stem cellderived hepatocytes (and other cell types commonly used for toxicity studies) offers a number of important advantages to investigative toxicity studies including: • Availability of a consistent source of cells that more closely match in vivo phenotype and physiology • Elimination of reliance on donor sources which can have sporadic availability • A more standardized, reproducible process for toxicity testing • Reduction in the use of animal models and animal tissue • Improvement in the predictive capabilities of early toxicity studies leading to reduction in late stage attrition of drugs
More efficient and predictive toxicity studies enabled by stem cell-derived cells can be expected to reduce development costs associated with the late stage failure of drug candidates. Identification of drug candidates with toxicity issues earlier in the discovery process will result in improved safety for clinical trial participants and patients.
reference
1. Guidance for Industry: Drug-induced
Liver Injury. US Department of Health and
Human Services. July 2009.
Robert Shaw is the Commercial Director for the Stem Cell Initiative, EMD Millipore
For more Cell Culture information visit our rESEarCH aNd dEvELOPmENT Web Portal at