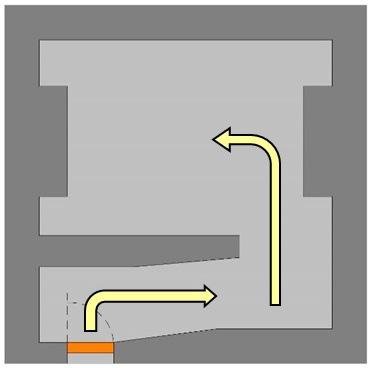
20 minute read
An Innovative Approach to Vault Design and the Evolution of the Radiation Oncology Environment
03
Outside the Box: An Innovative Approach to Vault Design and the Evolution of the Radiation Oncology Environment
Sapna Bhat, RA, LEED AP, sapna.bhat@perkinswill.com Justin Parscale, AIA, LEED AP, justin.parscale@perkinswill.com
Abstract
This article investigates the design strategies for enhancing the well-being, comfort, and safety of the patient along with providing spaces for the care givers that streamlines workflow, adapt to technology and care within the premises of radiation oncology treatment. The vault is the core of the radiation oncology treatment process. This study analyzed how the vaults and adjacent program could be reimagined with a goal and purpose to not only improve patient outcomes but also to provide efficiencies within the clinical operational and treatment model. The research method included a case study of the client’s existing cancer facilities and literature reviews followed by design explorations. In addition to the vault design, the owner challenged the design team to incorporate spaces for staff and physicians that aid in collaboration and advancement of the treatment methods through research and technology. The outcome of the final design implementation has been included in this article. Keywords: linear accelerator, cancer, mazeless, radiation, collaborative, and technology
1.0 Introduction
Radiation therapy or radiotherapy is one method of cancer treatment where a high dose of radiation is administered to and through a tumor to kill malignant cells and shrink the size of a cancerous tumor. This high dose of radiation is administered fractionally over the course of several weeks with the intent of destroying the DNA of the tumor cells thereby rendering it incapable of multiplying and growing. The radiation can be administered either externally or internally. This study focuses on the external radiation therapy application using photons, where a measured dose of radiation is guided by equipment known as a linear accelerator. The linear accelerators (linac) do not penetrate or touch the patient’s body; however they move around the body and direct the radiation towards the targeted tumor. The treatment is administered in a room often referred to as a vault or a bunker, where the patient is positioned on a treatment table in a predetermined position (as established days earlier by CT simulation). Each patient’s positioning is unique to their treatment. Multiple patient-positioning devices exist and each aid to restrict the movement of the patient during radiation treatment (beam on process). The total treatment usually lasts for 30 minutes, during this time the patient is alone in the vault while the care team is observing and recording and communicating with the patient from the control area just adjacent to the vault.
Due to the high amount of radiation present in the vault, the vault itself must be shielded so that the radiation remains contained within its designated space thereby eliminating the radiation exposure to personnel in the adjoining areas. This requires the vaults to be constructed of either cast-in-place high density concrete, or if budget permits, by using lead blocks of thicknesses determined by a physicist working in conjunction with the design team. In earlier designs, vaults were in the basement of the facility in part to lessen the burden of constructed shielding walls, utilizing the adjacent
earth’s soil as shielding. This below-grade construction approach compromised the overall efficiency and experience of both the staff and patients. Many of the most recent cancer center designs locate the vault above grade near the main entry level, providing easier wayfinding and more efficiency in terms of patientthroughput, However, challenges remain in terms of efficiency for staff and experience by the patient within the vault itself.
While designing a new radiation oncology facility at the University of Texas Southwestern Medical Center (UTSW) in Dallas, Texas, our primary task was to “rethink everything”, including the re-imagining of the vault design and addressing multiple points, including patient experience, staff experience, efficiency, supply chain management and overall safety of the users of the space.
2.0 Methodology
The research method for this project included a case study of the client’s existing cancer centers, literature reviews followed by in-depth design explorations. Understanding UTSW’s existing workflows, patterns, pit falls and successes within their existing cancer centers helped establish the priorities for the care team in their new facility. Additional literature reviews of vault design, shielding and other construction methodologies aided in the design explorations that were studied and proposed to the client.
3.0 Approach 3.1 Case Study
University of Texas Southwestern Medical Center (UTSW) engaged Perkins&Will to design and create a single patient-centric radiation therapy facility by consolidating their clinical and treatment spaces within one building and providing a singular venue to expand their operations. The goal was to create a state-ofthe-art facility which incorporates innovative design concepts and provides spaces that aid in research and collaboration. At the onset, the client’s focus was the design of the treatment vault itself. In-depth analysis and understanding of prevalent vault designs, the recognition of existing challenges and pitfalls provided the foundation for the reimagined vault, thus becoming a key marker for the overall success of this project. The design solution had to be innovative, functional, patient/ staff centric, efficient, and flexible to newer technologies and cost-conscious.
Additionally, since this facility is part of the university system, research and collaboration amongst various cancer care personnel was important. The design team was tasked with the challenge of creating spaces that break down silos and encourage engagement of physicians, nurses, physicists, residents, and the expanded clinical team.
Radiation Therapy Treatment Vaults: As mentioned before, radiation therapy vaults are enclosed treatment rooms where a patient diagnosed with specific type of cancer is treated with radiation.
In a traditional vault design, the radiation that is passed from linacs involve primary and secondary scatter of radiation particles (gamma rays). The primary shielding protection is provided by thick concrete or lead walls following the path of travel of the primary beam while the remainder of the vault is comprised of secondary shielding as protection from radiation scatter. Together the primary and secondary concrete or lead shielding form the vault itself. Often a third element is incorporated with the inclusion of an internal wall, forming the maze, as shown in Figure 1.
Figure 1: Entry into a treatment vault through a maze.
As part of the design process, Perkins&Will analyzed two UTSW’s Radiation Oncology facilities, which were to be consolidated into a single facility—Simmons Radiation Oncology and Moncrief Radiation Oncology. Based on the analysis of the existing facilities, the following were some of the findings for vault design and operations. The existing Simmons facility incorporated three mazed vaults, each housing high-energy linear accelerators. Figure 2 indicates the floor plan and the location of the vaults contained within it. Figures 3 and 4 are photographs of the existing conditions, showing that the treatment vaults are cluttered and lack much structured organization and storage. Though not unique to UTSW, there is a natural sense of foreboding for the patient as they traverse the circuitous maze into the treatment zone.
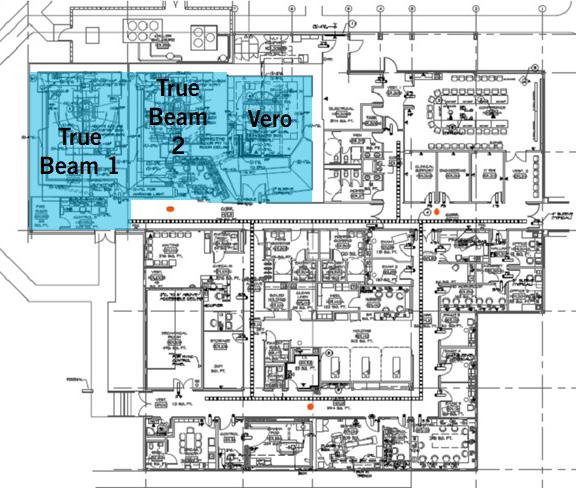
Figure 2: Floor plan of Simmons Radiation Oncology.
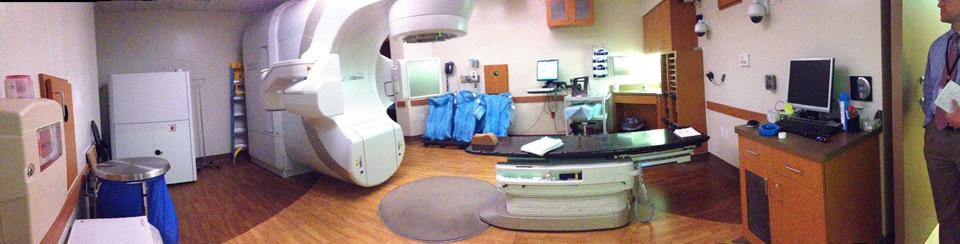
Figure 3: Existing conditions of radiation therapy treatment vault (True Beam equipment) within Simmons Radiation Oncology.
Figure 4: Existing conditions of radiation therapy vault (Vero equipment) within Simmons Radiation Oncology.
Figure 5: Circuitous entry into the linac at Simmons Radiation Oncology. Figure 6: Control area adjacent to the vaults at Simmons Radiation Oncology.
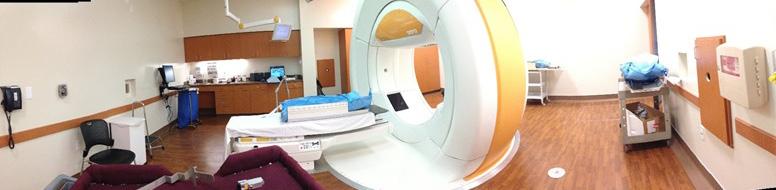
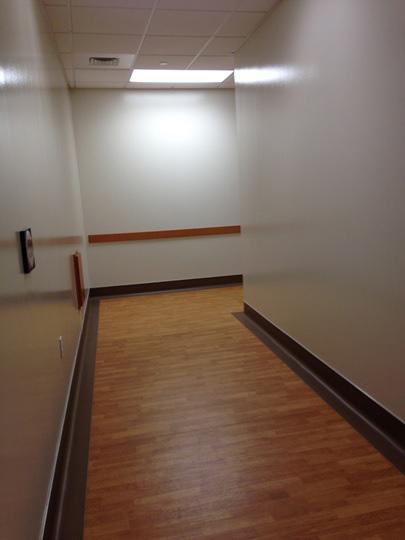
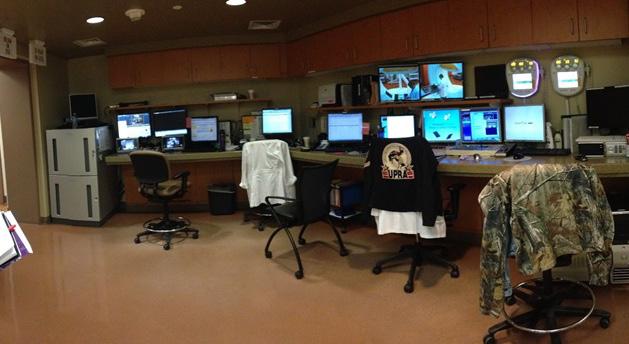
At UTSW’s Moncrief Radiation Oncology building, the care and treatment program were distributed between three floors: the basement housing the linac vaults, clinic, first floor housing the main lobby, patient registration and financial counselors, and the second-floor housing CT simulation and treatment planning. At each vault, a maze led the patient into the treatment zone and the treatment area was cluttered with supplies and storage space was direly needed. The underlying comment from the staff at both these facilities was not just the lack of physical availability of storage space but the lack of ease of finding supplies and immobilization devices designated to each patient.
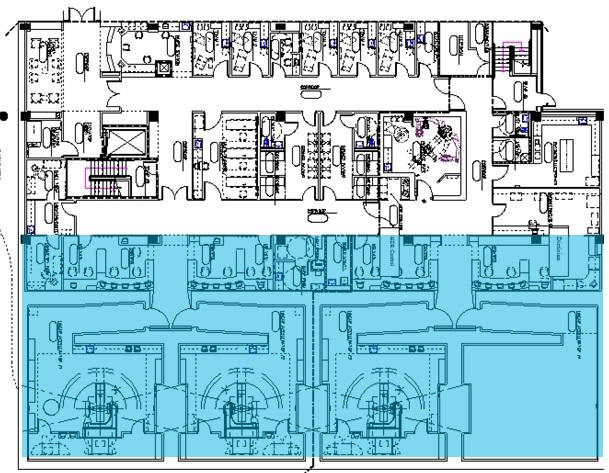
Figure 7: Floor plan of Moncrief Radiation Oncology.
Figure 8: Circuitous mazed entry into the treatment vault at Moncrief Radiation Oncology. Figure 9: View of the vault at Moncrief Radiation Oncology.
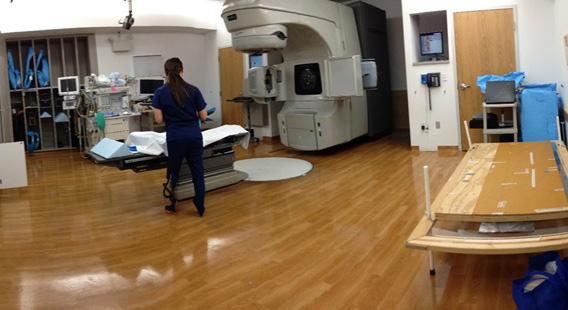
An additional vault design challenge was the incorporation of a mobile CT that was to be used during the treatment process. The mobile CT, which is approximately 36”w x 48”d x 60”h required space to park and maneuvered into and within the vault easily without compromising the patient experience or inhibiting staff workflow. The goal of UTSW’s Radiation Oncology care management team was to be more efficient and effective through thoughtful workflow planning. The overall design was to create a road map to direct their care team in support of accomplishing their goals. Their current layout created silos amongst the care team, creating a restricted environment to advance research and growth.
3.2 Literature Review
Findings from the literature review were grouped into three categories:
nj Radiation protection & safety
nj Effects of physical space on individuals, and
nj Cost metrics. 3.2.1 Radiation Protection and Safety
Radiation protection and safety are critical factors in designing the vaults. The treatment room shielding should be designed in accordance with suitable recommendations by the physicist performing the shielding calculations in compliance with the shielding regulations imposed by jurisdictions. The room should be large enough to accommodate the specific room’s treatment machine, allowing the full range of motion of the treatment table and patient transport. Access to the radiation treatment room should be clear and should be furnished with a visible signal indicating whether the radiation source is on or off. The door interlocking mechanism to prevent unintended opening during treatment is important, as well as providing a manual release button in case of emergencies. Visual access of the patient is necessary through the cameras within the treatment room which communicate to the control console and care team.1
Primary and Secondary Beam Shielding: The treatment beam is known as the primary beam and portions of the wall, floor and ceiling providing necessary shielding protection from the primary beam are called primary shielding. The orientation of the linac within the room requires increased concrete thickness to attenuate x-ray
beams that fall on the shielding surfaces after passing through the patient. Radiation produced when energy is bounced off the patient and other wall/floor ceiling surfaces are called secondary scatter, and secondary shielding provide the necessary protection. Typically, a maze is designed as part of the treatment bunkers to prevent primary and secondary scatter. In instances where photon energies exceed 8.5MV, additional protection is required to attenuate scattered neutrons and is usually achieved by longer maze and shielded door at the end.2
Shielded Doors: Door shielding is complicated because access to the facility necessitates a break in the required continuous shielding. The door must be comprised of a shielding material equivalent to the shielding that would be removed by the door opening. The door may not be placed in the primary barrier. The shielded door can only be located within a secondary shielded wall. If neutrons are present, the door should be comprised of borated polyethylene combined with lead and steel laminate to prevent the scatter from escaping as determined by the physicist. These doors are extremely heavy requiring the use of a motor to operate.3
Shielding Materials: Different materials offer protection of varying degrees for shielding applications. Materials are primarily incorporated based upon their effectiveness of shielding properties from different radioactive elements. Additionally, cost and convenience are the driving factors of material incorporation. Construction materials such as concrete, steel and earth are the most used materials for shielding applications. Neutron shielding requires materials that contain hydrogen, while x-ray shielding materials need high mass and atomic number. Materials that cater to one or most of these requirements are concrete, lead, steel, polyethylene, earth, and wood. Concrete is the most incorporated shielding material as it provides protection against x-ray, neutrons while being comparatively less expensive and can be poured in different configurations. There are options to use normal weight concrete or high-density concrete. Higher density concrete uses higher density aggregates and provides better photon attenuation but is not necessarily ideal for neutron shielding. High density concrete provides a design advantage of requiring thinner walls as compared to normal weight concrete. Lead is a dense material and offers excellent shielding against photons. However, lead is not able to support its own weight requiring additional structure and cannot shield against neutrons.
Steel is not as dense as lead, but it is structurally sound and non-toxic. As mentioned previously, borated polyethylene is perhaps the best neutron shielding material available and is often used to fill the voids around HVAC, wiring, and in doors.3
3.2.2. Effects of Physical Space on Individuals
Research has shown that the general perception of the physical surroundings through the aid of senses impacts the physical wellbeing of an individual.⁴ An individual’s appraisal or perceptions of the event rather than the event itself are predictive of the deleterious effects of stress on health and wellness (Joseph, 2007; Smith, 2007).⁴ There have been studies that indicated high blood pressure levels, increase in heart rate, nausea, and other varied responses to stress.⁴ The responses differ among individuals and are further enhanced if the individual is a patient that is already stressed stress due to the diagnosis of their current health condition. The staff and care takers are also function at higher stress levels directly affecting not only their personal health but can also interfere with the quality of care they provide. With the patient being the most important user of a healthcare facility, it is more important to create spaces and provide opportunities that add to the overall patient experience. Many indignities experienced by patients may be reduced through careful space planning and design. Medical clutter, waste containers, water coolers, coffee makers, personal displays and decorations culminate in a distressing level of visual chaos. Facilities that reduce stress for patients have the same impact on staff, alleviating tension as they care for patients. Putting the patient's experience first may contribute towards initial higher investment, but in return studies have shown that the providers enjoy a strong reputation and exceptional customer loyalty.5
Careful orchestration of various functions within the space, selection of materials, lighting and other design interventions that helped in reducing stress perceived through the sensorial organs were studied and implemented.
3.2.3 Cost Metrics
According to the statistical report by US Oncology, the market share for radiation therapy is expected to grow to 3.9 billion dollars by 2027.6 The key factors that have shaped this trend is the prevalence of cancer along with technological advancements that have made radiation therapy a viable option for treatment. The need to have efficient facilities in treating patients with faster turnarounds becomes more critical in achieving targeted patient volumes. Radiation therapy requires high capital expenditure and is staff and resource-intensive.
Initial investment costs for radiation oncology are driven by equipment costs, along with the construction materials and methods implemented for shielding. Coupled with that, the operational costs are driven by multiple factors, including the choice of working hours of the facility, supply and demand of consumables, ease of maintenance of the facility as well as equipment, staff retention and satisfaction, and quality of care.
3.3 Evaluating Scenarios
Based on the literature reviews, the design team engaged in discussions with the client’s choice of linear accelerator manufacturers to understand the spatial needs, equipment limits and restrictions. To consider different scenarios related to the vault design, the design team hired a cost estimating consultant to understand the initial cost scenarios of using cast in place concrete versus the use of shielded blocks for shielded wall construction. Based on the cost metrics, it did not seem feasible to employ shielded blocks for the case study, but to design around cast in place concrete. The design team explored methods to reduce the volume of concrete used in these vaults and focused on solutions to achieve economies by sharing the shielding between vaults.
4.0 Re-Imagining Vault
There were three principal areas that design of the vault focused:
nj Reduced square footage (especially important as this would bring cost savings due to reduced volume of concrete) nj Patient and staff experience
nj Ease of access to supplies and movement of mobile CT (staff efficiency). The design solution first targeted the area used by the maze. The maze not only caused stress and anxiety to the patient, but it also increased staff’s foot traffic and encouraged unsightly storage of equipment. Elimination of the maze required additional radiation shielding protection at the entry/doorway specifically from photons and neutrons, resulting in a more substantially shielded door. Based on the literature review and discussions with the physicist and the door manufacturer, this door needed to incorporate both borated polyethylene and lead to provide shielding protection. Efficiency of workflow being a key factor in the design process, the incorporation of a bi-parting door in lieu of a slower moving traditional swing-door was integrated to support a quicker patient turn-around. This elimination of the maze resulted in a reduction of cubic yards of concrete by reducing the vault costs. This allowed for the incorporation of a more costly, yet efficient shielded door resulting in costneutral savings. The treatment area within each vault was carefully studied such that the spatial requirements were adequate and accepted by all incorporated linac equipment vendors.
As discovered during the literature review, primary shielding is directly related to the orientation of the equipment within the vault and is a continuous belt that goes across walls, ceiling, and flooring. As indicated in Figure 10, the vault with the maze has primary shielding projecting into the vault on opposite sides. In the proposed vault, concrete was used as the main shielding material along with strategic placement of lead blocks. The use of lead blocks embedded within the concrete helped in reducing the wall thickness required at the primary shielding. Since the lead blocks are more expensive, this combination was only applied to the wall where it made a bigger impact with the overall aesthetics of the vault. In this design, the combination of lead and concrete was applied to the wall closest to the patient entry. This enabled the removal of the unsightly projection into the vault as the patient walked in and provided no opportunities for unwanted storage nooks. This wall became the accent wall with quiet elegance and was devoid of clutter and chaos.
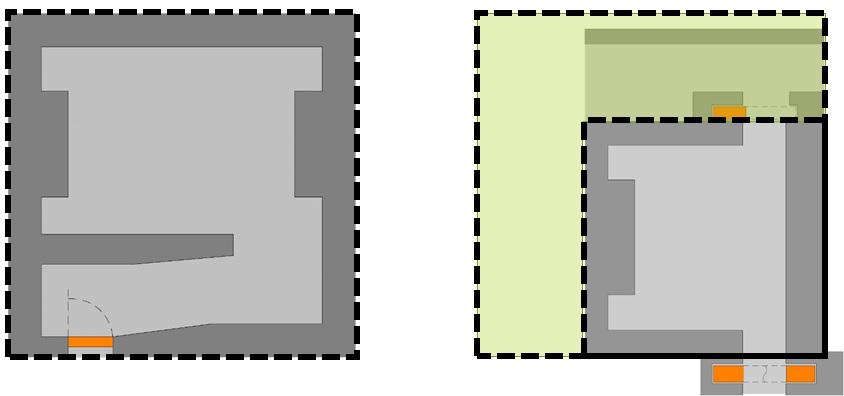
Figure 10: Square footage comparison between a vault with maze and the vault at UTSW..
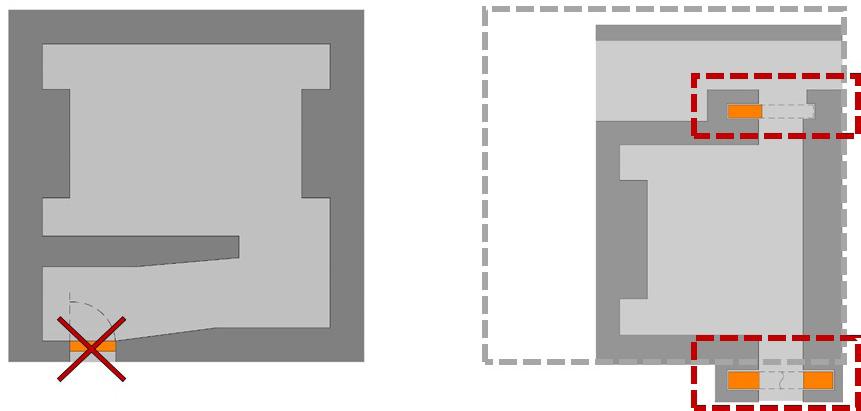
Figure 11: Addition of 2 shielded doors at UTSW vault – separating patient and supplies entry points.
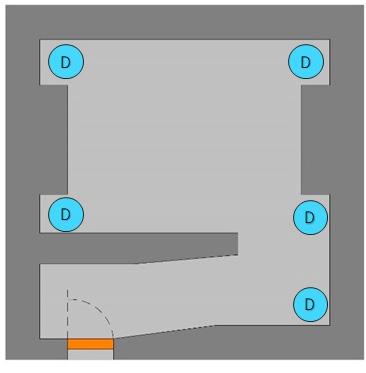
Figure 12: Possible places to store and create clutter within a traditional vault design. Figure 13: Examples of storage within the vault at Moncrief Radiation Oncology.
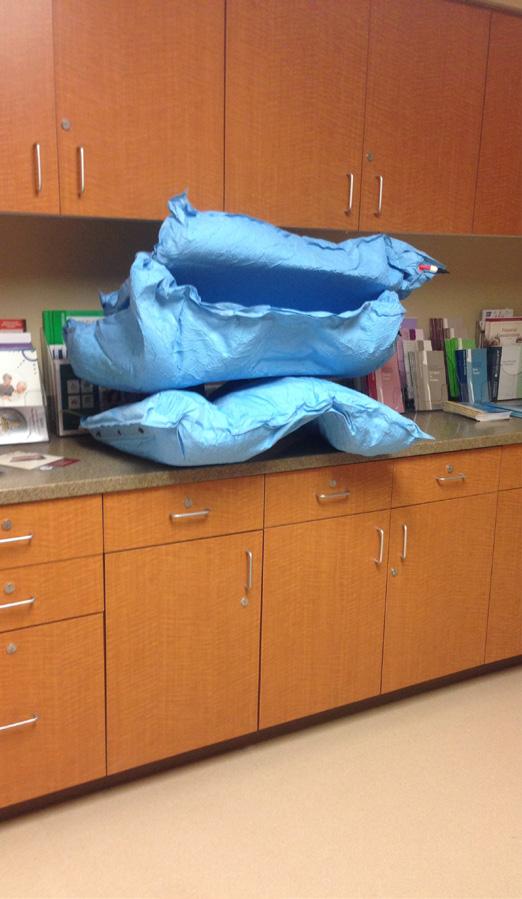
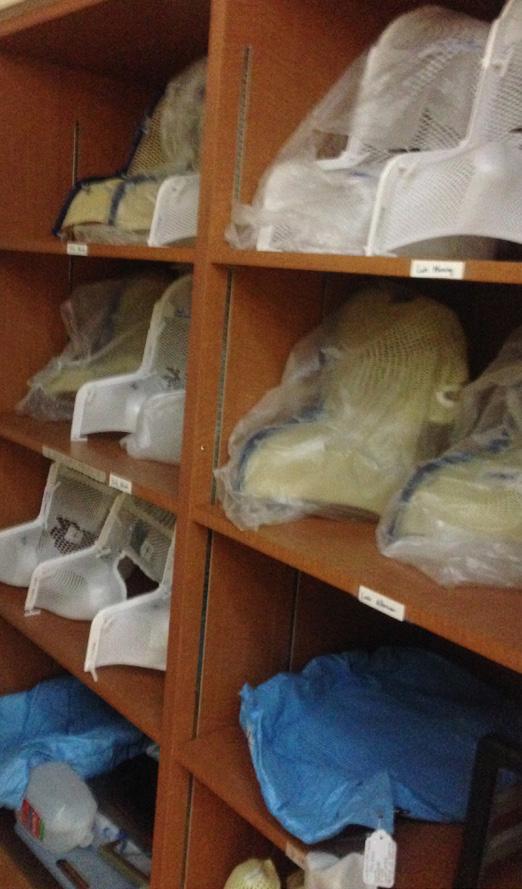
Perhaps the most innovative modification to the design of the vault itself is the incorporation of a second shielded door. At UTSW we intentionally minimized the opportunity for in-vault storage, often perceived as clutter by the patient and an inhibitor of efficiency to the care provider. This second door allows for the movement of not only treatment supplies but also the passage of a planned mobile CT. Much like the sterile core supports a surgical suite in the main hospital, the inclusion of what we have deemed a “technical corridor” has been incorporated along the back of a series of vault treatment rooms—accessed by the additional door. The technical corridor provided ample space for storage to organize regular supplies and vac lock bags, molds, cones, and lenses specific to each patient. The technical corridor also housed blanket warmers, soiled bins closet, and provided a parking space for the mobile CT at either end of the corridor. Space was also allocated for vendorspecific equipment storage. This innovative design has helped reduce clutter within the vault, provided greater and more organized storage space for staff at a lesser cost per area, improved staff efficiency and improved the overall experience of the patient.
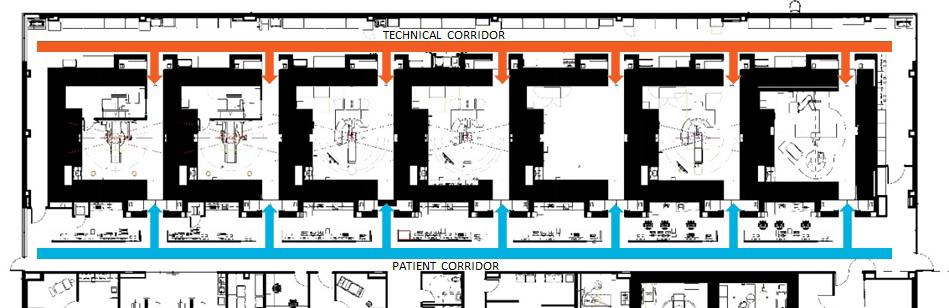
Figure 14: Patient (blue) and supply (orange) flow into each vault..
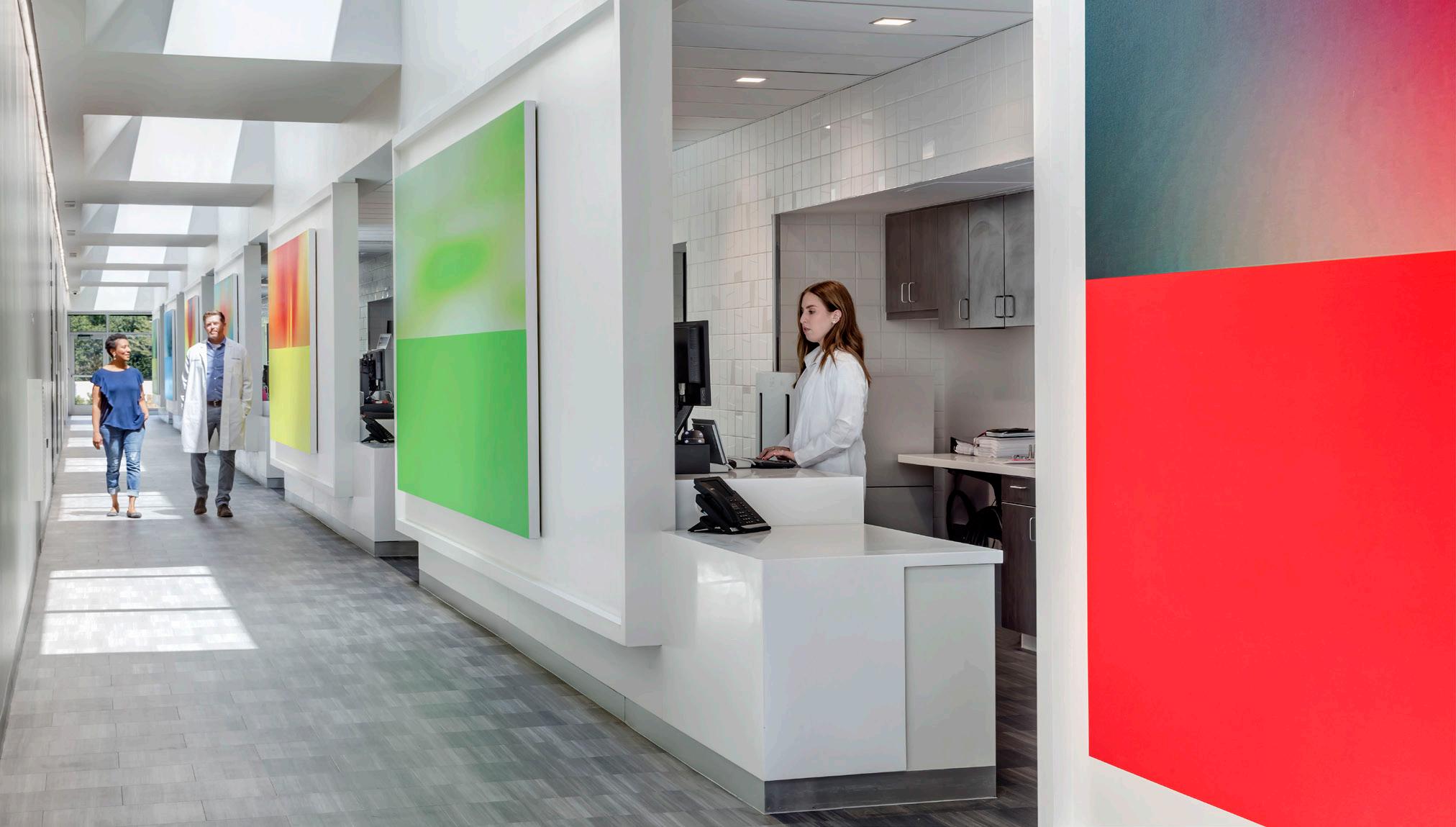
Figure 15: Daylit patient corridor at UTSW leading to the treatment vaults.
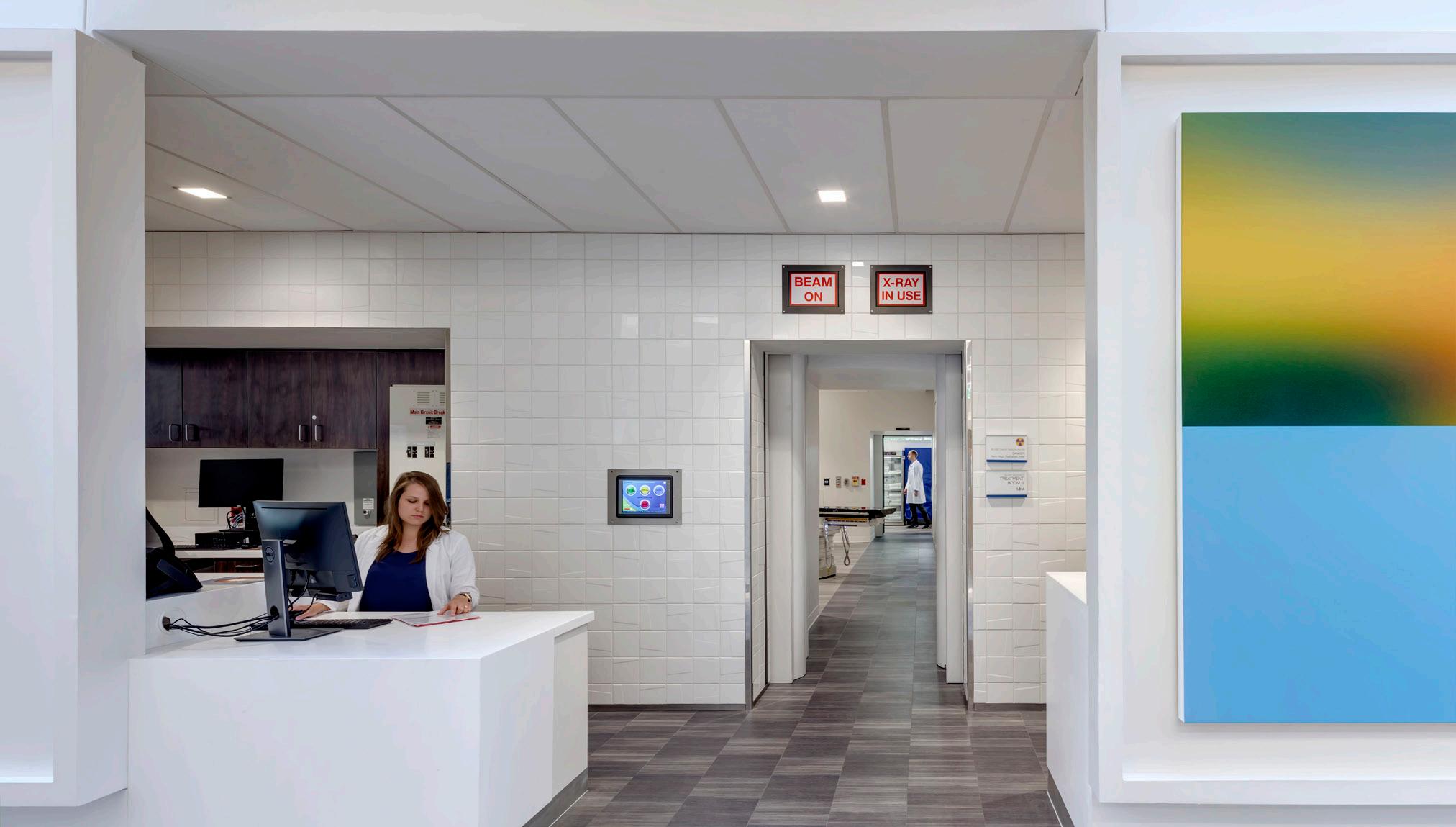
Figure 16: View from the patient corridor into the control console and vault with the technical corridor in the background.
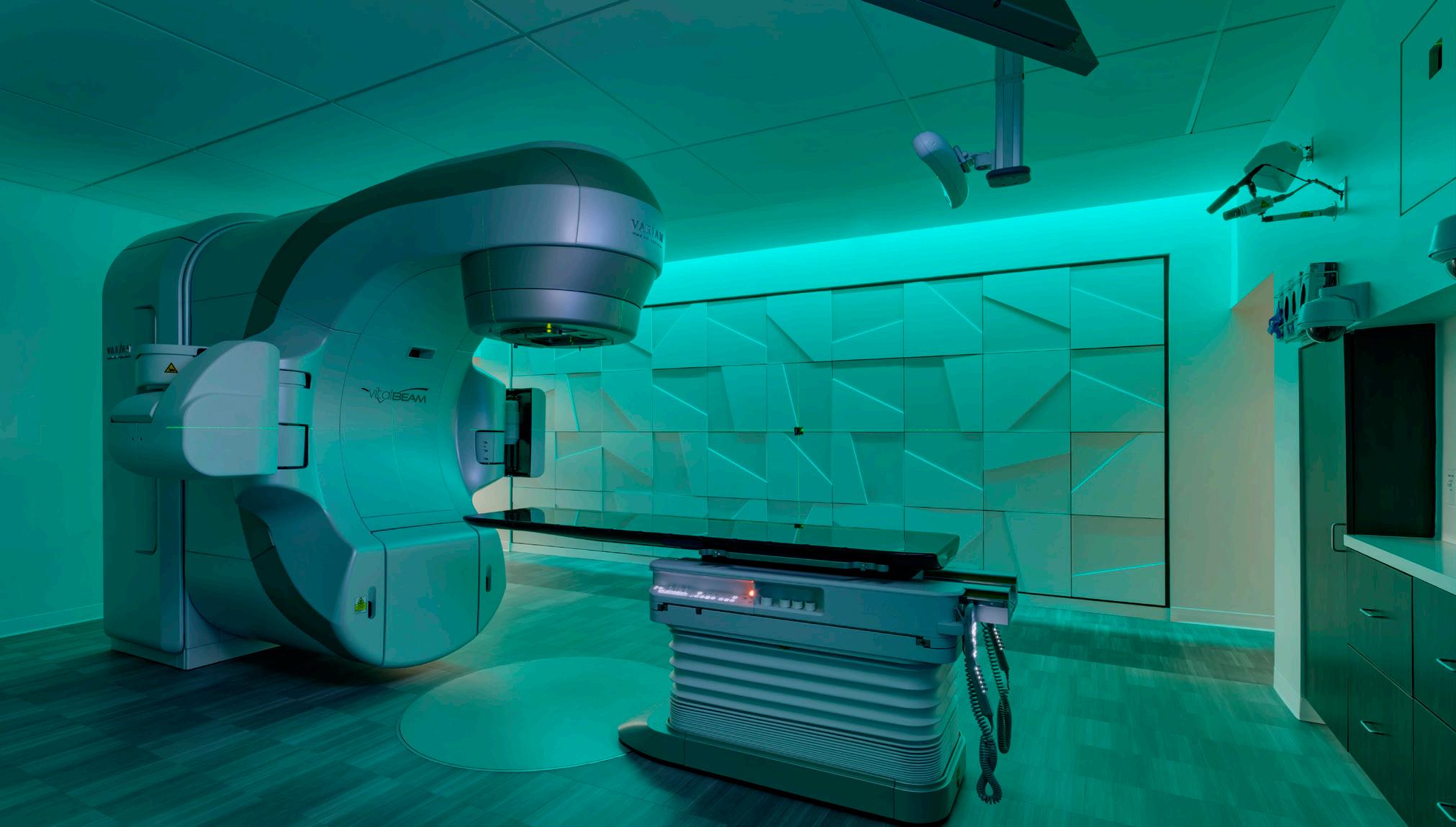
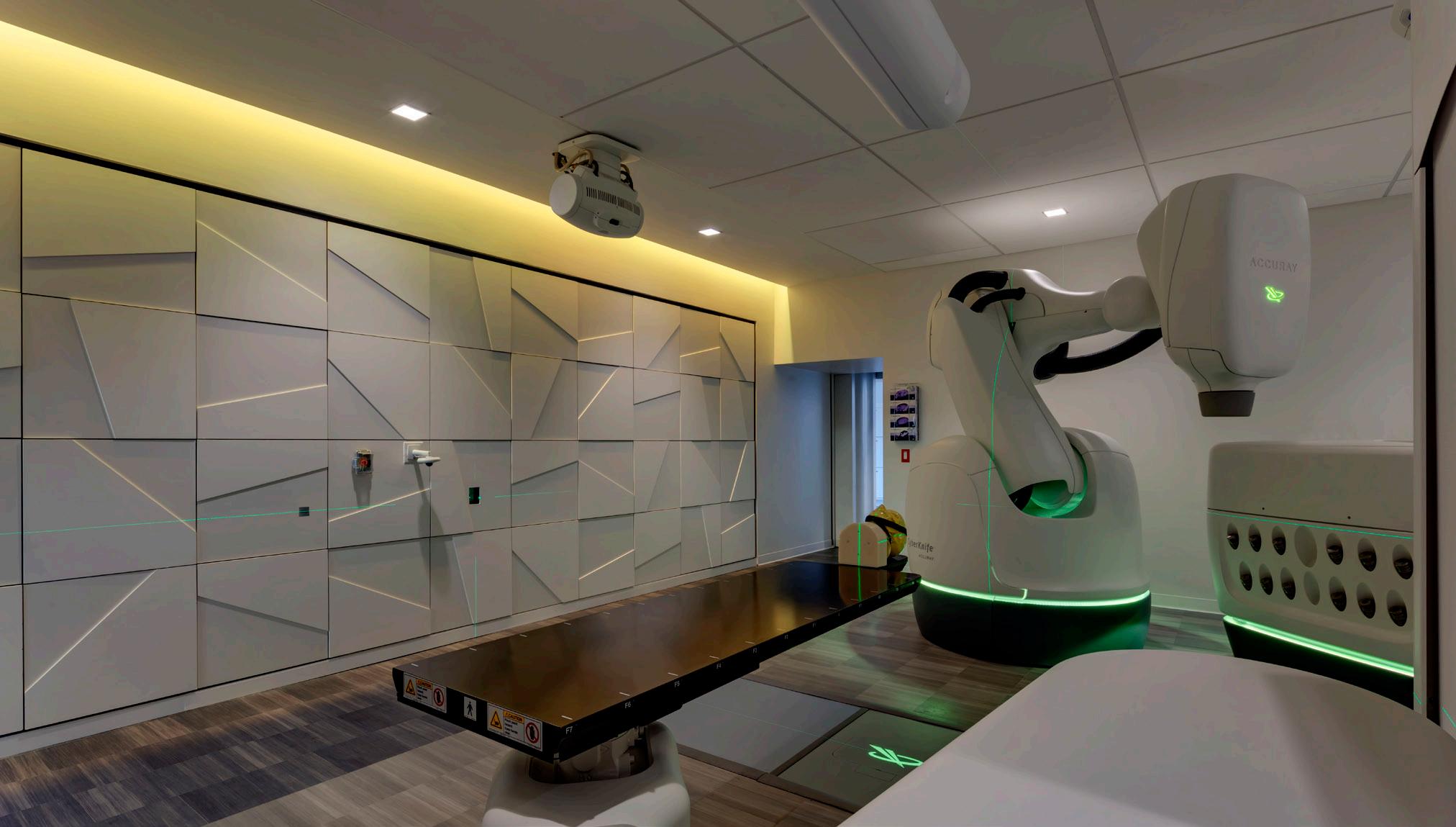
Figure 17: (Above and Below) Views inside the vaults at UTSW, with the elimination of in-vault storage and clutter.
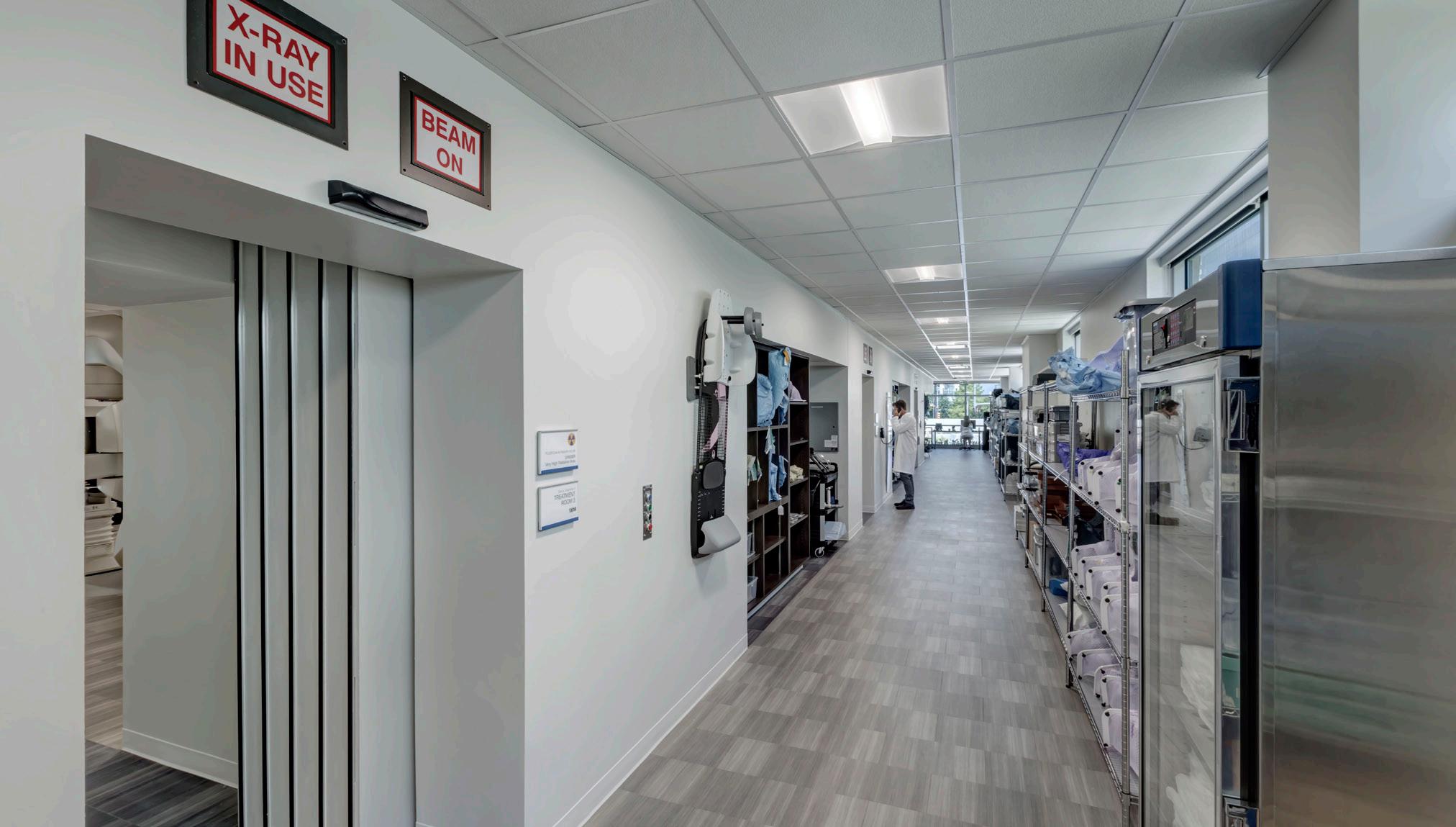
Figure 18: View of the technical corridor showing organized storage/supplies and access to daylight.
By forming Disease Oriented Teams (DOTs), UTSW put the staff and physicians closer to the center of their field of expertise. Each DOT worked in an open office collaborative area, where they were able to exchange information easily amongst their specific teams and amongst other disease teams. This located the physicists, dosimetrists, medical assistants, and residents all working in close relation with one-another and the physicians. Each team had a central open office color-coded zone surrounded by the respective physicians and physicists. Between each of the three zones were informal huddle spaces that encouraged serendipitous collisions amongst peers. These informal collaboration zones pave the way for changing UTSW’s Radiation Oncology team’s way of communicating, educating and sharing information and in delivering care.
5.0 Conclusion
The vision set forth by UTSW and their challenge to the design team to "reimagine everything” resulted in the reimagined vault and associated Technical Corridor, resulting in a collaborative work area that engages staff holistically in providing care. This facility was the first to incorporate the two-door vault and Technology Corridor. This NCI-designated comprehensive cancer center at UTSW was completed in 2018. Since the completion of UTSW, Perkins&Will has designed additional cancer centers each incorporating and even improving upon the two-door, maze-less vault and Technology Corridor design albeit on a smaller scale. Some of the improvements included within the vault design are:
nj Increased floor area by approximately 50 SF to allow for better patient/equipment maneuverability within the vault, specifically at the foot of the couch. nj Incorporation of lead block within the primary shielding wall providing less physical interruption of the interior wall surface.
nj Newer technologies have more ceiling mounted equipment which are sensitive to light resulting in careful selection and placement of light fixture within the vaults.
Further study is needed to understand the efficiencies of the vault design coupled with the technical corridor on small scale projects.
Acknowledgements
The authors would like to acknowledge the entire project team at UT Southwestern for their vision and commitment during the design process. Also, a very special thanks goes to Claire G. Almanza, Director of Marketing, UT Southwestern, Radiation Oncology for her support of this article.
References
[1] Podgorsak, E.B., (2005). Radiation Oncology Physics: A Handbook for Teachers and Students, Austria, International Atomic Energy Agency (IAEA) Publication. [2] McMorrow, A., (2019). “Radiation Shielding and Bunker Design”, Biomedical: Scientific and Technical Research, Vol. 18, No. 1, pp. 13322-13324. [3] Darby, K., Mcclanaha, T., Moore, A., Crutcher, C., and Megonigal, M., (2015). "Accelerator Facility Shielding Design", Chancellor’s Honors Program Projects, Report, Retrieved on 04/2021 from https://trace.tennessee.edu/ utk_chanhonoproj/1830https://trace.tennessee.edu/cgi/ viewcontent.cgi?referer=https://www.google.com/&http sredir=1&article=2842&context=utk_chanhonoproj. [4] McCullough, C., (2010). Evidence Based Design for Healthcare Facilities, Indianapolis, IN: Sigma Theta Tau International.
[5] Jarvis, J., (2003). “Transforming the Patient Experience in Radiation Therapy”, Radiology Management, Vol. 25, No. 6, pp. 34-36. [6] Business Wire, (2020). “ United States Radiation Oncology Market Size, Share & Trends Analysis Report, 2020-2027”, Report, Retrieved on 04/2021 from https:// www.businesswire.com/news/home/20200915005600/ en/United-States-Radiation-Oncology-MarketSize-Share-Trends-Analysis-Report-2020-2027--ResearchAndMarkets.com.