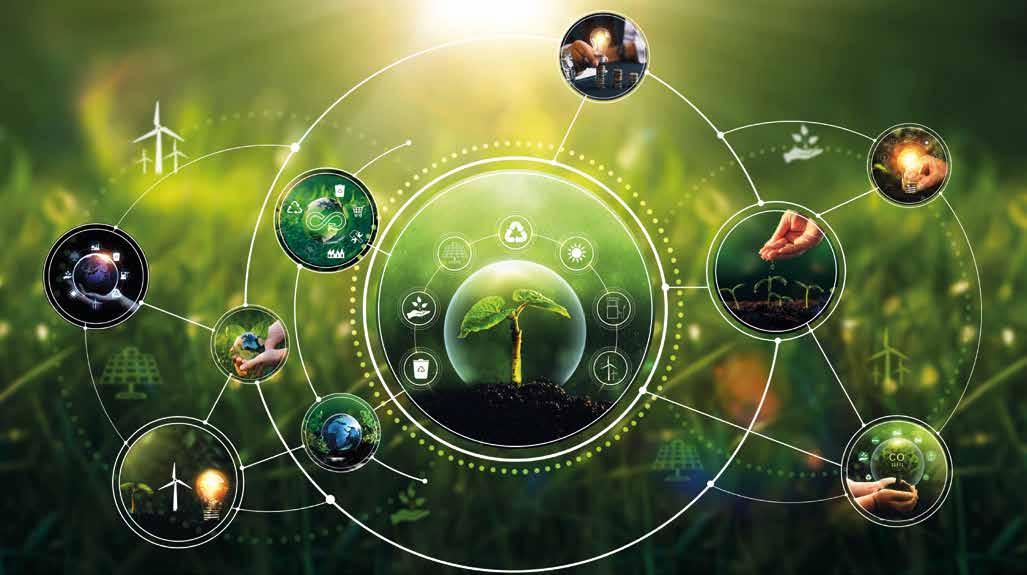
7 minute read
A SYSTEM CAPABLE OF REVERSING THE COURSE
Examples Of Circular Economy In The Energy Sector
“
The world has a finite volume of natural resources and a limited ability to produce new resources”. (From the report “The use of natural resources in the economy: A Global Manual on Economy Wide Material Flow Accounting”, prepared by the United Nations Environment Programme). Our planet is not in possession of anything that is “unlimited”, and this also goes for its ability to produce new resources. The considerable increase in the demand for raw materials and energy and the increase in consumption suggest a dramatic situation in the near future, so much so that, according to NASA, at least 3 habitable planets are needed annually to meet the current demand for resources (source: Dennis Bushnell, Chief Scientist at the NASA Research Centre in Langley 2018). The issue of sustainability can no longer be considered as a “secondary aspect” of the industrial processes and development of our society. If at one time the protection of the environment could not be included among the objectives of economic manoeuvres and technological development, today it must be the starting point and must represent a constraint that, when not respected, vetoes any operation carried out within the economic and social system.
A New Model
It is evident that a system capable of reversing the course is needed. The new economic model to be adopted should aim to redefine the concept of “growth”, focusing on the benefits it can bring to society, the environment and the economy at the same time.
According to the “National Report on the Circular Economy” produced by the Circular Economy Network (CEN), the shortage and increasing price of raw materials (phenomena exacerbated before the pandemic, now even more so by the international geopolitical situation following the war in Ukraine) can only be countered thanks to a large-scale implementation of an economic model, called “Circular” (Figure 1).
“Circular Economy” means that modus operandi through which everything that is extracted or obtained from the environment and used in order to create a product, a service or energy useful for their own realisation, can continue to be part of the economic cycle even after the satisfaction of the need for which it was conceived.
According to estimates relating to the need for industrial raw materials until 2030, at the end of the next decade the gap between demand and supply of commodities will be around 8 billion tons, and then gradually increase from year to year. Therefore, the need to adopt efficient waste management systems and reuse of waste is evident. Analysing the proposal from a strictly pragmatic and not only moral point of view, in addition to the improvement of climatic conditions, the introduction of a circular model would be a significant economic advantage. In addition to the decrease in waste materials, there would be the possibility of economic growth (an estimated increase in GDP of 0.5%) and an increase in jobs (in the EU, thanks to the circular economy, 700,000 new jobs could be created by 2030). Although it does not pass through easily viable paths in economic terms, the energy transition we need can take place through several paths, each one devoted, despite their differences, to the same purpose. The optimal energy transition must be effective, immediate and have an incisive approach. This will be possible globally only by adopting various solutions, which provide for different speeds, depending on the specific contexts.
Biorefineries
Among the many renewable energy sources, biomass is the most sophisticated form of solar energy storage. Through the process of chlorophyll photosynthesis, plants are able to convert solar energy into chemical energy, then store it inside high-energy molecules. Biomass is a versatile, renewable and, above all, programmable energy source. In the last decade, biomass has also proved to be, after solar photovoltaic and wind, the renewable source with the greatest development, and the production of energy related to it has registered a constant growth.
The society in which we live is strictly dependent on oil, which is used for the production of goods or derivatives useful for the production of the same, but also and above all for fuels. Oil consumption over time
(mainly gasoline and medium distillates) is constantly increasing. The global population is projected to reach 9.7 billion in 2050, and it is expected that there will be a sharp increase in fuel demand in the near future, exacerbated by a shift towards higher standards of living even in developing countries.
According to the ENEA (National Agency for New Technologies, Energy and Sustainable Economic Development), currently the concentration of CO2 in the atmosphere is equal to about 390 ppm, with a considerable growth rate. Among the multiple causes that can be investigated, the use of fossil fuels for energy production is the main responsible for massive CO2 emissions, also representing 77% of total GHG emissions (Green House Gases). Biomass could become an economically and, above all, ecologically credible alternative.
According to the International Energy Agency (IEA), biorefining is “the sustainable transformation of biomass into a spectrum of marketable products and energy”. The biorefinery operating process guarantees a drastic reduction – greater than 70% compared to the traditional production cycle – of the emissions of polluting substances.
In biorefinery, biomass is transformed into energy, biofuels and products of basic chemistry, fine chemistry, biopolymers and bioplastics, through technologies and processes that produce minimum waste and have a low impact on the environment. The approach has similarities with traditional oil refining in fuels and oils, the only substantial difference being the starting material: biomass.
Biomass, just like petroleum, has an articulated composition, which requires a suitable split into several groups of substances, whose subsequent treatment will lead to a complete variety of products. The initial organic material (such as agricultural and forestry residues, food and other solid waste) can be broken down into multiple compounds through a cascade of reactive phases.
Currently, there are about 298 biorefinery plants throughout Europe that are dedicated to the production of biofuels, bio-oils or green chemistry intermediates.
Green Hydrogen
To date, there are many reasons why hydrogen is considered one of the key energy carriers for the future energy transition:
• it is the most abundant element in the entire Universe and constitutes 75% of normal matter by mass;
• a single kilogram of H2 is capable of developing 142 MJ of energy; a considerable value compared to 56 MJ for natural gas, 45-46 MJ for petrol, diesel or kerosene, 30-32 MJ for coal and 16 MJ for wood;
• its combustion does not produce CO2 emissions or other pollutants.
Paradoxically, the fact that an element is widely present on Earth does not imply that it is readily available in the required form. Different extraction technologies give names to different types of hydrogen. The term “Green Hydrogen” refers to the production of molecular hydrogen through a process powered by energy from renewable sources. In this case, water (H2O) is split into biatomic hydrogen (H2) and oxygen through electrolysis. Scientists believe that this technology can meet 24% of the world’s energy needs and contribute significantly to the zeroing of net emissions by 2050. However, the main obstacle to achieving these goals is cost, despite the fact that the actual costs of the electrolysis process have decreased by 50% in the last five years. The main feature of this process is that it has zero environmental impact.
Blue Hydrogen
Although green hydrogen represents a more than viable long-term solution, analysts suggest that in the short term (until about 2045), it may not be as economically beneficial as blue hydrogen.
FARMING/COLLECTION1
BIOCHEMICAL
REUSE/REDISTRIBUTE
MAINTAIN/PROLONG
1 Hunting and fishing
2 Can take both post-harvest and post-consumer waste as an input
SOURCE Ellen MacArthur Foundation Circular economy systems diagram (February 2019) www.ellenmacarthurfoundation.org
Drawing based on Braungart & McDonough, Cradle to Cradle (C2C)
Blue Hydrogen refers to the production of hydrogen by fossil fuels, such as natural gas. In this case, the production plant is coupled with a system for capturing and permanently storing the CO2 produced in the process. In this way hydrogen can be generated, without emissions that are harmful to the climate. Blue hydrogen could be the key element for the transition to low-carbon energy production (Figure 2).
Ccs
It is undoubtedly the technology with the greatest potential for a massive reduction in CO2 emissions in the short term (10-20 years).
The acronym CCS (Carbon Capture and Storage) indicates the entirety of all the techniques that allow:
• for the geological containment of carbon dioxide (CO2) from large combustion plants;
• the fluidisation of CO2, to allow it to be transported to a storage facility;
• the storage of CO2, i.e. its conservation, ensuring that no dispersion into the atmosphere occurs.
The International Energy Agency says that it is necessary, by 2025, to reduce current carbon dioxide emissions by at least ten times, in order to achieve the goals set for the global protection of the environment and health. The Global CCS Institute estimates that, by 2040, 2,500 CCS facilities are expected to be operational worldwide, each with a capacity to process about 1.5 million tons of CO2 per year. At the moment, CCS is the only technology available that is capable of massively reducing emissions
From Large Industrial Plants
The invitation is to take advantage of all possible elements to become fully aware of the defects of the current system and the possibilities of renewing and reorganising it in the best possible way. The aim is to promote a more livable and, at the same time, economically and socially sustainable system. Technological development and environmental protection cannot and must not be regarded as diametrically opposed concepts, but rather their substantial complementarity must be recognised.
This article was originally published in n.9/22 of “Il Giornale dell’Ingegnere”.
Translated by Translated https://energytracker.asia/hydrogen-oil-green-vs-blue-whats-the-difference/)
Capture technologies separate CO2 from other gases at the emission source
This can be a coal or gas fired power station or an industrial plant such as a steel mill or cement factory. Sufficiently high carbon prices or taxes create a business case to capture and store CO2
Transportation Storage
Once captured and separated, the CO2 is prepared for transport.
In most cases, CO2 would be pumped through a pipeline to an onshore or offshore storage location lt may also be transported by a vessel.
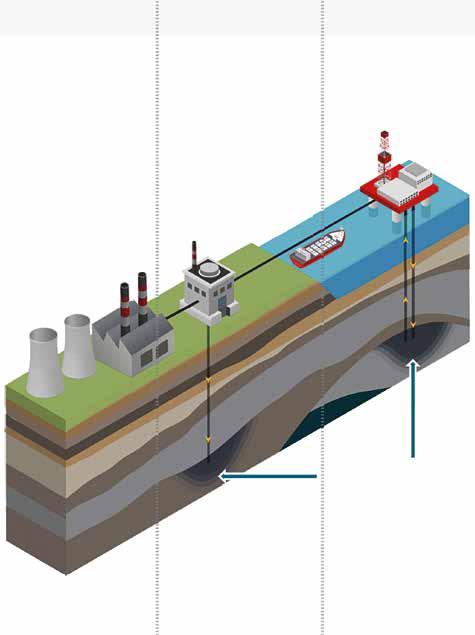
lf CO2 is stored offshore it is transported to an injection platform or (former) oil/gas rig.
oil or gas rig
CO2 can also be used instead of stored. Researchers are exploring future use options that could generate revenue.
Permanent storage may occur in deep geological formations (saline aquifers) or depleted oil or gas fields. CO2 can also be injected into oil or gas fields to enable ‘enhanced oil recovery’: injecting CO2 squeezes out remaining reserves. In this case, CO2 is a commodity that can generate revenue https://www.eca.europa.eu/Lists/ ECADocuments/SR18_24/SR_CCS_EN.pdf)