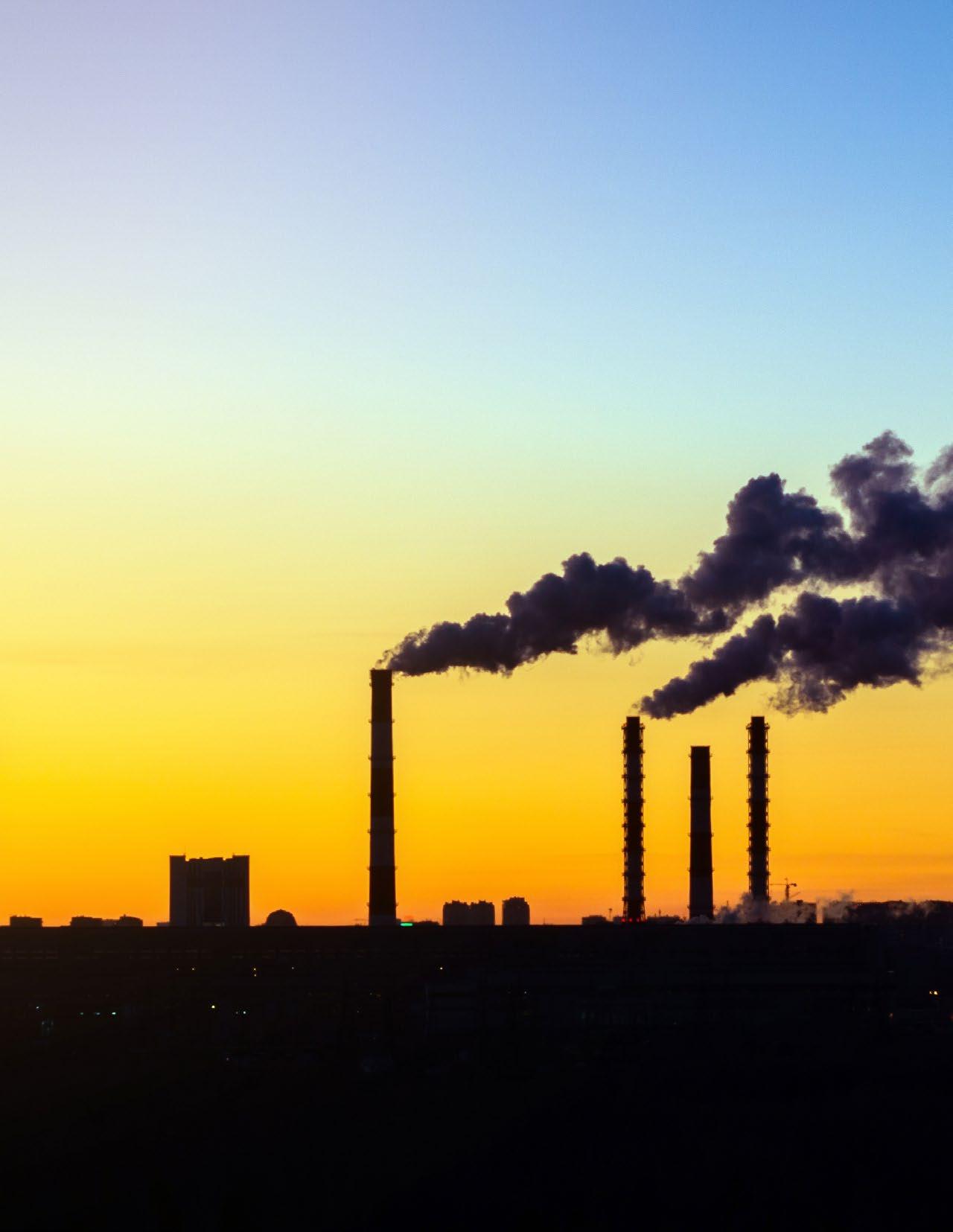
7 minute read
Lead Story: Carbon Capture & Storage Potential In Colorado
BY SUSAN HOVORKA AND EMILY MOSKAL
Carbon dioxide (CO2) is the by-product of fuel combustion (coal, oil, gas, or even wood) that produces energy as heat or electricity. In addition, other non-combustion processes such as liquefying natural gas, manufacturing cement, producing steel, refining, and petrochemical manufacturing produce CO2. Heating and cooling associated with these processes also produces CO2. CO2 released to the atmosphere from point sources is a growing concern for industries – for example, investors are increasingly shopping for ventures with reduced CO2 liability, and incentives for CO2 emission reduction are developing.
In order for the world to meet its climate targets and prevent a rise of global temperatures by 1.5 degrees Celsius, carbon neutral and negative emission technologies need to be developed at a large scale. According to the Intergovernmental Panel on Climate Change, the world’s leading authority on the topic, carbon capture and storage (CCS) needs to account for 13% of emissions reduction by 2050. The International Energy Agency states that we need to upscale carbon capture and storage by two orders of magnitude from where it is now by 2050. Geologists have a prime opportunity and the skillset to contribute to the success of climate change mitigation.
There are many attractive options for CO2 reduction, from energy conservation to renewable energy source development. CCS is an emerging technology, with projects developed in the U.S., Canada, Australia, Japan, Brazil and other countries.
Although no projects have been developed in Colorado, the state’s geology is amenable to CO2 injection and suggests that CCS should be pursued by geologists in the state. The two basins discussed here are potential targets for injection, given various properties that lend to high injectivity. CCS requires geology that has a variety of properties, allowing for easy injection without risk of pressure buildup, ensuring that CO2 is retained in the reservoir. Parameters like porosity, permeability, depth, isolation from other natural resources, and others ensure that a rock formation is a likely candidate for safe, long-term storage.
For geologists, CCS is an attractive option. Upon capture, storage in sedimentary basins is a key component of the process. Many options are available for capture. The higher the CO2 concentration, the more attractive the supply. Nearly pure CO2 is available as the off-gas from ethanol manufacturing and from gas processing and gas purification for LNG. Other high concentration sources can be derived from refined products such as hydrogen or ethylene oxide. Unlike these high concentration options, conventional combustion dilutes the CO2 with air. Options to capture CO2 from such dilute mixtures include chemical and physical processes that effectively concentrate CO2, or alternatively, re-engineering energy production processes in various ways to produce a concentrated CO2 stream. CO2 from direct air capture (DAC) is the most dilute of sources, and this endeavor requires favorable engineering and clever processes to lower energy costs. In all cases, upon capture, CO2 is compressed to a dense phase and shipped via pipeline to a permitted storage site.
Selecting a storage site requires a geologist’s expertise, while designing the injection process is in the domain of subsurface engineers. The skills needed are similar to those in hydrocarbon exploration and production, with interesting reversals and modifications. It may be useful to repurpose a depleted oil or gas field; however, management of the isolation of many wells can be onerous and lead to high long-term leakage risks. It is therefore also desirable to consider storage in areas that never trapped hydrocarbons, known as deep saline formations (DSF). Other sequestering options such as CO2 enhanced oil recovery (EOR) are well established in depleted hydrocarbon fields. More novel options such as storage in fractured shale or cleated coal, mineral trapping, or various forms of enhanced reaction or dissolution also require geotechnical skillsets; however they are not the focus for our discussion here.
Scoping a suitable storage site must be done with care as the natural characteristics of the injection area provide most of the assurance of long-term storage. Top selection criteria are a suitable high transmissivity (high permeability over a suitable thickness) with sufficient lateral continuity to accept large amounts of CO2. Injection of 0.5 to 1.5 million metric tons per year per well, is generally required to provide favorable economics. As more wells are required to obtain the injection rate, the value of storage resource drops. If the reservoir has not held hydrocarbons, the issues of viability of a seal becomes important to assess. However, CO2 is more soluble than hydrocarbons and has approximately equivalent migration losses because of pore-scale trapping. Reservoirs that greatly retard migration of CO2 over 1,000 year time frames may be acceptable, even though they did not retain oil over geologic time scales. In addition, many other factors such as protection of freshwater and other resources and avoidance of induced seismicity require geotechnical consideration during development.
Not all the reservoirs in the Rocky Mountains have been assessed for CO2 storage. Some samples can be considered in the University of Texas’ database at: https://www.beg.utexas.edu/gccc/ CO2-data/ data-main.
DENVER BASIN, LYONS FORMATION
Two Colorado injection targets are considered here (Figure 1).
In the south-central part of the Denver Basin, the Permian Lyons Sandstone was evaluated based on a literature review of CO2 storage potential. Overlying the Lyons Formation, the porous sandstone units from the Triassic (Dockum sandstone), the Triassic-Jurassic (Jelm-Entrada sandstone), and the Cretaceous Dakota Group likely favor CO2 injection as well, making the Denver Basin a highly favorable “stacked storage” prospect. The Lyons sandstone occurs at favorable depths (2,400 to 400 ft) for CO 2 storage and has suitable thickness (200 to 600 ft) with high-net sandstone to the south. The well-sorted near-shore and eolian depositional environments likely create high injectivity. Further study is needed to assess the stratigraphic complexity that favors trapping. In addition, the diagenetic history of calcite, quartz and iron oxide precipitation that limit injectivity are barriers to some parts of the Lyons Formation. 25 to 160 feet of Triassic-age Lykins red shale and siltstone, evaporite, and carbonate are likely to form a good quality top seal on the Lyons. The interference or benefit from major producing fields such as the Black Hollow and Pierce fields would need to be evaluated. As is typical of many Rocky Mountain basins, the flow system in the Lyons Formation is dynamic. Fresh water less than 10,000 M/L TDS is protected from CO 2 injection. It would be critical to assess if this standard can be met.
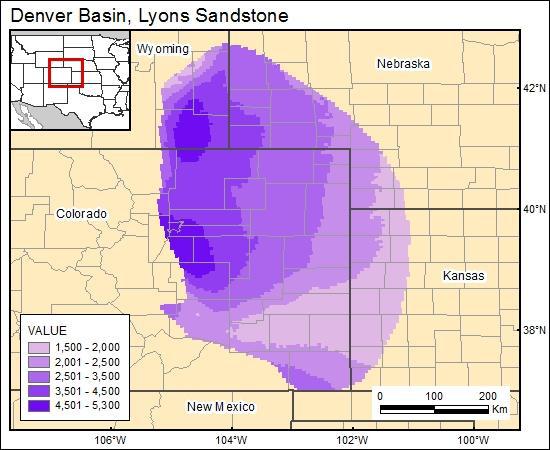
FIGURE 1: A GIS map of the depth of the Lyons Formation shows the regional extent of the formation in Colorado, Kansas, Nebraska, and Wyoming.
SAN JUAN BASIN, MORRISON GROUP
The San Juan Basin (Figure 2) is another prospective storage location. To explore its potential, we consider the stratigraphically complex Morrison Group. The Morrison Group has favorable transmissivity of up to 5 m/day, excellent thickness of 50 to 350 m, and is most suitable for storage at depths of 1.5-3 km. Reservoir complexity is expected to be high where the depositional environments are fluvial. This is favorable for trapping CO 2 but will require sufficient exploration to locate “sweet spots’’ for injection. Mudrock zones within the Morrison Group can serve as confining layers. For example, the Brushy Basin Member of the Morrison Formation, composed of green, olive, and maroon mudstones, is a suitable top seal. Because the mudrock zones are variable in thickness and lithology, detailed study would be needed at the site-scale to identify permissible storage sites. Like the units in the Denver basin, the hydrocarbon resources in the Morrison are both a benefit and a risk. To the former point, they provide high-value, dense data, not only on rock properties but the flow of fluids within them. Additionally, depleted fields like the San Juan Basin can be considered as EOR candidates or for proven storage volumes. However, the quality of wells that penetrate the Morrison are a containment risk that must be evaluated with care, as any interference with remaining hydrocarbon resource may limit the conflicting use of the subsurface. Like the Lyons sandstone, fresh water recharge areas at the basin margins require protection and will also limit the viable injection targets, although the deep basin is quite saline. Lastly, the mineralogically complex rocks may react with CO2, providing additional trapping potential.

FIGURE 2: A GIS map of the depth of the Morrison Group shows the regional extent of the formation in Colorado, New Mexico, and Arizona.
ADDITIONAL STORAGE SITES
The Glen Canyon Group in Western Colorado is more problematic. The rock properties of the thick, clean eolian sandstones in the group are excellent for injection, with a suitable top seal in the Carmel-Twin Creek units. However, in most areas, the units occur at too shallow a depth to be suitable for storage and also have active fresh water recharge so that fresh waters would be protected and unavailable for injection. More exploration is needed to assess the structurally deepest part of these units as well as the suitability of older units beneath them.
SOME BACKGROUND ON GULF COAST CARBON CENTER (GCCC)
GCCC is a research consortium in Austin, Texas that is a world leader in CO2 storage technology. Researchers at GCCC study the pressure buildup and migration of the fluid through the potential storage reservoirs to ensure safe storage that complies with local and federal regulations. The GCCC is a research consortium within the Bureau of Economic Geology, the state geological survey, at the University of Texas at Austin. The GCCC has been around since 1998 and initiated the first U.S. CO2 injection not intended for oil recovery as part of the Frio Brine Pilot Experiment, funded by the Department of Energy.