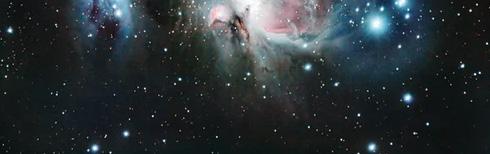
7 minute read
Diffuse Interstellar Bands
Diamonds in the Diffuse Interstellar Bands: The Rough Graveyard of Many a Career
By Tom NealBy Larissa Aravantinou
Advertisement
Space is filled with stars and planets. Between these celestial bodies are gaps filled with thinly spread gas and dust known as interstellar dust clouds. Through telescopes scientists can take a look into space and use measurements of electromagnetic radiation to determine which particles are present in the interstellar medium. This is possible because atoms and molecules interact with radiation in different ways. Consequently, different atoms and molecules have characteristic patterns of absorption. Scientists can then use this information to match absorption patterns of known atoms or molecules with the patterns observed in space.1
But what if the absorption patterns in space don’t match to those of any atom or molecule known to scientists? That is exactly the case with the Diffuse Interstellar Bands (DIBs).
The Diffuse Interstellar Bands are absorption bands belonging to interstellar material in space known as carriers. There are over 500 DIBs that range between 4000 Å to 10000 Å as shown above. They have baffled scientists for over 100 years since their first discovery by Mary Lea Heger in 1919. This is because despite many DIBs having been detected, there is only one molecule that has been found to match two diffuse interstellar bands at 9577 Å and 9632 Å - and that is the C60+ buckminsterfullerene cation.2
The confirmation of C60+ as a carrier in 2015 signified a breakthrough in the research of diffuse interstellar bands and the understanding of the interstellar medium. It meant that larger, more complex structures may exist in interstellar space compared to what was previously thought. There may even be molecules in the interstellar medium that have never been observed on Earth!2
Astronomers assume that some of the DIB carriers may be polyaromatic hydrocarbons or other larger fullerenes. However, btaining laboratory evidence to confirm these carriers is extremely difficult since it requires scientists to essentially replicate the conditions of deep space in a lab. The molecules have to be isolated in temperatures as low as 4.2 K in neon or helium matrices in order to obtain their gas phase spectra and compare them to absorption spectra from space.3
Moreover, whilst there is some clue as to the identity of the DIB carriers, nobody really knows how they actually got there. So far speculations suggest that they are formed in the atmosphere of carbon rich stars and are then carried into space by the star’s stellar wind. However this is far from conclusive.3 There is still a lot to be uncovered in regards to the chemical and physical reactions occurring in the interstellar medium and in space. Whilst astronomers are one step closer to identifying carriers for the DIBs, the fascinating century-old mystery continues.
1. https://bit.ly/3dsSfj5 2. https://bit.ly/32qlz3u 3. https://bit.ly/32qlz3u
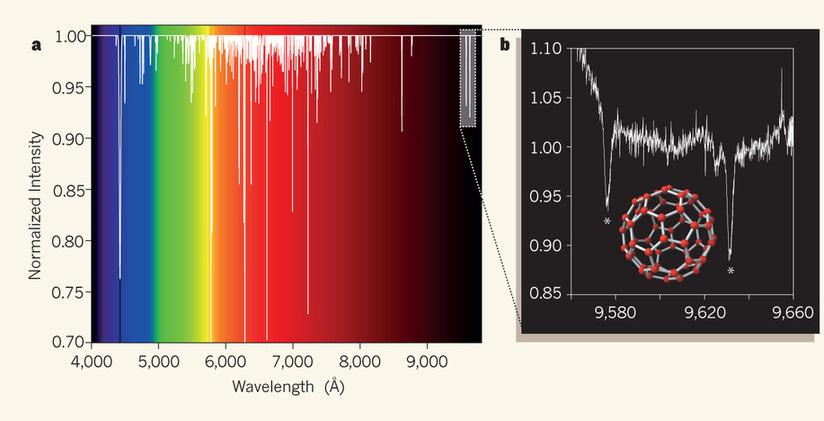
Infrared Spectrum detailing the apearance of diffuse interstellarbands.1
Female astronomer Mary Lea Heger, who made important discoveries in interstellar medium.
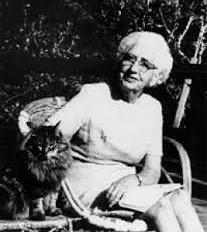
The Future of Sustainable Polymer Production
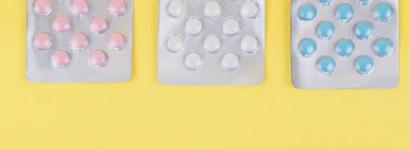
By Ida Shahriari Zavareh By Ida Shahriari Zavareh
There is a significant need to discover more sustainable and affordable polymer production mechanisms, due to the everincreasing plastic waste problem and environmental devastation from the burning of fossil fuels.
PHAs or polyhydroxyalkanoates are leading sustainable polymer alternatives. They posess the ability to be converted into carbon dioxide and water in the presence of oxygen by microorganisms, and they can be synthesised from renewable carbon sources.1
Poly(3-hydroxybutyrate-co-3hydroxyvalerate) or PHBV is a type of PHA. PHBV is a biodegradable copolymer which is produced through a controlled fermentation process using microorganisms. Its main use is in the drugs industry, but not for the drugs themselves, but instead they are used in the creation of the packaging.1
The polymer backbone is made of carbon and oxygen atoms, allowing PHBV to undergo bacterial degradation into carbon dioxide and water which is favourable in many medical applications. PHBV is a thermoplastic copolymer made up of the monomers, 3-hyrdoxybutanoic acid and 3-hydroxypentanoic acid which are also known as 3-hydroxybutyrate (PHB) and 3-hydroxyvalerate (3HV), respectively. These monomers are joined together by ester bonds through condensation reactions. The monomer units are synthesised simultaneously in bacterial cytoplasm.1
The different ratios of the two monomers result in different properties of PHBV. As the fraction of 3HV monomer in the polymer increases the degredation rate and melting point of the resultant PHBV also increases. This increase can be atributted to the low crystalinity of the biopolymer.1
In addition to its favourable biodegradable and renewable qualities, PHBV also has many outher advantageous qualities. It is non-toxic, has biocompatiblity with many types of cells, a high degree of crystallinity, it is resistant to ultraviolet radiation and posesses high surface tension and flexibility.1
However, PHBV applications are limited due to its poor mechanical properties compared to conventional polymers. it has increased fragility and low impact resistance while having relatively high production costs, making it extremely cost ineffective.
PHBVs biodegradability and renewable qualities cause it to lack mechanical strength, porosity, electrical and thermal properties. However, new advancements altering the polymer chemistry have improved these qualities. These enhancements will help the shift from packing based on fossil fuels to more renewable polymer production.2
If PHBvs have any place in the sustainable polymer market then solutions to their poor mechanical properties must be found. Introducing crosslinking with other long chain molecules or altering the 3HV fraction has been seen to enhance PHBVs mechanical properties. With more progressive research, more sustainable and affordable solutions will be developed. All of which are critical in the fight against environmental devastation.2
1. https://bit.ly/2SGXHaf. 2. https://bit.ly/3eA
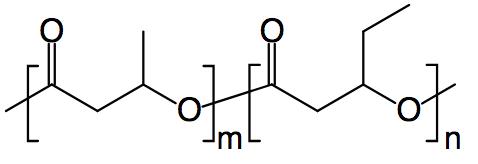
PHBV monomer repeating unit.
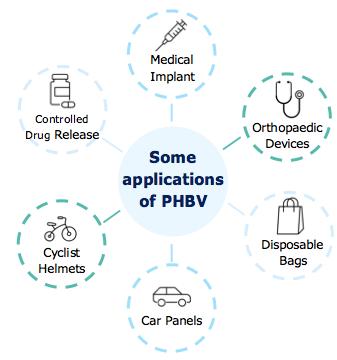
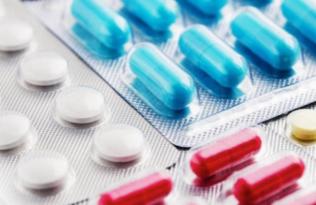
PHBV widely used in drugs packaging.
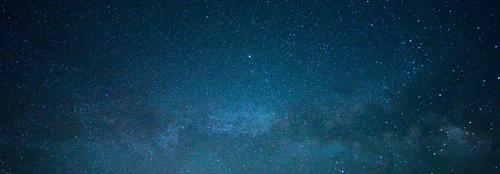
Homes Under the Printer: The Future of Construction
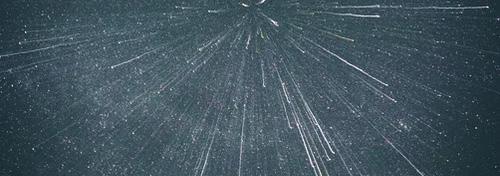
By Zak Pinkney By Zak Pinkney
From toys and artwork to human organs and houses on mars, the scope for 3D printing is limited only by the imagination of the designer. With so much potential, what does the future of 3D printing have in store for us?
While it is somewhat unlikely that 3D printers will become as ubiquitous as laser printers, most people will encounter a 3D printer in one form or another as a direct result of their functionality, which it owes largely to the range of materials that can be used. From metals such as titanium and stainless steel, to thermoplastics and carbon fibre, the gamut of materials possible is only set to increase as materials science develops new and exciting mediums to construct with.
SpaceX and NASA have the goal of colonising Mars within the next 10 years, so we need a relatively inexpensive method of building habitats for crews to live in.1 Enter 3D printing, with completely automated in-situ construction; large scale 3D printers can be sent to Mars to construct the habitats in preparation for the arrival of humans.
In 2019 NASA concluded a multiphase challenge to construct sustainable 3D printed housing for deep space exploration. The winners being AI Space Factory with their ‘Marsha’ design, which utilises the ability to print using resources available on mars to cut the cost of sending construction materials. The habitats will be constructed out of a mixture of basalt fibre (found in Martian rocks) and renewable bioplastics (Polylactic Acid, PLA) which would be processed from plants grown on Mars.1
Unlike the brick-and-mortar houses on earth, homes on Mars would need to be able to withstand the chilling thermal stress of -60 oC and internal atmospheric pressure for the inhabitants, requirements that are met by the composite used. This combination of basalt fibre and PLA was proven by NASA to be 2-3 times stronger than concrete in compression. The PLA bioplastic is also an effective shield for ionising cosmic radiation due to its low atomic weight. It also has a low coefficient of thermal expansion which is imperative for creating a basalt fibre composite. The emissions released from PLA formation are benign – unlike petrochemical plastics.2
Following the ‘Marsha’ Martian house design, AI Space Factory have designed a similar concept called ‘Terra’ for sustainable low-cost housing on earth. Using the same autonomous 3D printing strategy alongside the use of composite materials, it is easy to see how our traditional construction techniques may be replaced.2
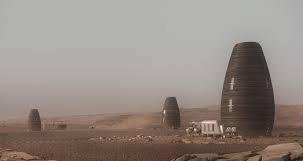