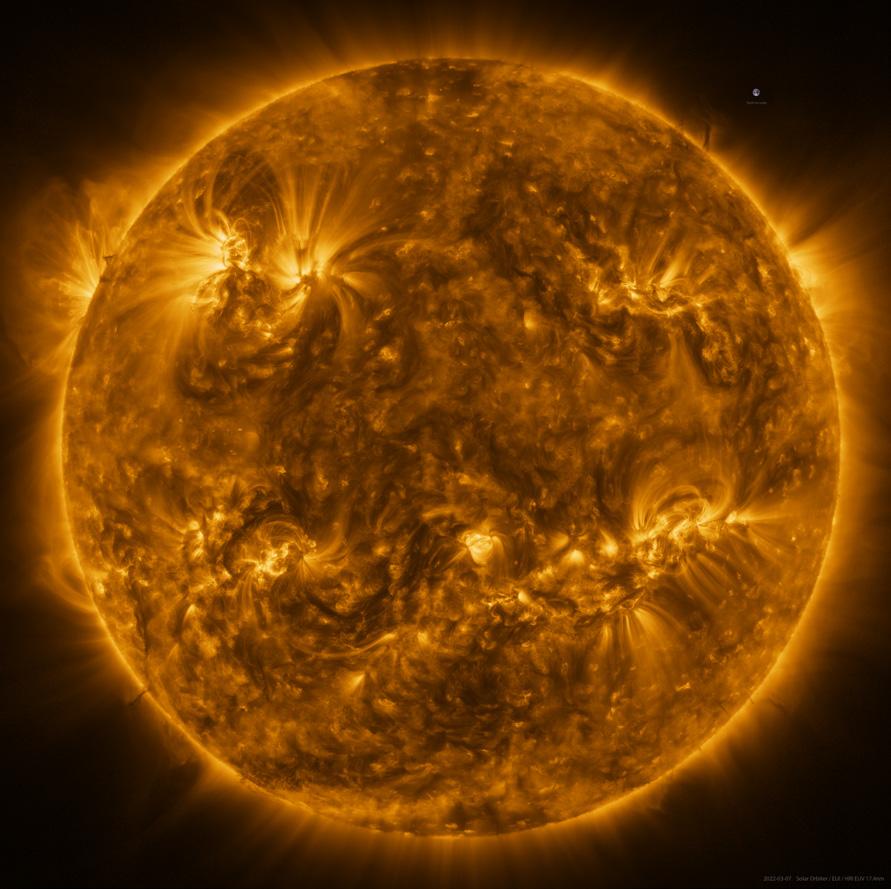
12 minute read
Let’s talk about... solar physics
from Contact 11
Let’s talk about... solar physics
BY CASSANDRA CAVALLARO (SKAO)
Solar missions are very hot right now. In March, the ESA- NASA Solar Orbiter made its first near fly-bys, around 48 million km from the Sun, inside the orbit of Mercury. Equipped with multiple imaging instruments, it is creating the closest images ever of the Sun’s surface.
Only a few months earlier, NASA’s car-sized Parker Solar Probe flew through the Sun’s corona, its upper atmosphere, in another historic first. Subsequent fly-bys will take Parker even closer, ultimately getting to within 6.2 million km of the Sun’s surface, more than seven times closer than any other spacecraft has been before.
While these heat-defying space missions will bring a wealth of new information, they’ll be complemented by insights gleaned from radio astronomy observations, including those using the SKA telescopes.
One might imagine that there isn’t much light that still needs shedding on the Sun, given its relative closeness to Earth, but that is not the case.
“There are some topics which remain mysteries in solar physics even if we have understood the basics of the phenomena for several decades now,” says Dr Nicole Vilmer, a solar physics specialist based at Paris Observatory, and member of the SKAO’s Solar, Heliospheric and Ionospheric Physics Science Working Group. “To name a few: the generation of the solar magnetic field and the origin of the magnetic cycle, the formation and acceleration of the solar wind, and the production of energetic particles.”
THE SOLAR CYCLE
The Sun has an 11-year cycle of activity, and at a certain point the magnetic field lines flip, meaning north becomes south and vice versa.
Before jumping into the finer details, let’s briefly slice into the Sun to understand its structure.
At the Sun’s core, the temperature is around 15 million degrees Celsius. Here nuclear fusion is taking place: protons travelling at great speed smash into each other and fuse together, a process which ultimately turns hydrogen into helium and releases energy in the form of gamma ray radiation.
The energy is then transported from the core to the Sun’s “surface”, the photosphere, through radiative and then convective transport.
The photosphere is the luminous layer, and it is so bright that it outshines the chromosphere above it, which can only be seen as a red glow during solar eclipses. Next is the thin transition region, and finally the tenuous solar corona, the Sun’s outermost atmosphere. Stretching around 8 million km into space, the corona is a region of diffuse plasma (hot ionised gas) only seen during a total solar eclipse as a bright halo around the dark Moon, or with specialised instruments.
The corona is of particular interest to radio astronomers for a simple reason.
“The tenuous solar corona is completely transparent at optical and higher frequencies, but the low radio frequency solar emission arises exactly from this magnetised and turbulent atmosphere of the Sun,” says Associate Prof. Divya Oberoi of India’s National Centre for Radio Astrophysics (NCRA), co-chair of the SKAO’s Solar, Heliospheric and Ionospheric Physics Science Working Group.

The Sun as seen by the ESA-NASA Solar Orbiter in extreme ultraviolet light from a distance of roughly 75 million kilometres. It is the highest resolution image of the Sun’s full disc and outer atmosphere, the corona, ever taken.
Credit: ESA & NASA/Solar Orbiter/ EUI team; Data processing: E. Kraaikamp (ROB)
Puzzlingly, despite being further away from the core than the photosphere, the corona is more than 200 times hotter. This is known as the “coronal heating problem”.
“This is among the most enduring astrophysics puzzles of our times: how does the 5,800K photosphere sustain a million K corona above it?” Divya says. “We have known about this mystery for more than 70 years, and have some pretty good ideas about how it might come about, but as a global community we have been struggling to find the evidence for this.”
UNDERSTANDING KELVIN
Stellar temperature is measured in kelvin (K). Zero kelvin is absolute zero, or -273.15 degrees Celsius, the point at which particles stop moving. To convert a kelvin temperature to Celsius, simply subtract 273.15.
Scientists think the heat is coming from energy in the Sun’s magnetic fields, but the mechanism that transfers that energy to the corona and heats it up is unclear. Solar magnetic fields vary in strength, whereas the corona is searingly hot all over, all the time. This suggests that whatever is causing coronal heating must happen regularly all over the Sun, rather than intermittent expulsions of energy like dramatic solar flares.

A coronal mass ejection on Feb. 27, 2000 taken by SOHO Large Angle and Spectrometric Coronagraph Experiment (SOHO) C2 and C3 instruments.
Credit: SOHO ESA & NASA.
One theory, first put forward in the late 1980s by the renowned American astrophysicist Prof. Eugene Parker (who died earlier this year, and for whom the Parker Solar Probe is named), is that socalled “nanoflares” are behind it.
“Prof. Parker proposed that the corona is maintained at its million K temperature by a very large number of extremely small explosive releases of energy in the corona – nanoflares – too weak to be detected individually but which can collectively deposit sufficient energy to heat the corona,” Divya explains.
“Efforts to detect the signatures of nanoflares have focused on X-ray and ultraviolet observations, but radio observations are very promising too. That’s because while X-ray and ultraviolet observations are usually dominated by thermal emissions, in the radio band we see signatures that arise from non-thermal, coherent emission processes.”
“Coherent” emission is concentrated in a few frequencies, while incoherent, thermal emission is spread out over a large bandwidth.
RADIO EMISSION EXPLAINED
Imagine a set of radio waves travelling through space.
The wave form is known as the “phase”.
“Relative phase” is the difference between two waves’ points in that up/down cycle.
“Coherent” radio emission means the frequency of the emission is all the same, and the relative phase differences between any two waves of the emission is constant.
“The coherent nature of radio emission means that despite the low energy of the phenomenon which gives rise to them, the radio emissions can be much brighter. While this has been known for a while, these observations of radio signatures of nanoflares are very challenging and have simply not been feasible with traditional radio telescopes. This contrasts with general solar radio bursts which have been studied in great detail with the earlier and present generations of instrumentation.”
Divya’s team has made promising progress on that front recently using the Murchison Widefield Array (MWA), a low-frequency SKA precursor telescope located in Western Australia. They detected tiny flashes of radio light coming from all over the Sun, even from “quiet Sun” areas where magnetic fields are the weakest. This is considered among the strongest evidence yet that nanoflares might be behind the coronal heating mystery.
The magnetism that is believed to drive coronal heating is also the cause of dramatic explosions. The twisting and realignment of magnetic field lines can cause sudden bursts of electromagnetic radiation known as solar flares, and coronal mass ejections (CMEs) which comprise large amounts of energetic plasma and associated magnetic fields.
“Magnetic fields are now understood to be the driver of most solar phenomena,” Divya says. “They are however notoriously hard to measure and radio observations provide some of the most promising ways to estimate them. That’s because the magnetic fields permeating this turbulent region leave tiny imprints on these radio waves by changing their polarisation properties.”
Radio astronomers can measure this change in the received radio emissions and, in principle, estimate coronal magnetic fields.
“The Sun is not only a very interesting object to study by itself,” Nicole adds. “It is also crucial to understand its activity since it affects our terrestrial environment and may affect our technology through what we call space weather activities.”
Ah, space weather. A slightly innocuous term for something that can have dramatic consequences.
The Sun’s influence permeates through the Solar System via an enormous bubble of plasma called the heliosphere. This has a vital protective role, shielding our cosmic neighbourhood from the much stronger radiation of interstellar space, a region only NASA’s iconic Voyager probes have ventured into, recording the drastic change in environment that lies beyond. The heliosphere is fed by the solar wind, a constant stream of plasma from the corona.

ASSOCIATE PROF. DIVYA OBEROI, NCRA
“The processes by which energetic particles are transported from acceleration regions in the low corona to the heliosphere are still a subject of interest and are one of the key questions to be addressed by NASA-ESA Solar Orbiter,” says Nicole. “The heliosphere is also the only place where remote sensing observations [analysing electromagnetic radiation] in a wide range going from radio to X-ray, can be combined with direct measurements, such as energetic particle measurements taken in space, to study plasma physics processes in a natural plasma, rather than plasma created in a laboratory.”
When the Sun has a violent outburst, like a CME, this can travel all the way to Earth. At their most harmless, the effects are seen as colourful and spectacular auroras that illuminate the northern and southern skies at high latitudes. But at the other extreme they can cause electrical black outs, disrupt radio and GPS communications and damage satellites. One of the most famous examples occurred in March 1989, when a huge coronal mass ejection led to a 12-hour electricity blackout across the whole Canadian province of Quebec.
“Only a few of the CMEs are ‘geoeffective’ and currently we have a very limited ability to reliably predict which ones will impact us adversely. The most crucial parameter to measure for is the direction and strength of the CME magnetic field,” Divya explains.
“Currently our most reliable measurements come from satellitebased instrumentation located about 1.5 million km from Earth along the Sun- Earth line, which gives us less than an hour’s notice before the more energetic CMEs impact our environment. We are working towards improving this by using radio telescopes to measure how the magnetised plasma of CMEs changes the observed polarisation of background radiation (a process called Faraday rotation) while it is still halfway between the Sun and the Earth, which could increase our advance warning time to about a day.”
While radio telescopes can be powerful instruments for solar studies, this type of science faces some unique challenges, not least because the Sun is enormous and extremely active.
The Sun’s apparent size in the sky (known as “angular size”) is even bigger when observing in radio than in visible light, close to 1 degree across if observing in the lowest frequencies covered by the SKA-Low telescope, Divya explains. That’s around double the size of the Moon in the sky.
“It also has elaborate structures spanning a large range of angular scales, and the solar radio emission can be extremely variable, so the usual tricks of observing a source for long durations or across wider bands to improve imaging quality are simply not useful for the Sun. To add further complications, multiple different emission mechanisms often operate simultaneously on the Sun and emission strengths can easily vary by more than nine orders of magnitude between them.”
This all demands a special approach not usually used to observe other objects. To explain it, we need to get a bit technical. Over to Divya.
“Some of the most interesting information, both from space weather and coronal heating perspectives, is buried in the comparatively weaker emissions or features. So to meet the science needs, one requires images with very high fidelity (meaning no information is missing and no errors have been introduced) and dynamic range (which improves the contrast between different types of emission happening at the same time). If you have these things, you can reliably detect and characterise faint emission features even in presence of those a million times stronger.”
The method radio astronomers will use is called “high fidelity and dynamic range spectropolarimetric snapshot imaging”. Quite a mouthful, but in simple terms it means making independent radio images of every tiny slice of the frequency channel being observed for every time slice in the data. In many other areas of radio astronomy an average is used instead, because most objects aren’t as variable as the Sun on these scales, nor as bright – in fact it’s this brightness that enables scientists to divide up the observations so finely, as even these fine slivers of solar signal outshine the noise that can obscure fainter sources. This technique is already paying dividends using other telescopes, and the SKA will be able to do it on a grander scale.
“The SKA telescopes are uniquely suited for solar science and will enable us to make solar radio images of unprecedented quality. What makes the telescopes exceptional is the large number of elements in the arrays, much larger than any other earlier instrument. This ability to gather an unprecedentedly large amount of information about the solar radio emission will give the SKA telescopes their cutting edge,” Divya says.
As the Sun emits across such a wide part of the spectrum, SKA observations in the radio band can also provide a fuller picture when combined with those from other facilities, particularly those in space.
“If the SKA provides regular solar observations in a manner that can be easily used by solar physicists (not necessarily radio astronomers), it will provide complementary observations, for example of flares, which can be combined with X-ray or extreme ultraviolet observations provided by the Solar Orbiter or other solar space probes,” Nicole adds. “The radio observations can also be combined with coronagraph observations on space probes to explore the early development of CMEs.”
Then, of course, there are the breakthroughs that we cannot anticipate.
“We must not forget that every time we make observations finer than have been possible with earlier generations of instrumentation we have always found unexpected discoveries and phenomenon,” Divya adds. “So we very much expect this trend to very much continue with the SKA telescopes as well.”

A coronal mass ejection (CME) travelling at 900 miles per second in August 2012, which caused aurora to appear in our skies a few days later.
Credit: NASA/GSFC/SDO