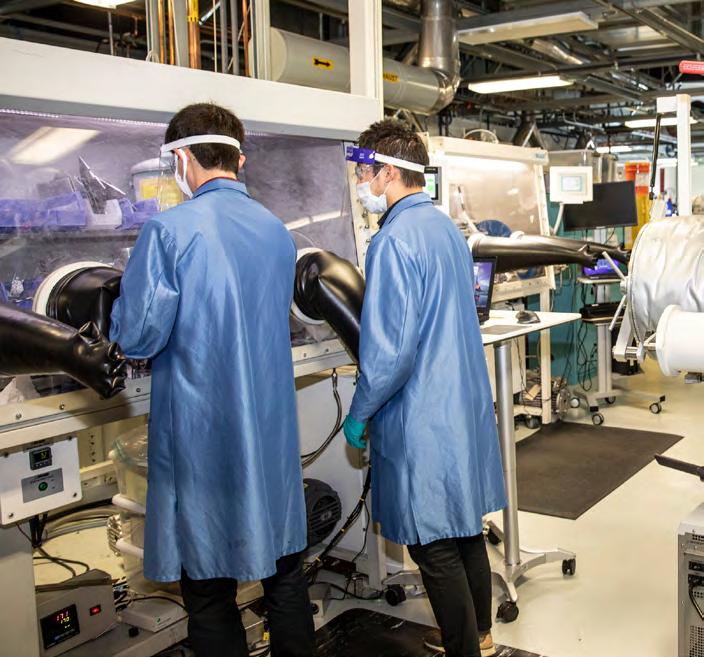
9 minute read
Solid-State Technology
An Investigation into the Development of Electric Vehicle Batteries
As the search for safer and more efficient electric vehicle (EV) batteries continues, solid-state technology emerges as an attractive topic. Solid-state batteries (SSBs) are viewed as the next paradigm leap in battery technology, offering potential benefits above traditional lithium-ion batteries. Nevertheless, they face significant technological obstacles.
Positive features of solid-state batteries
1. SSBs can store more energy than regular lithium-ion batteries. This is because solid-state electrolytes contain more lithium and allow for the use of lithium-metal anodes with higher capacities.
2. Solid electrolytes used in SSBs are generally non-combustible, which decreases the risks of overheating and thermal runaway. This intrinsic safety may eventually result in reduced battery pack cooling and protection requirements, lowering the weight and cost of electric vehicles.
3. Extended Durability: SSBs are less susceptible to deterioration than liquid electrolyte-based batteries. This results in an extended operational time, which may lead to batteries that outlast the vehicle's lifespan.
4. Furthermore, solid-state technologies may enable faster charging times. The absence of liquid electrolytes in contemporary batteries considerably reduces the likelihood of lithium dendrite formation, which is a critical issue limiting rapid charging.
Technological obstacles
1. Material Compatibility and Interface Difficulties: One of the most difficult aspects of SSBs is maintaining stable interfaces between electrodes and solid electrolytes. Material degradation and interface resistance are two concerns that can impede a battery's performance.
2. Manufacturing and Scalability: At the moment, it is challenging to produce SSBs at a scale suitable for automotive applications. These batteries' manufacturing processes are more complex and costly than those of traditional lithium-ion batteries.
3. Temperature Sensitivity: Certain solid electrolytes lose functionality when exposed to lower temperatures. To utilize SSBs in electric vehicles, it is necessary to develop materials that maintain a high degree of ionic conductivity across a wide temperature range.
tive recycling technologies reduces reliance on basic material extraction while also addressing waste issues.
Application of Renewable Energy in Mining
Implementing renewable energy sources in mining operations is a significant step toward sustainability. By incorporating solar, wind, and hydroelectric power into mining operations, the sector not only contributes to the electric vehicle (EV) industry's general goal of reducing carbon footprints, but it also reduces greenhouse gasses.
Projects of Community Participation and Rehabilitation
Furthermore, sustainable mining needs reclamation activities and strong community engagement. By adopting post-mining land use planning options such as ecosystem restoration and community space development, the mining legacy can be altered for the betterment of the environment.
Understanding the complexities of the supply chain for critical materials
The electric vehicle (EV) revolution is dramatically changing the mining industry, resulting in the convoluted nature of essential material supply networks. In addition to increasing output, the challenge is efficiently negotiating geopolitical intricacies and ensuring a stable and diverse supply.
Geopolitical and commerce interdependencies
Geopolitical difficulties have a significant impact on the supply chains for commodities like nickel, lithium, and cobalt. These resource-rich countries usually wield significant power over the global supply chain, exposing them to potential vulnerabilities in the event of political unrest or trade wars. Political instability in regions such as the Democratic Republic of the Congo, a major cobalt supplier, has had an impact on worldwide prices.
Sources of Critical Material Diversification
Diversifying the sources of crucial minerals is critical to mitigating these risks. This necessitates establishing international collaborations, investing in countries with undiscovered resources, and exploring new mining sites. Diversification of this kind not only enhances supply chains, but it also reduces reliance on a single supplier, allowing for price and supply stability.
Advances in Technology for Supply Chain Management
In addition, technology is critical for supply chain optimization. Blockchain technology has the potential to promote ethical procurement practices by enabling transparency and traceability. Furthermore, supply and demand trends can be forecasted using AI-powered predictive analytics, allowing for more effective and flexible supply chain management.
Human Rights and Ethical Sourcing Considerations
Furthermore, ethical procurement is critical, particularly for elements like cobalt, which have been related to human rights issues. To avoid involvement in human rights breaches, responsible procurement methods require extensive supply chain auditing and strict adherence to international standards.
An in-depth examination of the engineering of EV battery materials.
The creation of batteries for electric vehicles (EVs) requires a complex interplay of materials science, chemistry, and physics. The fundamental operation of these batteries is dependent on the properties and conditions of the constituent materials, which primarily include the cathode, anode, and electrolyte.
Materials and Properties of the Cathode
The cathode is a critical component that determines the battery's capacity and voltage. Typically, it is made of lithium metal oxide. Lithium nickel manganese cobalt oxide (NMC), lithium iron phosphate (LFP), and lithium cobalt oxide are all common cathodes. Each material offers a distinct combination of energy density, durability, and security. For example, NMC is known for its extremely high energy density, making it ideal for longer-range propulsion systems.
Materials and function of the anode
In the majority of modern EV batteries, the anode is made of graphite, which serves as a lithium ion host. During battery charging, lithium ions go from the cathode to the anode and are stored in the graphite layers. The effectiveness of this technique is important to the overall functionality of the battery. Ongoing research is being conducted on alternate materials like silicon, which has the ability to hold a higher amount of lithium ions but faces expansion and durability challenges.
Electrolyte: The Metallic
During charging and discharging, lithium ions move between the cathode and anode via the electrolyte. The typical composition consists of lithium salt dissolved in organic solvent. Critical electrolyte properties include ionic conductivity, stability, and safety. Solid-state electrolytes are being investigated as a potential substitute for liquid electrolytes in order to improve energy density and safety.
Safety and Thermal Management Considerations
Effective thermal management during operations is critical to assuring safety and efficiency. Thermal runaway, which occurs when heat accumulates, might cause the battery to burn or detonate. Strict thermal management systems are essential for maintaining optimal operating temperatures; they include heat sinks, cooling surfaces, and thermal interface materials.
Materials and Technologies of Tomorrow
Emerging technologies, such as solid-state batteries, aim to boost energy density and safety by utilizing solid electrolytes. Alternative research projects focus on the use of sulfur-based cathodes to increase energy storage capacity and the development of lithium metal anodes.
Present State of Development solid state Batteries
Several major battery and automobile manufacturers are investing heavily in solid-state battery technology. Although prototypes and small production lines have been constructed, the time has not yet arrived for mass commercialization. Manufacturing and material challenges must be overcome before SSBs can be used in mass-market EVs.
Environmental and Regulatory Navigation Regarding the Production and Disposal of Electric Vehicle Batteries
The rapid expansion of the electric vehicle (EV) sector has increased the importance of the regulatory framework that oversees battery manufacture and disposal. Emerging technologies, such as solid-state batteries and traditional lithium-ion batteries, present significant environmental challenges, emphasizing the need for a comprehensive regulatory framework.
2. Recycling Incentives and Mandates: Governments are increasingly focused on recycling activities. To encourage the recycling of electric vehicle (EV) batteries, which is crucial for the long-term management of the materials they contain, legislative measures and incentives are being implemented.
Impact of Battery Materials on the Environment
1. Carbon Footprint: While electric cars (EVs) have lower lifecycle emissions than conventional vehicles, the production of EV batteries contributes to carbon emissions. The battery manufacturing industry is making concerted attempts to reduce carbon emissions by combining renewable energy sources and improving manufacturing processes.
2. Land and Water Use Consequences: Mining for battery materials can have a significant impact on land and water resources. Implementing sustainable mining methods is critical for mitigating these environmental problems.
The Future of Solid-State Batteries
Regulatory Framework for Battery Production
1. Emission Standards and Material Sourcing: Emissions from battery production are regulated to ensure that resources are sourced responsibly. To reduce ecological impacts, lithium, cobalt, and nickel extraction, for example, must follow environmental protection requirements.
2. Workplace Safety Standards: Because of the hazardous material handling and potential chemical exposure risks, battery manufacturing plants are subject to stringent safety standards. These regulatory measures are primarily designed to improve the well-being of employees.
1. EV batteries must meet exact performance and quality standards, which include efficiency, durability, and safety. The aforementioned restrictions are critical in maintaining consumer confidence and ensuring the reliability of electric car technology.
Environmental Regulations for Battery Disposal and Recycling
1. Regulations restrict the disposal of lithium-ion batteries to reduce the danger of environmental pollution. Regulations mandate proper battery management and disposal to reduce the risks associated with hazardous waste.
Although solid-state batteries are still in the development stage, they have the potential to lessen environmental impact. The potential use of less hazardous elements, as well as the lack of liquid electrolytes, may help to improve battery manufacture and recycling safety. Nonetheless, under upcoming regulatory frameworks, the environmental impact of innovative materials used in solid-state batteries would require careful consideration.
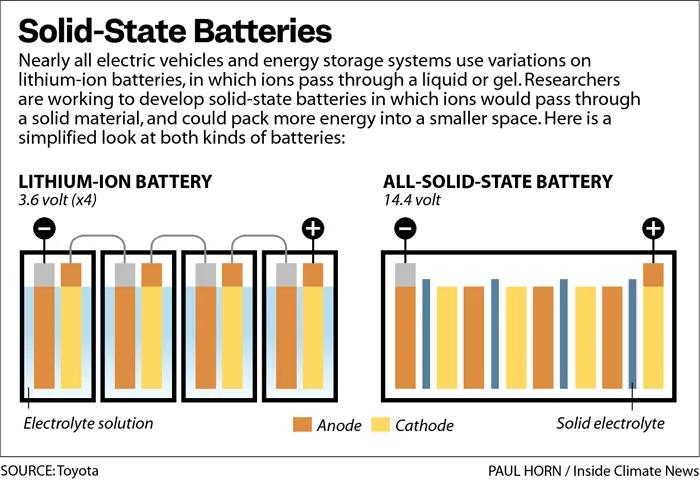
Future Prospects: Forecasting Advances in Mining and Electric Vehicle Battery Technology.
With the continued expansion of the electric vehicle (EV) sector, significant improvement is expected in battery technology and alternative materials research. These improvements have the potential to increase the power and effectiveness of electric vehicles while also having important implications for the mining industry.
Technological advancements in batteries
Current research and development in electric vehicle (EV) battery technology aims to enhance overall efficiency, reduce charging times, and boost energy density. Solid-state batteries have the potential to provide better energy density and improved safety than conventional lithium-ion batteries, which is a huge step forward. This transformation may result in a shift in primary resource demand, with worldwide implications for mining activities.
Investigation of Substitute Materials
To decrease or eliminate the use of scarce or expensive components like cobalt, the industry is looking at alternative materials in an effort to produce more environmentally re- sponsible and cost-effective solutions. Such efforts have led to the development of lithium-sulfur batteries and the spread of nickel-rich chemistry. As a result, the mining sector may reorganize as demand for certain minerals increases while demand for others decreases.
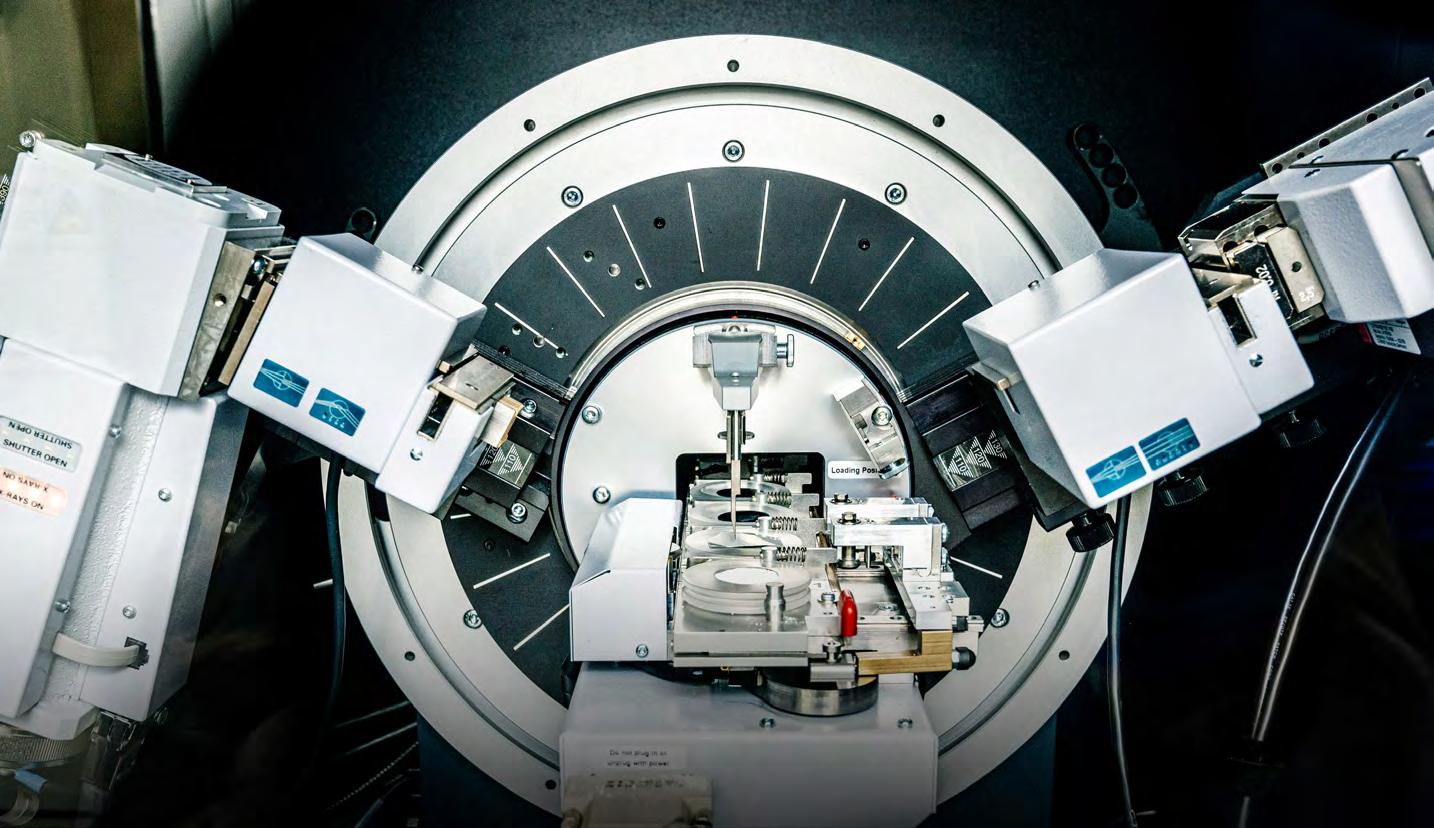
Impact on the Mining Industry
Technological advancements in the mining industry need the use of agile techniques and proactive planning. Mineral extraction, refining, and supply chain management techniques must change alongside battery technology. Investing in new mining technologies and supporting sustainable mining practices are critical for the industry to adapt to a changing environment.
Difficulties and Opportunities.
Although these transformations provide certain challenges, they also offer opportunities for progress and expansion in the mining industry.
New mining operations, particularly in places with unexplored alternative material resources, have the potential to boost economic growth and employment creation. However, this must be weighed against sustainable practices and environmental concerns.