
9 minute read
The Laser — Our Modern Lightsaber
The visionary gadgets used in Gene roddenberry’s creation Star Trek have had many fans suggest a foreshadowing of great technology advances – one such advance being the original cell phone with a flip top, that uncannily resembled the “communicator” used by Captain Kirk and crew in almost every episode of Star Trek, as well as in the subsequent movies. Not to be outdone, the Star Wars franchise (yes Sheldon, i used both of them in a single paragraph), brought to life by the imagination of George lucas also featured a recognizable technology element that was leveraged as the defensive weapon of the Jedi Knights. The keepers of the universe used lightsabers to do everything from deflecting lasers fired from blasters, to Force impacting hand to hand combat, to lighting pathways, to piercing metal “blaster doors.” The color of the lightsaber had meaning too, especially in the earliest releases, as the characters wielding red lightsabers were widely viewed as followers of the Dark Side, while our heroes and heroines that used blue lightsabers were representative of truth, integrity, hope, and all the good in The Force.
in Star Wars: Episode i – The phantom Menace, Qui-Gon Jinn is dispatched to settle a Trade Dispute and in the early parts of the movie he uses his lightsaber to turn thick doors into molten metal – as the thickness of the metal is increased by different layers being added, Qui-Gon must slow down his rate of cutting and double the focus on his lightsaber capabilities. Audiences loved the special effects – and may not even realize that in today’s industrial manufacturing environment, the use of light energy to cut metal is a very widespread reality. This light energy is from lasers – which itself is an acronym that stands for light Amplification by Stimulated Emission of radiation. First showing up on the cultural scene in a movie from the 1960s, as a weapon used against secret agent 007 James Bond by one of his adversaries in the movie Goldfinger, laser technology is being used today in automated cutting, engraving, measuring, and welding. And as amazing as it sounds, the color of the laser does indeed have meaning to the power that it yields and whether it can work with various metals. Most notably, improvements in blue laser technology are changing the game in welding capabilities for thin film highly reflective metals.
We went to www.explainthatstuff.com/lasers.html
(Woodford, Chris. (2006/2019) l asers. retrieved from https://www.explainthatstuff.com/lasers.html. [Accessed March 19, 2023]) to get some insight on the details of how lasers work. Before we discuss lasers and how they work, it's important to understand a bit about light and how photons can be created. initially, atoms in a stable state consist of a nucleus orbited by one or many electrons. When an atom absorbs energy, one or several electrons shift further away from the nucleus into higher energy levels, and the atom is said to be in an “excited” state. This is known as absorption. These electrons tend to move in an instant back to their original energy levels. When the electrons move back to their original energy orbits, they emit the excess energy in the form of a photon of energy, or packet of light.
Now, imagine if you will, firing a photon with just the right energy through several atoms to create this state, and ultimately causing one of the excited electrons to jump back down to its ground state, yielding both the photon we fired in and the photon produced by the electron's change of state. Because we're stimulating atoms to get radiation (not the harmful kind of radiation, but the light energy packets) out of them, this process is called stimulated emission. We get two photons out after putting one photon in, effectively doubling our light and amplifying it (increasing it). These two photons can stimulate other atoms to give off more photons, so, pretty soon, we get a cascade of photons—a chain reaction—throwing out a brilliant beam of pure, coherent laser light. What we've done here is amplify light using stimulated emission of radiation—and that's how a laser gets its name.” (Woodford, Chris. (2006/2019) l asers. retrieved from www.explainthatstuff.com/lasers.html. [Accessed March 19, 2023]) l asers can be created by stimulating the atoms in different forms of matter, including solids, liquids, or gasses. Generally crystalline ruby or other crystalline based solids are pumped with energy from high intensity light to generate a laser beam. For gasses, various compound gasses are generally used for a medium with the most common being CO2 excited by electricity. liquid mediums can be tuned to emit specific wavelengths which can be important when seeking different colors and power. Two other forms of lasers are semiconductor diode lasers and fiber lasers. Fiber lasers use an optical fiber cable made of silica glass, that has been doped with ion impurities, to guide light. The resulting laser beam is more precise than with other types of lasers because it is straighter and smaller.
A laser is a concentrated or coherent beam of light that is the same color. Each wave of light has the same length (wavelength) that has been amplified through the laser creation from the photons emitted. To make an electron jump from a lower to a higher level, you have to feed in a precise amount (quantum) of energy, equal to the difference between the two energy levels. When electrons flip back down from their excited state to their ground state, they give out the same, precise amount of energy, which takes the form of a photon of light of a particular color.
By guiding the light we can focus it down to very narrow beams. Stimulated emission in lasers makes electrons produce a cascade of identical photons—identical in energy, frequency, wavelength—and that's why laser light is monochromatic. The photons produced are equivalent to waves of light whose peaks and troughs line up (in other words, they are "in phase")—and that's what makes laser light coherent.
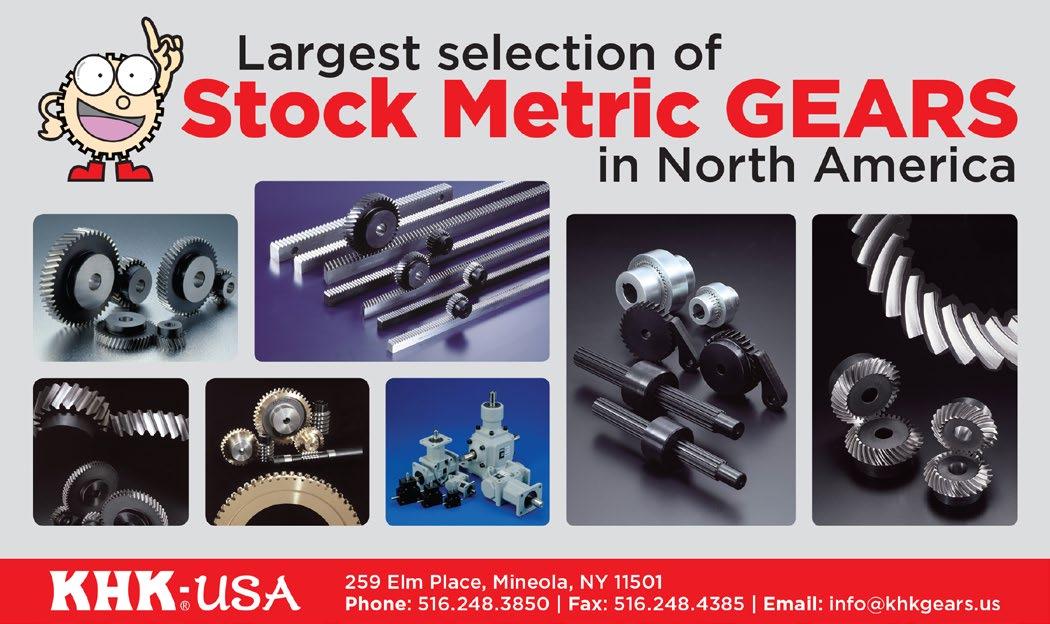
Within industrial manufacturing, CO2 lasers and fiber lasers tend to be the most widely used options. Crystal lasers are generally more expensive and more difficult to maintain than CO2 and fiber lasers. As the most recently invented, fiber lasers also tend to be the least expensive with a smaller footprint and generally are viewed as producing the highest power output for the power consumption required, yielding a more sustainable solution.
Metal cutting with lasers is a thermal separation process. The focused photon energy in the laser beam is absorbed in a thin surface layer of the metal. The absorbed energy is converted into heat that is intense enough to raise the temperature of the metal to its melting point or to a vaporization level. The molten “hole” is then moved through the metal cut – creating an area about the width of the laser called the kerf. A coaxial jet blast of assist gas is generally used to blow cut by-products through the hole. The underside of the cut is called the dross and this is where any remnants or burrs may occur. The intense kerf (cut) leaves behind an HAZ – or heat affected zone.
The primary factor for cutting speed and thickness is beam power or energy density. A faster rate of cutting though is also a compromise on the quality of the cut. While only 7-8% of the energy in a focused beam is initially absorbed, nearly 50% is absorbed in the molten metal. Coupled with pure oxygen or nitrogen as the assist gas, an
History of Industrial Lasers
extremely intense exothermic reaction occurs in the laser spot diameter, ultimately melting through the thickness of metal. The gas pressure ejects the molten matter from the kerf. This approach requires more critical focus on control of gas pressure, shape of the gas stream, and positioning of the gas nozzle orifice above the metal surface.
Ultimately the absorptivity of the metal will determine how well the cut or weld will perform. in most cases, fiber lasers operate in a visible ir light range, as do CO2 lasers. A fiber laser generates light in the 1,060 nm wavelength range, while CO2 operates in the 10,600 nm wavelength range. Whether a material can absorb light within the operating wavelength range is a large determinant for which type of laser to use. in general the smaller wavelength has more absorption across a broader set of metal materials and this can be seen in the advances in blue diode lasers with smaller wavelengths which are now enabling more effective cladding and welding with highly reflective metals such as aluminum and copper and gold alloy. The smaller wavelength can be more tightly focused as well, yielding higher power densities. On the Bystronic.com website, an analysis of the focused energy of a fiber laser vs a CO2 laser was dramatic. A 4400 watt CO2 laser could be focused down to a 2.2 MegaWatt/cm2 energy density, where a fiber laser could be focused to a 10 MegaWatt/cm2 energy density. When comparing it's easy to see if you are processing stainless, aluminum, brass or copper materials which are highly reflective (having a lower absorptivity), fiber laser technology is the fastest and most economical regardless of thickness.
lasers are one of several options for fabricators – and one that is very precise, but there are also others such as plasma cutting, waterjet cutting, CNC milling, and abrasive cutting. The choice of the most appropriate cutting method should be
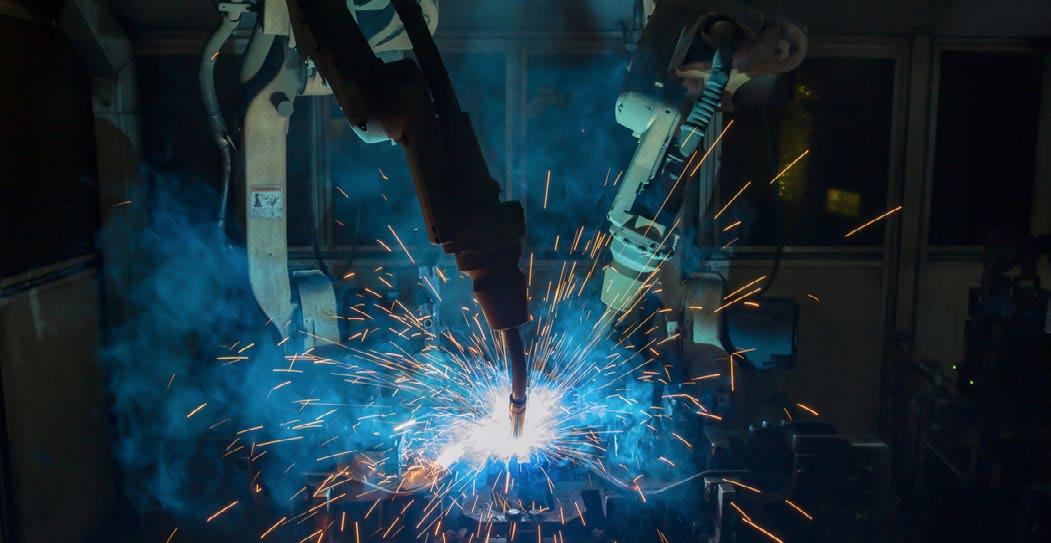
» 1917 – Albert Enstein developed the theory of stimulated emission of radiation
» 1951 – Charles Towns demonstrated the Maser - microwave amplification
» 1959 – Gordon Gould suggested that stimulated emission of radiation could also amplify light
» 1960 – Theodore Malman created the first ruby laser in a California laboratory.
» 1961 – Elias Snitzer invented the fiber laser
» 1962 – robert W. Hellwarth and r .J. McClung demonstrated Q-switching (pulsed laser beams)
» 1963 – Snitzer demonstrated the use of fiber lasers
» 1964 – Bell l abs scientist Kumar patel invented a gas laser thermal cutting process that used CO2 as a more effective solution over ruby laser.
» 1965 – Western Engineering research Center in Buffalo, New York uses lasers to drill die holes for electrical wires
» 1966 – Development of the fluorescent dye laser, making the color of the laser light freely selectable
» 1969 – Boeing uses gas laser cutting in commercial processes to cut titanium, Hastelloy, and ceramic.
» 1970s – Aerospace industry adopts gas laser cutting machines
» 1979 – prima industrie of Collegno, italy invents 3D laser cutting technique
» 1980s – Widespread use of gas laser cutting machines
» 1988 – First double-clad fiber laser is demonstrated by Snitzer (more efficient light guiding)
» 1990s – Widespread use of fiber laser technology based on the type of metal being used, the required precision of the cut, the thickness of the metal, and other factors. The cost of lasers varies depending on several factors such as power output, wavelength, manufacturer, and the specific application for which the laser is being used. Here is a rough estimate of the cost ranges for four types of lasers: it's worth noting that these are very rough estimates, and the actual cost can vary widely depending on several factors. recent advances have yielded a class of laser called Ultra High power lasers, which are generally above 10kW in power output. Additionally, the cost of laser cutting or other laser-based fabrication services will depend on a variety of factors beyond just the cost of the laser itself, such as the complexity of the project, the materials being used, and the desired precision and speed of the cutting process.
» Green disk lasers: These lasers typically have a power output in the range of 10W to 100W and a wavelength of around 532nm (nanometers are 10-9m). The cost for general green cutting lasers can range from $10,000 to $100,000 (generalized) and up. New advances have seen output (e.g. Trumpf TruDisk) with continuous wave power of 3kW and pulse power as high as 4kW.
» Blue diode lasers: These lasers typically have a power output in the range of 100mW to 5W and a wavelength of around 445nm. The cost for general blue lasers can range from $500 to $10,000 (generalized). l aserline Blue Diode lasers have continuous wave output at power as high as 3kW.
» CO2 lasers: These lasers typically have a power output in the range of 20W to 20kW and a wavelength of around 10.6 microns (microns are 10-6m). The cost for these lasers can range from $5,000 to $50,000 and up.
» Fiber lasers: These lasers typically have a power output in the range of 10W to 6kW and a wavelength of around 1,064nm. The cost for these lasers can range from $20,000 to $100,000.
As laser technology evolves, the power of light and the ingenuity of people will come together to create new solutions now and continue to innovate – maybe even getting to that lightsaber concept soon. Some companies and resources that will be helpful for you in your search for the perfect laser cutting equipment at your facility are:
» l aserax
» www.lasersystemseurope.com
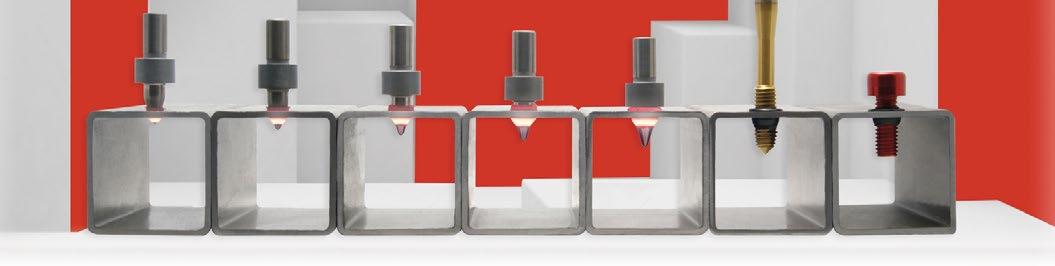
» Thunder l aser USA
» Bystronic
» l aserline
» Trumpf
SOME MATERIAL FROM