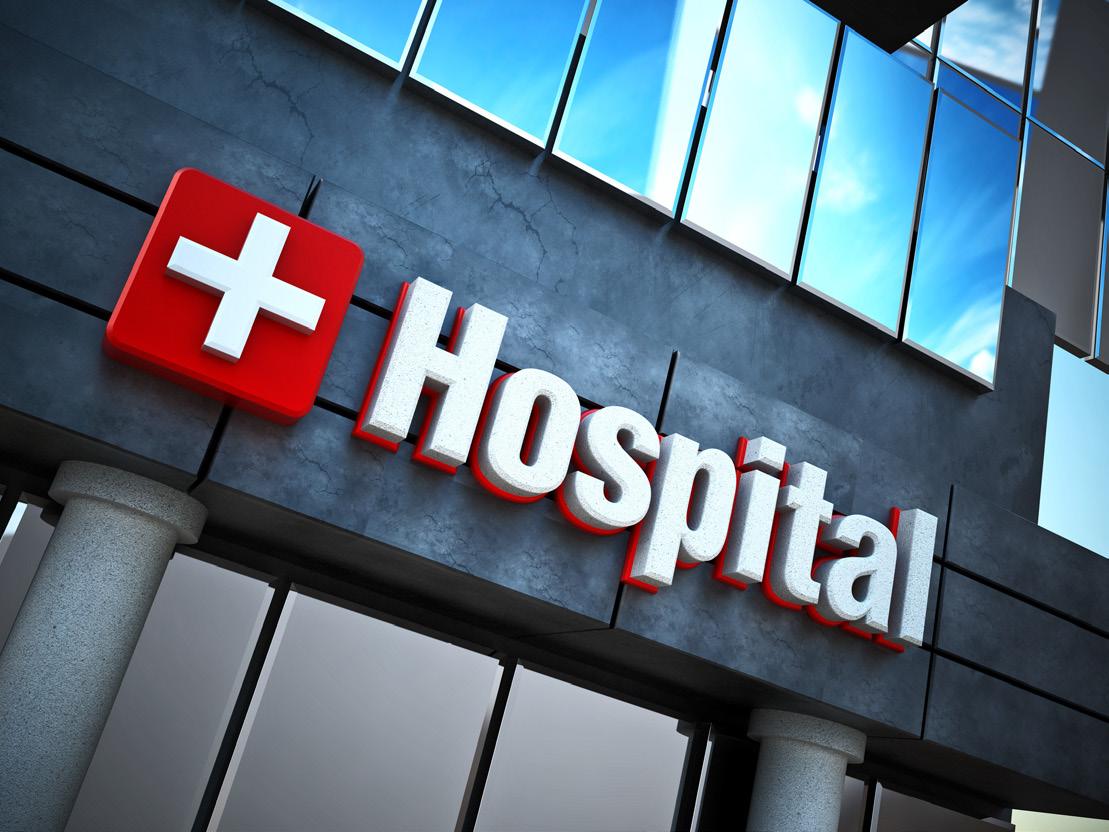
5 minute read
Medical Historic Vignette: Sir Godfrey Hounsfield and CT
by TEAM
RiChaRD h. DaFFneR, mD, FaCR
Wilhelm Konrad Roentgen, a German physicist, discovered x-rays in November 1895 and reported his findings to the Würtzburg Physical Medical Society in December of that year1. Less than two months later, the first medical x-rays of a broken forearm were obtained at Dartmouth University. Over the next eighty years refinements in the practice of radiology resulted in improved safety for patients and operators of x-ray equipment as well as a wide variety of newer applications and techniques in the field.
Advertisement
Radiographs (x-ray images) were (and still are) superb for diagnosing lung diseases as well as most fractures and bone lesions. However, when it came to studying the brain or the solid organs of the abdomen it was necessary to opacify those structures which could be opacified with either positive contrast or air to see if a disease process was displacing the normal organs. Physicians longed for the ability to see the body’s organs from the earliest days of radiologic practice. It would take the innovative thoughts of a British engineer, who like Roentgen was not a physician, to bring those desires to fruition.
Sir Godfrey Hounsfield (Fig. 1) was born August 28th, 1919, in Suttonon-Trent, in Nottinghamshire, England.
As a child he was fascinated with electrical gadgets and machinery that he found on the family farm. While attending school he excelled in physics and mathematics. In 1939 he joined the Royal Air Force volunteer reservist squadron 89, where he learned the basics of electronics and radar, that would prove critical to the allied victory in World War II. Following the war, in 1946 he attended Faraday House Electrical Engineering College in London. There, he combined practical experience with theoretical study. In 1949 he began work at EMI (Electric and Musical Industries, Ltd - the same company that made the Beatles famous), where he researched guided weapon systems and radar and became interested in computers. In 1958, he helped design the first commercially available all-transistor computer in Britain, the EMIDEK 1100.
In 1960, Hounsfield had an idea that one could determine what was inside a box by taking x-ray readings at all angles around the object. He began constructing a computer that could take input from those x-rays at various angles to create an image of the object in “slices”. This led him to propose what we now know as computed tomography (CT). Hounsfield was unaware of the work Allan Cormack (1924–1998), a South African physicist had done on the theoretical mathematics for such a device. Hounsfield built a prototype scanner and tested it, first on a preserved human brain (Fig. 2), then on a fresh cow brain from a local butcher shop, and then on himself. On October 1st, 1971, the first successful CT brain scan was performed in London on a patient with a cerebral cyst. In 1975 he built the first whole body scanner for EMI.
The principles of image formation with CT evolved from the work of Austrian mathematician
Johann Radon (1887–1956) (Fig. 3), who, in 1917, while working on gravitational fields, postulated that the image of a 3-dimensional object could be reconstructed from an infinite number of 2-dimensional projections of that object. His theories would have to wait until the computer age for implementation. In 1959, William Oldendorf (1925–1992) (Fig. 4), a neurologist at UCLA declared his goal was “to scan a head through a transmitted beam of x-rays, and to reconstruct the radiodensity patterns of a plane through the head” using Radon’s mathematical formulas. Hounsfield followed in their footsteps.
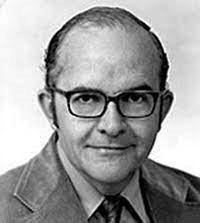
In diagnostic imaging there are four “classic radiographic densities –gas (air): black; fat: dark gray; water (soft tissue) gray; and metal (bone): white. The brain, heart, muscle, and abdominal organs all appear in various shades of gray, which are, for the most part, indistinguishable from one another. The principles of CT are identical to those of x-ray physics. Whenever an x-ray beam passes through an object, some of it is absorbed, some is scattered, and the rest passes through to form an image on a piece of film or a digital detector plate. The degree of absorption can be mathematically measured from the amount of radiation reaching the detector and is referred to as the absorption coefficient. Absorption coefficients vary for different tissues within the body. The human eye is limited in how many shades of gray it can discern. Computer technology, on the other hand, can measure the actual absorption coefficient and portray the differences in an unlimited number of shades of gray. In CT, absorption coefficients are measured in Hounsfield Units (HU), where water is the standard and is given the value of 0, bone being the most dense is 1000, and air, the least dense is -1000 (Table 1). This allows us to directly see the organs of the interior of the skull, thorax, and abdomen, whereas in the pre-CT era, we would opacify those structures we could opacify (GI tract, urinary tract, blood vessels, and ventricles of the brain) and look for impingement, distortion, or displacement
CT had an immediate impact on the practice of medicine, and the numbers of CT machines quickly proliferated throughout the world. CT immediately made a number of diagnostic imaging procedures obsolete, such as conventional
From Page 13 tomography, pneumoencephalography, lymphangiography, and Sweet’s eye locating intraocular foreign bodies. Furthermore, the technology of the CT machines themselves continued to evolve, becoming faster and more efficient in being able to portray body organs with greater accuracy. The computer-generated images used in the 1977 movie Star Wars prompted Dr. Michael Vannier, a radiologist at the Mallinckrodt Institute of Radiology in St. Louis, to inquire of George Lucas if his computer algorithms could be adapted to CT imaging. Lucas shared the algorithms with Dr. Vannier, who used them to develop multiplanar (sagittal and coronal) and 3-D reconstructions which are now commonplace components of every CT scan.
Other applications include combining CT with PET (Positron Emission Tomography) scans for more accurate localization of tumors and infections. PET scanning uses cyclotron generated isotopes that emit positrons. These isotopes show the increased use of glucose by tumors and infections. In addition, CT is used for image- guided interventions such as performing precise tissue biopsies or even in the placement of intraosseous orthopedic screws2. Finally, the virtual autopsy (“virtopsy”) was developed in Europe as an alternative to the traditional postmortem examination. The virtual autopsy preserves the body by performing a head-to-toe nondestructive data-gathering study. It is particularly useful for those areas that are difficult to assess such as the upper spine or on bodies that have undergone advanced decomposition.
By injecting intravenous contrast material, the cardiovascular system can be visualized. Numerous studies have shown that the virtual autopsy has an accuracy of approximately 99% when compared to the traditional autopsy3
Godfrey Hounsfield received many honors, including Commander in the Order of the British Empire (1976), and the Nobel Prize in Physiology or Medicine, which he shared with Allan Cormack (1924–1998) (Fig. 5) in 1979. He was knighted by Queen Elizabeth II in 1981. He retired from EMI in 1986 and accepted a consulting professorship at the Mallinckrodt Institute of Radiology, where he continued his work on applications of CT scanning as well as improvement in the efficiency of CT machines. After returning to England, he used the prize money from his Nobel to build a personal laboratory in his home. Hounsfield died at KingstonUpon-Thames, Surrey, in 2004 at the age of 84.
Wilhelm Konrad Roentgen and Godfrey Hounsfield were two nonphysicians whose discoveries and ingenuity have radically changed not only how we diagnose diseases but also how we treat patients. In 1895, Roentgen’s discovery provided us with a means to examine a patient on the inside. In 1971, Hounsfield’s ingenuity gave us an entirely different and improved means of making that examination. The CT imaging algorithms that Hounsfield developed facilitated and formed the basis of magnetic resonance (MR) imaging that we use today.
References
1. Daffner RH. Roentgen and the discovery that changed medicine. ACMS Bulletin Nov 2020, pp 331–333.
2. Sciulli RL, Daffner RH, Altman DT, Altman GT, Sewecke JJ. CT-guided Iliosacral screw placement: technique and clinical experience. Am J Roentgenol 2007; W 188:181-192.
3. Flach PM, Thali MJ, Germerott T. Review. Times have changed! forensic radiology - a new challenge for radiology and forensic pathology. Am J Roentgenol 2014; 202; W 325–334.
Dr. Daffner is a retired radiologist, who practiced at Allegheny General Hospital for over 30 years. He is Emeritus Clinical Professor of Radiology at Temple University School of Medicine. He is also an amateur historian.
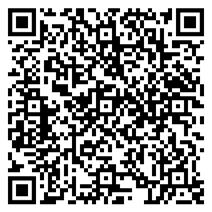
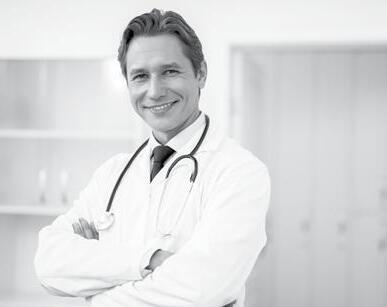