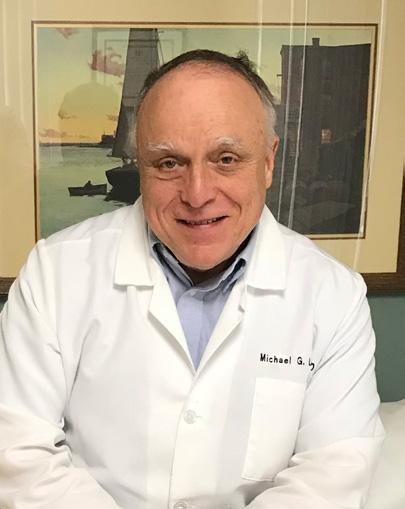
14 minute read
March Is Trisomy Awareness Month Down Syndrome: It’s Mitochondrial and It’s Treatable
by TEAM
miChael G. lamB mD
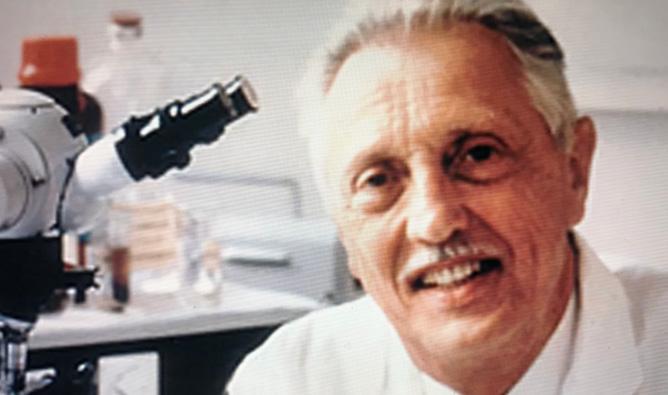
Advertisement
“Mitochondrial dysfunction plays a primary role in the neurodevelopmental anomalies and neurodegeneration of Down syndrome subjects.” That statement came from Drs. Mollo and Nitti’s group in the Department of Molecular Medicine at the University of Naples in the June 2019 issue of Frontiers in Genetics. Two years earlier, Valenti et al noted that “dysfunctional mitochondria and mitochondrial dependent activation of intracellular stress cascades are critical initiating events in many neurodevelopmental and neurodegenerative diseases including Down syndrome.” Helguera et al at the University of California in 2013 stressed that “mitochondrial dysfunction and oxidative stress are common features of Down syndrome”. More recently a 2021 study out of Spain (M. Pilar Bayone-Bafalvy et al) had the title “Down Syndrome is an Oxidative Phosphorylation Disorder”. In this paper they state that “Down syndrome is the most common genomic disorder of intellectual disability and is caused by trisomy of the 21st chromosome. Several genes in this chromosome repress mitochondrial biogenesis”. These are just a few of the many published articles linking Down syndrome to defects in oxidative phosphorylation secondary to mitochondrial dysfunction. Decades ago, famed geneticist and co-discoverer of trisomy 21, Jerome Lejeune postulated that Down syndrome would turn out to be a metabolic disease. Some mitochondrial metabolic disorders are treatable, and others are potentially treatable. Down syndrome has thus at the very least become a potentially treatable metabolic condition.
One would think that this type of discovery would be heralded and make medical headlines. It did not and it is not clear why not. In fact, it can safely be stated that most pediatricians, internists, obstetricians, and family medicine specialists are unfamiliar with this literature even though it is extensive. The lack of familiarity with this research even includes physicians working in “Down Syndrome Clinics”. It’s not because this data is relatively new, because this research goes back to more than 20 years ago with work done by S.H. Kim that demonstrated decreased levels of mitochondrial respiratory enzymes in Down syndrome fetuses and adults. Since then, the increase in studies involving mitochondrial dysfunction, mitochondrial dysmorphology, and even mitochondrial therapies related to Down syndrome has been exponential and yet physician awareness of these reports has been minimal. Most of these reports have been in genetics, pharmacology, molecular medicine, biochemistry, and neuroscience journals and that may be part of the problem. In the past 20 years, multiple review articles on these topics have been published but none of them are in the 4 major pediatric journals: The Journal of Pediatrics, JAMA Pediatrics, Lancet Child and Health, and Pediatrics. One wonders why a topic with this much biochemical and genetic research and involving a common disabling pediatric condition has apparently been ignored by the major pediatric journals.
A lot of the initial work regarding the relationship between Down syndrome and mitochondrial dysfunction was done in mice with trisomy 16 (a Down syndrome animal model). These studies uniformly showed mitochondrial enzyme deficiencies, a decreased amount of mitochondrial DNA, defects in oxidative phosphorylation, reduced energy production (i.e. high energy phosphate compounds), increased oxidative stress, abnormal mitochondrial cristae, a reduction in mitochondrial membrane potential, and abnormal cytoarchitectural ordering of mitochondria. Studies in human Down syndrome cells cultured in vitro have confirmed the results observed in the trisomy 16 mouse models. These abnormalities were exhibited at various ages (fetus, child, and adult) in several different Down syndrome cell types including amniocytes, neuronal stem cells, fibroblasts, brain cells, cardiac myocytes, blood monocytes, blood lymphocytes and skeletal muscle cells. The study of cultured neuronal stem cells by Mollo and Esposito et al showed mitochondrial dysfunction as early as seven days and a marked tendency to form glial cells rather than functional neuronal cells. The study involving skeletal muscle was an in vivo study using phosphorus magnetic resonance spectroscopy. The cardiac myocyte studies suggested that more severe mitochondrial dysfunction was associated with the presence of congenital heart disease. The fact that mitochondrial abnormalities were evident in fetal, childhood, and adult cells of various types points to the mitochondrial abnormalities being congenital and in all cell types.
The in vivo study demonstrates that this problem with mitochondrial function is a significant issue in live patients and not just found in cultured cells.
The study of mitochondrial disease in Down syndrome is not confined simply to cell morphology, biochemistry. and cell physiology. Detailed genetic studies have also been a part of this research. As far back as 1995, Abruzova published on the role of mitochondrial DNA in trisomy 21. Three years later she presented a paper at the 6th world Congress on Down syndrome in Madrid, dealing with “why is it necessary to study the role of the mitochondrial genome in Trisomy 21 pathogenesis”. Subsequent studies showed that multiple genes affecting mitochondria are located on chromosome 21. A decreased level of ATPase (a mitochondrial DNA product) has also been documented in Down syndrome patients. So, there have been both nuclear and mitochondrial genes identified as abnormal. This data suggests that oocyte mitochondrial dysfunction is involved in the pathogenesis of the chromosomal non-disjunction in the syndrome. Mitochondrial dysfunction has also been implicated in heterotaxy and defective migration of embryonic cells in Down syndrome. This is because normal ATPase generation is required for those processes. In this regard, it’s notable that trisomy 18, trisomy 13, and Klinefelter syndrome patients also have been reported as having abnormal mitochondrial function, cellular oxidative stress and or anomalies of the mitochondrial genome. This is all consistent with oocyte mitochondrial dysfunction being a causative factor in several conditions in which nondisjunction is present. Mitochondrial function generally worsens with advancing age. This helps to explain why non-disjunction is more common in older mothers. Mitochondrial dysfunction has also been implicated in autism spectrum disorder, fragile X syndrome and fetal alcohol spectrum disorders (alcohol is a mitochondrial toxin).
Mitochondrial medicine and the understanding of normal and pathologic mitochondrial anatomy and physiology is at the cutting edge of modern medicine. It will only become more important in the future. Of significance here is the fact that at least some mitochondrial disorders are treatable with agents such as ubiquinol, carnitine, and alpha lipoic acid. Down syndrome now presents the option of being treatable. In the January 2023 issue of “Mitochondrion”, Ganguly and Kadam wrote that “a number of pharmacologically active natural compounds such as polyphenols, antioxidants, and flavonoids show convincing outcomes for reversal of the dysfunctional mitochondrial network and oxidative metabolism and improvement of intellectual skills in mouse models of Down syndrome and humans with Down syndrome”. In another 2023 article, Tan et al commented that “it is prudent that improving Down syndrome pathophysiologic conditions or quality of life may be feasible by targeting something as simple as mitochondrial biogenesis and function”. Fatty acid supplements seem to be beneficial in the mouse model of Down syndrome. One case study showed that fish oil rich in omega 3 fatty acids reversed mitochondrial dysfunction and was well tolerated in a Down syndrome child. Another group has reported modest improvement in coordination and muscle function in Down syndrome children treated with ubiquinol (Co-enzyme Q). Agents that have caused a dramatic improvement and even reversal of mitochondrial network abnormalities and abnormal bioenergetics include metformin, pioglitazone, reservatrol and EGCG (epigallocatochin 3 gallate). Those studies were done with in vitro cultures of Down syndrome cells. Metformin is an especially exciting therapeutic option since it has a long history of use, multiple other indications, an acceptable safety profile, crosses the blood brain barrier, and has been used in children. The in vitro benefits of metformin are impressive. In their paper on metformin treatment, Izzo et al concluded that “metformin represents a promising strategy to counteract mitochondrial dysfunction in Down
Continued on Page 18 syndrome”. Currently, the University of California is conducting a metformin study to treat fragile X syndrome and its’ associated obesity. There are also multiple trials using metformin regarding longevity and aging. Presently, however, there are no therapeutic trials available in the U.S.A. involving either metformin or pioglitazone in Down syndrome. A recent internet search showed that there are also no American controlled trials using flavanoids, fatty acids, polyphenols, or ubiquinol. This is difficult to comprehend given the fact that for over a decade there has been a lot of convincing evidence that Down syndrome is a mitochondrial disease that in vitro responds well to these agents.
An internet survey of several Down syndrome clinics in the Eastern United States at major medical centers showed no mention of mitochondrial dysfunction or mitochondrial treatment on their web sites. Most of these clinics did offer the option of genetic counselling and prenatal diagnosis. Prenatal diagnosis affords the option of abortion as a “treatment”. Early stimulation programs and learning therapies were generally offered by all the clinics. In the absence of controlled trials, should Down syndrome patients be afforded the option of “off label” structured empiric treatment with metformin? This might be particularly advantageous because of the safety profile plus the added benefit of metformin in obese pre-diabetic patients (a common problem with Down syndrome). Given the potential benefits, it would seem justified that metformin be offered as an empiric option. The possibility of offering a structured trial of the other agents with in vitro effectiveness should also be considered especially in regard to supplements of ubiquinol and fatty acids which have minimal side effects. Additionally Down syndrome patients should prudently avoid known mitochondrial toxins including alcohol, valproic acid, chloramphemicol, gentamycin, and tobramycin. It is possible that other antibiotics could also be hazardous in Down syndrome given the fact the mitochondria likely evolved from primitive bacteria. The potential for Down syndrome to be a treatable medical condition should have been received with enthusiasm and excitement. This has not been the case. Perhaps this is due to the fact that major pediatric journals have neglected this topic. This may also be due in part to certain physician attitudes regarding Down syndrome and people with cognitive dysfunction. Studies have documented that a significant percentage (as much as 24%) of practicing physicians and resident physicians are “not comfortable” caring for people with Down syndrome. It is hoped that this article may help a bit in making morephysicians aware of this literature and the available treatment options.
Mitochondrial Disease In Down Syndrome References
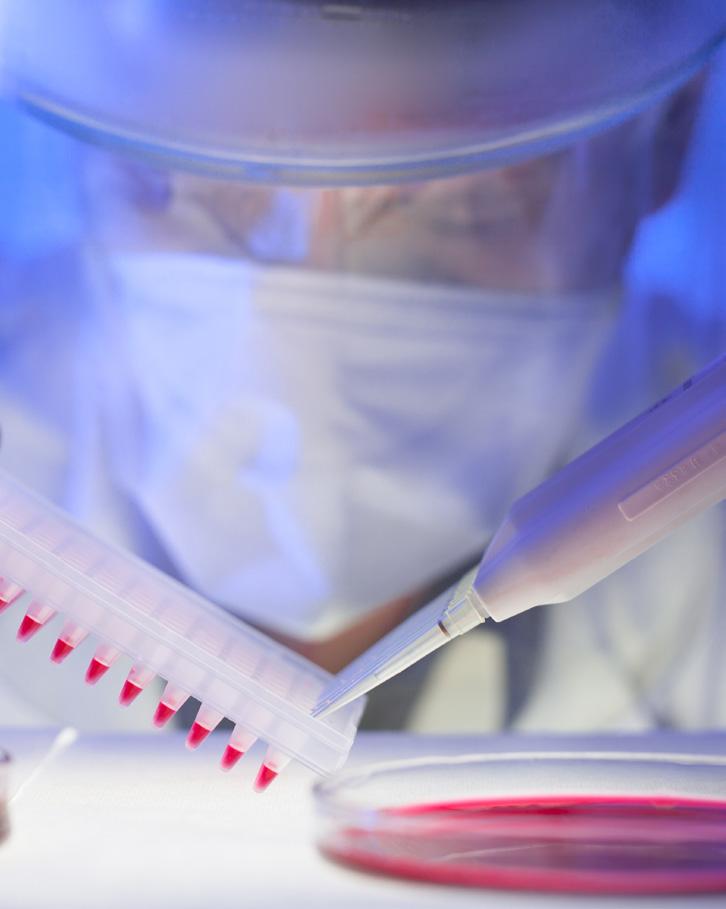
Craven et al, 2017. “Recent Advances in Mitochondrial Disease”, The Annual Review of Genomics and Human Genetics. 18:257-275.
Podolak et al, 2022. “The Role of Mitochondria in Human Fertility and Early Embryo Development: What Can We Learn from Clinical Application of Assessing and Improving Mitochondrial DNA?, Cells 2022,11, 797.
Wilding et al, 2009. “Mitochondria and Human Preimplantation Embryo Development”; Reproduction 137:619-624.
Schuchmann et al 1995. “Altered Ca Signaling and Mitochondrial Deficiencies in Hippocampal Neurons of Trisomy 16 Mice: A Model of Down’s Syndrome”, The Journal of Neuroscience September 15, 1998. 18(18):7216-7231.
Shukkur et al,2006.
“Mitochondrial Dysfunction and tau Hyperphosphorylation in Ts1Cje, A Mouse Model for Down’s Syndrome”, Human Molecular Genetics, vol 15, no.18:2752-2762.
Valenti et al, 2017. “Inhibition of Drp1 Mediated Mitochondrial Fission Improves Mitochondrial Dynamics and Bioenergetics Stimulating Neurogenesis in Hippocampal Progenitor Cells From a Down’s Syndrome Mouse Model”, Biochim Biophys Acta Mol Basis Dis.2017 Dec 1863(12):3117-3127.
Valenti et al, 2021. “Impaired Brain Mitochondrial Bioenergetics in the Ts65Dn Mouse Model of Down Syndrome is Restored by Neonatal Treatment with the Polyphenol 7,8-Dihydroxy flavone”, Antioxidants, 2021 Dec 28; 11(1):62.
Bayona-Bafaluy et al, 2021. “Down Syndrome is an Oxidative Phosphorylation Disorder”, Redox Biology 41(2021) 101871.
Dierssen et al, 2020. “Down Syndrome is a Metabolic Disease: Altered Insulin Signaling Mediates Peripheral and Brain Dysfunctions”, Frontiers in Neuroscience July 2020, vol 14, article 670.
Helguera et al, 2013. “Adaptive Downregulation of Mitochondrial Function in Down Syndrome”, Cell Metabolism 2013 January 8:17(1):132-140.
Pecze et al, 2020. “Meta-Analysis of Metabolites Involved in Bioenergetic Pathways Reveals a Pseudohypoxic State in Down Syndrome”, Mol Med. 2020 Nov 9;26(1):102.
Nowinski et al, 2020. “Mitochondrial Fatty Acid Synthesis Coordinates Oxidative Metabolism in Mammalian Mitochondria”, Elife 2020 Aug 17;9:e58041.
Anderson et al, 2021. “Trisomy 21 Results in Modest Impacts on Mitochondrial Function and Central Carbon Metabolism”, Free Radic Bio. Med. 2021 Aug. 20;172:201-212.
Busciglio et al, 2002. “Altered Metabolism of the Amyloid Beta Precursor Protein is Associated With Mitochondrial Dysfunction in Down’s Syndrome”, Neuron Feb 28;33(5):677-88.
D’Acunzo et al, 2021. “Mitovesicles are a Novel Population of Extracellular Vesicles of Mitochondrial Origin in Down Syndrome”, Sci Adv. 2021 Feb 12;7(7):eabe5085.
Zamponi et al, 2019. “The Shape of Mitochondrial Dysfunction in Down Syndrome”, Dev. Neurobiol. 2019 Jul;79(7):613-621.
Piccoli et al, 2012. “Chronic ProOxidative State and Mitochondrial
Dysfunctions are More Pronounced in Fibroblasts from Down Syndrome Foeti with Congenital Heart Defects”, Hum Mol Genet. 2013 Mar 15;22(6):1218-32.
Conti et al, 2001, Altered Expression of Mitochondrial and Extracellular Matrix Genes in the Heart of Human Fetuses with Chromosome 21 Trisomy, BMC Genomics 2007 Aug 7;8:26
Hefti et al, 2014. “Analysis of mtDNA, miR-155 and BACH1 Expression in Hearts from Donors With and Without Down Syndrome”, Mitochondrial DNA A DNA Mapp Seq Anal 2016; 27(2):896-903.
Hefti et al, 2017. “Analysis of Heteroplasmic Variants in the Cardiac Mitochondrial Genome of Individuals with Down Syndrome”, Human Mutat. 2017 Jan; 38(1):48-54.
Venegas-Zamora et al, 2022. “New Molecular and Organelle Alterations Linked to Down Syndrome Heart Disease”, Front Genet. 2022 Jan 18;12:792231.
Phillips et al, 2013. “Defective Mitochondrial Function in Vivo in Skeletal Muscle in Adults With Down’s Syndrome: a 31P-MRS Study”, Plos One, 2013 Dec 31;8(12).
Roat et al, 2007. “Mitochondrial Alterations and Tendency to Apoptosis in Peripheral Blood Cells from Children with Down Syndrome”, FEBS Lett, 2007 Feb 6;581(3):521-5.
Perluigi et al, 2012. “Oxidative Stress and Down Syndrome: A Route Toward Alzheimer-Like Dementia”, Curr Gerontol Geriatr Res. 2012;2012: 724904.
Valenti et al, 2014. “Mitochondrial Dysfunction as a Central Actor in Intellectual Disability-Related Diseases: An Overview of Down Syndrome, Autism, Fragile X and Rett Syndrome” Neuroscience Biobehav Rev. 2014 Oct;46 Pt 2:202-17.
Kim et al, 2000. “Decreased Level s of Complex III Core Protein 1 and Complex V beta Chain in Brains from Patients with Alzheimer’s Disease and Down Syndrome”, Cell Mol Life Sci. 2000 Nov; 57(12):1810-6.
Kim et al, 2001. “Decreased Protein Levels of Complex I 30-kDa Subunit in Fetal Down Syndrome Brains”, Neuro Transm Suppl. 2001;(61):109-16.
Kim et al, 2001. “The Reduction of NADH Ubiquinone Oxidoreductase 24- and 75-kDa Subunits in Brains of Patients with Down syndrome and Alzheimer’s Disease”, Life Sci. 2001 May 4 68(24):2741-50.
Mollo et al, 2021. “Human Trisomic iPSCs from Down Syndrome Fibroblasts Manifest Mitochondrial Alterations Early During Neuronal Differentiation”, Biology 2021, 10, 609.
Arbuzova, Svetlana, 1998, “Why it is Necessary to Study the Role of the Mitochondrial Genome in Trisomy 21 Pathogenesis”, Down Syndrome Research and Practice, 5(3), 126-130
Schon et al, 2000. “Chromosomal Non-disjunction in Human Oocytes: is there a Mitochondrial Connection?”, Human Reproduction vol. 15 (suppl.2) pp. 160-172.
Lee et al, 2003. “Expression of the Mitochondrial ATPase6 Gene and Tfam in Down syndrome”, Mol Cells. 2003 April 30;15(2):181-5.
Helguera et al, 2005. “ETS2 Promotes the Activation of a Mitochondrial Death Pathway in Down Syndrome Neurons”, J. Neurosci. 2005 Mar 2;25(9):2295-303.
Parra et al, 2018. “Down Syndrome Critical Region 1 Gene, Rcan1, Helps Maintain a More fused Mitochondrial Network”, Circ. Res. 2018 Mar 16;122(6):e20-e33.
Burkhalter et al, 2019. “Imbalanced Mitochondrial Function Provokes Heterotaxy Via Aberrant Ciliogenesis”, J Clin Invest. 2019 Jul 1; 129(7):28412855.
Buczynska et al, 2022. “Future Perspectives in Oxidative Stress in Trisomy 13 and 18 Evaluation”, J. of Clinical Medicine 2022, 11,1787.
Oikawa et al, 2002. “The Specific Mitochondrial DNA Polymorphism Found in Klinefelter’s Syndrome”, Science Direct Vol 297, issue 2, 20 September 2002, pp. 341-345.
Siddiqui et al, 2016. “Mitochondrial Dysfunction in Autism Spectrum Disorders”, Autism Open Access.; 6(5): doi:10.4172/2165-7890.
Parikh et al, 2009. “A Modern Approach to the Treatment of Mitochondrial Disease”, Curr Treat Options Neurol. 2009 November; 11(6):414-430.
Valero, Teresa, 2014. “Mitochondrial Biogenesis: Pharmacological Approaches”, Curr Pharm Res 2014;20(35):5507-9.
Franceschi et al, 2018. “Accelerated Bio-cognitive Aging in Down Syndrome: State of the Art and Possible Deceleration Strategies”, Aging Cell. 2019;18:e12903.
Mollo et al, 2020. “Targeting Mitochondrial Network Architecture in Down Syndrome and Aging”, Int. J. of Molecular Sciences 2020,21, 3134.
Izzo et al, 2018. “Mitochondrial Dysfunction in Down Syndrome: Molecular Mechanisms and Therapeutic Targets”, Molecular Medicine (2018) 24(1):2.
Ganguly et al, 2023. “Therapeutics for Mitochondrial Dysfunction-linked Diseases in Down Syndrome”, Mitochondrion 2023 Jan;68:25-43.
Valenti et al, 2018. “Mitochondria as Pharmacological Targets in Down Syndrome”, Science Direct vol 114, Jan 2018, pp. 69-83.
Ganguly, Bani, 2022, “Disarrayed Mitochondrial Function and Pathobiology in Down Syndrome and Targeted Therapeutics”, Science Direct 2022, pp. 219-243.
Tan et al, 2023. “Mitochondrial Dysfunction in Down Syndrome: From Pathology to Therapy”, Neuroscience, vol 511, 10 February 2023, pp. 1-12.
Vacca et al, 2015. “Green Tea, EGCG, plus Fish Oil Omega-3 Dietary Supplements Rescue Mitochondrial Dysfunctions and are Safe in a Down Syndrome Child”, Clinical Nutrition volume 34, issue 4, August 2015, pp. 783-784.
Martinezcue et al, 2022. “Fatty Acids: A Safe Tool for Improving Neurodevelopmental Alterations in Down Syndrome?” Nutrients 2022 Jul 13;14(14):2880.
Valenti et al, 2016.The Polyphenols Resveratrol and Epigallocatechin-3gallate Restore the Severe Impairment in Hippocampal Progenitor Cells from a Down Syndrome Mouse Model”, Biochim Biophys Acta. 2016 Jun;1862(6):1093-104.
Valenti et al, 2013. “Epigallocatechin3-gallate Prevents Oxidative Phosphorylation Deficit and Promotes Mitochondrial Biogenesis in Human Cells from Subjects with Down’s Syndrome”, Biochim Biophys Acta 2013 Apr, 1832(4):542-552.
Mollo et al, 2019. “Pioglitazone Improves Mitochondrial Organization and Bioenergetics in Down Syndrome Cells”, Frontiers in Medicine June 2019, vol. 10, article 606.
Izzo et al, 2017. “Metformin Restores the Mitochondrial Network and Reverses Mitochondrial Dysfunction in Down Syndrome Cells”, Human Mol. Genet. 2017, vol 26, no.6:1056-69.
Lagouge et al, 2006. “Resveratrol Improves Mitochondrial Function and Protects Against Metabolic Disease by Activating SIRT1 and PGC-1”, Cell 127, 1109-1122, Dec 15 2006.
Neergheen et al, 2017. “Coenzyme Q10 in the Treatment of Mitochondrial Disease”, Journal of Inborn Errors of Metabolism 2017, vol 5:1-8.
Quinzii et al, 2019. “Coenzyme Q and Mitochondrial Disease”, https:// www.ncbi.nim.nih.gov/ pmc/articles/ PMC3097389/.
Lagos Tara et al 2022, I Am Not The Doctor For You: Physicians Attitudes About Caring for People With Disabilities, Disability and Health, vol. 41, no. 10
Pace Jill et al 2011, Understanding Physician’s Attitudes Toward People With Down Syndrome, Am J Med Genet Jun;155A(6): 1258-1263
Hannah F. et al 2023, Education Research: Predictors of Resident Physician Comfort With Individuals With Intellectual and Developmental Disabilities, Neurology Education 2-e 2000045 doi: 10.1212
The American College of Surgeons – Southwestern Pennsylvania Chapter is excited to host its annual “Most Interesting Case Presentations” event. This event provides surgical residents the opportunity to present their most interesting cases for review and discussion. Presentations are judged by a panel of surgeons. Cash prizes are awarded to the three most interesting cases.
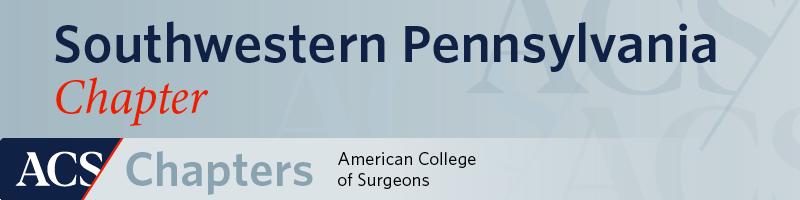
AGENDA
6:00 PM
EXECUTIVE BOARD MEETING (Invite Only)
6:30 PM – 7:00 PM
COCKTAILS
7:00 PM – 8:00 PM
PRESENTATIONS & DINNER
Awards
1ST PLACE - $500
2ND PLACE - $300
3RD PLACE - $200
OPEN TO ALL GENERAL SURGERY RESIDENTS AND SURGICAL SPECIALTY RESIDENTS.
SAVE THE DATE!
Registration coming soon.
Featured Grant Recipient
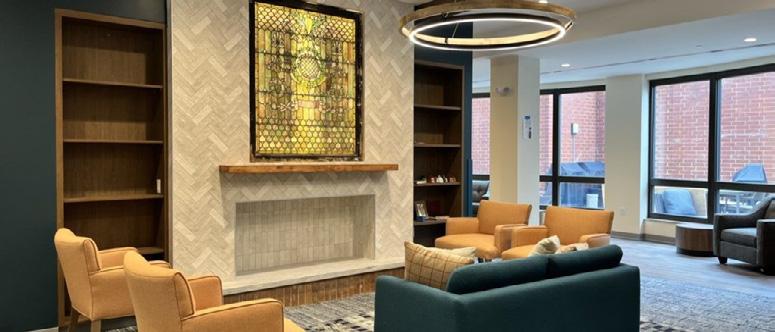
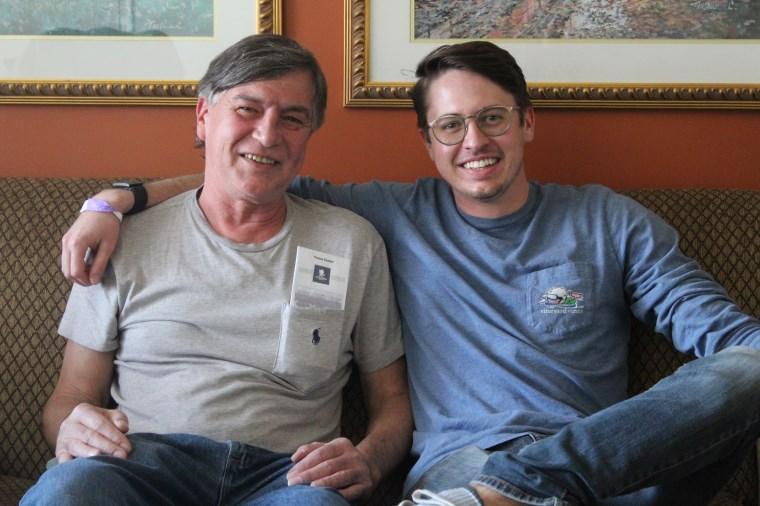
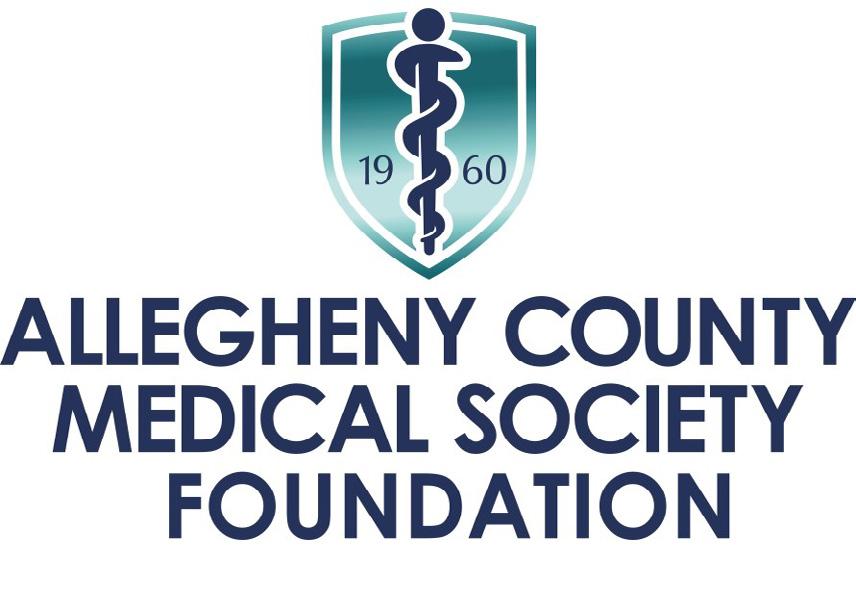
Family House
Family House opened on November 13, 1983 with a mission to provide a safe, comforting, and affordable “home away from home ” for patients and their families who travel to Pittsburgh hospitals for expert medical care. To fulfill its mission, Family House offers a room rate at a fraction of what one would pay for a hotel in the Oakland or Shadyside neighborhoods. The peaceful, home -like atmosphere at Family House has been intentionally designed, with complimentary services such as weekday breakfast, transportation, stocked food pantries, and more.
Family House is an essential partner in the region ’s healthcare delivery system, serving those who travel long distances, as well as Pennsylvania residents who must travel across the state. Family House’s lodging facility and the services, carried out by its professional staff and volunteer corps, are an essential part of the healthcare journey.
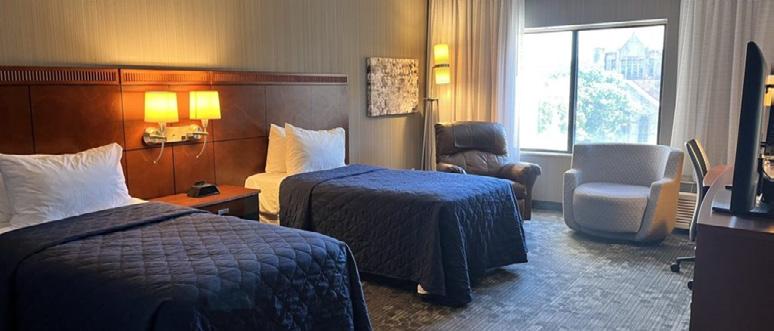
Everything Family House provides helps stretch financial resources for families in the midst of a medical situation. In FY21, Family House ’s room rates remained at deeply discounted levels, with 25% of room operating costs subsidized through philanthropic contributions. Family House met the lodging needs of 13,753 patients and caregivers over 8,235 reservations and 25,884 nights of service.
This impact is owed to each volunteer who prepares a home -cooked meal, every donor who mailed in a handwritten check, the corporations who invest in our annual fundraising efforts, and t the foundations who partner with us in making Pittsburgh hospitable for all.
To learn more about Family House visit: familyhouse.org
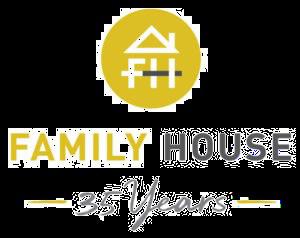
Donate to the ACMS Foundation to help support annual grant giving.
To learn more about the ACMS Foundation visit: acms.org/acmsfoundation