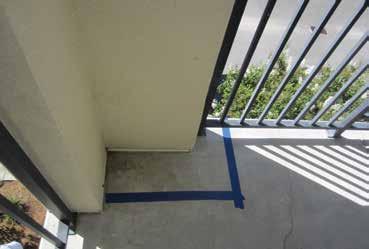
23 minute read
STRUCTURAL DESIGN
design issues for structural engineers The author’s company, a forensic engineering and architecture firm, has investigated hundreds of low-slope roof and exterior deck applications with water stains, ponding, framing damage, and structural collapse. The first article, Part 1: ¼ in 12 Design Slope and Water Drainage (STRUCTURE, August 2017), examined two building code parameters that contribute to lowslope roof and deck serviceability issues. This article identifies design and construction practices that limit or prevent free drainage. Potential solutions are presented to mitigate ponding that contributes to serviceability issues and structural framing damage. The goal is to raise awareness in the construction industry of typical practices that may cause harm to structural members and the building envelope. may create a negative slope or a “bowl” condition at the low end that limits or prevents free drainage. The condition is exacerbated for materials susceptible to creep deflection, such as wood. Beam members designed and installed to the ¼-inch per foot slope should be considered a susceptible bay. Readers are encouraged to visit the first article for additional information and potential solutions. Field observations have identified common design practices that contribute to serviceability issues. These design blunders limit or prevent free drainage and result in unsatisfactory building envelope performance. Additionally, the absence of specific design details and reference to a “best practice” often result in typical construction practices that may meet the general intent of the building code but limit free drainage.
Background Design Blunders
The 2015 International Building Code (IBC) establishes minimum parameters for building design and construction. A member or system that satisfies applicable individual code parameters may
When design professionals specify framing members to minimum building code parameters alone, it is possible for the constructed roof to have inservice low-slope issues related to ponding or drainage of the system. Five common design blunders Low-Slope Roof and Deck Design Considerations that contribute to low-slope issues and potential solutions are summarized below.
Part 2: Mitigate Ponding and Water Intrusion
By Scott D. Coffman, P.E., SECB
Scott Coffman is a Forensic Engineer with Construction Science and Engineering, Inc. in Westminster, SC. He can be reached at scottcoffman@ constructionscience.org. create a less than ideal condition when multiple minimum code parameters are combined. For Intersecting Planes example, the combination of the ¼-inch per foot Building offsets are common and create interdesign slope and a maximum permitted deflec- secting planes that contribute to drainage issues tion ratio can create a condition that inhibits free for low-slope applications. Design professionals drainage. The IBC, however, does recognize this frequently specify the minimum code-permitted potential condition in Table 1604.3 footnote “e” slope with little, if any, consideration of the resuland instructs a building designer to investigate tant valley slope created by the intersecting planes applications with insufficient slope or camber (Figure 1). Ponding water is commonly observed for ponding. at valley intersections for low-slope roof and deck Design professionals, contractors, and perhaps (balcony) applications. code officials have come to believe a roof or exterior deck surface designed to the ¼-inch per foot slope is satisfactory because it meets building code intent. However, member deflection ROOF DRAIN creates an average slope that limits free drainage and contributes to ponding toward the low end. The “average slope” is the slope of a line from the low-end support to the point of maximum deflec- PLAN VIEW tion for a member. Members optimized to a code-permitted deflection ratio further reduce the average slope and Figure 1. Common minimum roof slope plan with valley.
SLOPE
SLOPE LIVING ROOM
BALCONY
SLOPE
PLAN VIEW
Figure 2. The design slope is reduced at sloped plane intersections. Figure 3. Integrated balcony column without drainage provisions.
continued on next page
8'
The diagonal distance between two fixed elevation points is less than the design slope. This principle can be illustrated by two sloped planes that intersect at a right angle (Figure 2). The eight-foot wide balcony with a specified ¼ in 12 slope has a twoinch elevation drop from the wall to the free drainage edge. The diagonal distance denoted in red has the same two-inch elevation change. However, the elevation change occurs over a distance of approximately eleven feet four inches, creating a slope less than the ¼ in 12 minimum slope. The 2010 edition of the Minimum Design Loads for Buildings and Other Structures (ASCE 7), published by the American Society of Civil Engineers (ASCE) and referenced by IBC, states in part “surfaces with a slope of at least ¼-inch per foot toward points of free drainage need not be considered a susceptible bay.” Therefore, roof and balcony surface areas designed to a ¼ in 12 slope that intentionally direct water to a valley should be considered a susceptible bay. A potential solution is to assign the ¼ in 12 slope to the valley and calculate the associated roof or balcony slope to be shown on the construction documents.
Integrated Columns
Building codes and accepted design practice incorporate “crickets” to divert water for effective drainage. Balcony support columns present conditions that are rarely detailed within the construction documents. Design professionals routinely design integrated exterior balcony columns that serve as “dams” that inhibit water from flowing toward points of free drainage (Figure 3). Columns are frequently located at the balcony perimeter and contain interior edges or corners. Water becomes trapped at the interior edges and often contributes to damage. Design professionals should provide clear details that divert or allow free drainage at these locations throughout the life of the building.
Sloped Concrete Surface
Many design professionals specify horizontal framing members with a sloped topping surface for drainage (Figure 4). The topping surface is typically a lightweight concrete product installed in a semi-fluid state. Specifications for a “stiff” slump test or to install with a stipulated slope are difficult at best, rarely achieved, and often result in a constructed level surface. Water percolates the permeable topping surface to the horizontal plane created by the support members. Free drainage rarely occurs since the support member is level or deflected vertically downward, allowing water to pond. Forensic investigations often encounter damage to support members when water finds a breach in the protective membrane between the topping surface and structural framing. Structural members should be designed and oriented with a positive slope toward points of free drainage for water discharge. The topping surface should conform to the structural member slope to maintain positive drainage. Water that permeates the topping surface encounters the sloped surface and is directed toward the desired free drainage location.
SLOPE
Δ 1
Δ 2
NOTCH DECK FOR FLASHING THICKNESS
DROPPED FASCIA TO MAINTAIN SLOPE OF DECKING

Figure 6. Fascia detail to maintain slope to free drainage edge. Figure 7. Component thickness prevents free drainage.
Wood Framing Members
The use of ripped, solid sawn framing members is a common design and construction practice to achieve a desired slope. Lumber grade marks are assigned in accordance with criteria outlined in the code referenced by the American Softwood Lumber Standard (PS 20). The standard specifically states that the remanufacture (ripping) of a graded or grademarked wood member negates the mark and associated design values of the original product. The “ripping” of lumber members can be eliminated by modifying the framing detail. One option is to install each end of the lumber member at two distinct elevations to achieve the desired slope. A ceiling joist or furring may be required to obtain a “flat” ceiling. A second option is to specify a truss with the desired top chord slope for drainage and horizontal bottom chord for ceiling attachment. Another common framing technique orients the framing member perpendicular to the free drainage slope direction. Forensic investigation of this condition typically finds water accumulation toward the center as the member deflects downward. Framing members should be oriented and installed to promote water flow toward points of free drainage.
Differential Deflection
A system of members with the same span are anticipated to deflect a similar amount. Adjacent members with different spans, however, deflect a different amount; the longer span member deflects more, relative to a shorter span member, and creates a “bowl” that retains water. The Truss Plate Institute (TPI) recognized this phenomenon and identified differential deflection as a design parameter for metal plate connected wood trusses. TPI Section 2.3.2.4 (g) (4) specifically requires the building designer to specify differential deflection design limits. Differential deflections, however, are not limited to wood trusses and this practice should be adopted to other building components. The design professional should consider material properties of the cover, framing, and ceiling when evaluating an acceptable limit to evaluate differential deflections. A similar condition exists for structural members installed parallel to a wall supported by a foundation. The design intent is for the wall to be a free drainage location; however, the wall is “rigid” and does not displace downward under load (Figure 5, page 31). The structural member adjacent to the wall deflects downward creating a “bowl.” For lowslope members adjacent to a “rigid” member, water may begin to accumulate inward of the intended free drainage point.
Construction Practices
Construction practices also contribute to ponding for minimum slope applications. Fascia members are often installed flush to the top edge of the framing member to create a horizontal surface. Detailed fascia members should be shown “dropped” to maintain the slope of the plane (Figure 6). Flashing is often installed at the boundary, with one leg placed on top of the roof or deck substrate. The material thickness at the boundary impedes water discharge (Figure 7). The substrate should be notched to receive flashing members and accommodate material thickness. These are two examples of a common framing practices that may be found within construction standards and implemented in the field. Material installation or thickness impact low-slope drainage and are often neglected at the time of design or during construction. A design professional should recognize the limits of building code requirements, standard details or practices; In these cases, it is important to provide “best practice” details within the construction documents to mitigate potential ponding and serviceability issues.
Conclusion
Accepted design and framing practices often contribute to serviceability issues with lowslope roof and deck applications. Practices or conditions that inhibit or prevent the flow of water toward free drainage should be identified during the design phase and changed. Design professionals have the ability to create in-service conditions that diminish ponding and promote free drainage. Slopes should be increased to maintain a sufficient slope to drain at intersecting planes. Framing members should provide the drainage plane and not rely completely on the slope of the topping or finish surface. Additionally, differential deflection of adjacent structural members should be investigated and the appropriate limit assigned to mitigate low areas for water retention. Framing practices and standard construction details often create high points that inhibit water drainage in low-slope applications. The design professional is encouraged to detail boundary conditions to promote drainage.▪ The online version of this article contains references. Please visit www.STRUCTUREmag.org.
CREATING A
HIGHER STANDARD
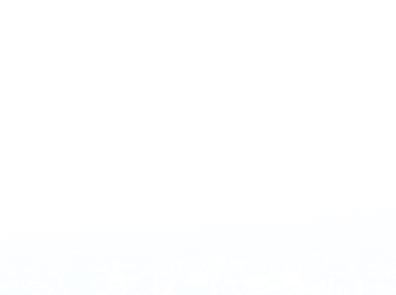
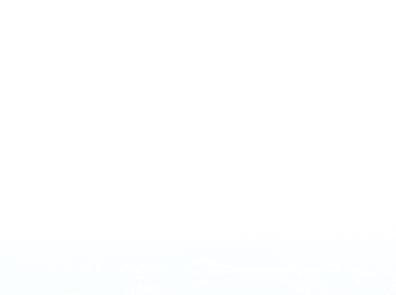
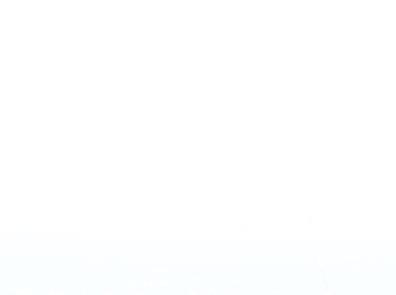
FOR THE MASONRY INDUSTRY
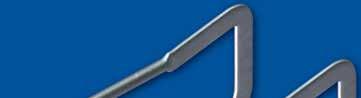
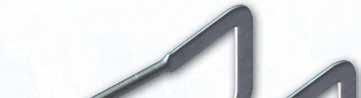

BIA TECHNICAL NOTE 44B:
“Specify ties with maximum defl ections of less than 0.05 in., or 1.2 mm, when tested at an axial load of 100 lbs. in tension or compression at maximum offset of 1¼ in. eccentricity.” 1¼” EFFECTIVE ADJUSTABILITY
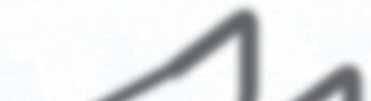
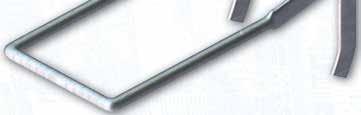
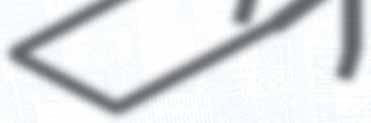
2X-STRONGER ANCHORING SYSTEMS
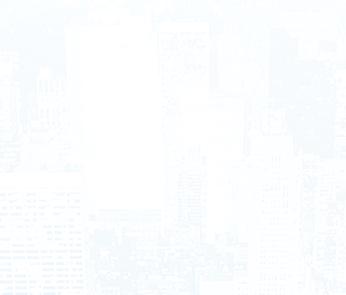
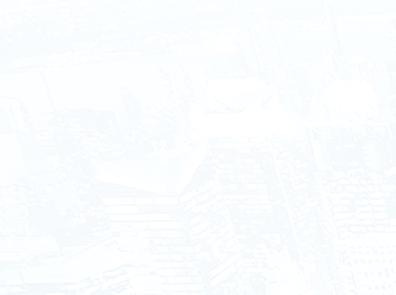
COMPRESSED VERTICAL LEGS
Hohmann & Barnard did extensive testing and discovered that compressing the legs of its pintles increases strength by over 100% compared to standard 3/16 ” diameter hooks.
The 2X-HOOK system tested at over 200 lbs. in tension or compression at 1¼” eccentricity in cavities up to 7½” wide!
2X-HOOK
U.S. Patent: 8,613,175 Other Patents Pending
MASONRY JOINT REINFORCEMENT
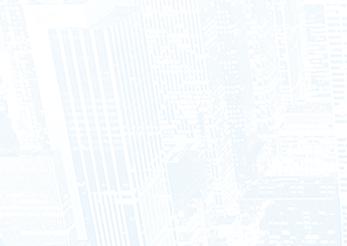
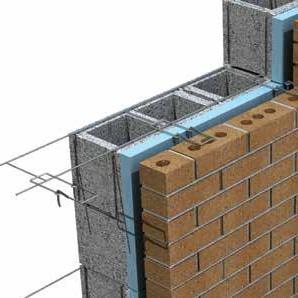
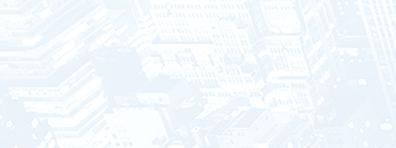
The 2X-HOOK can be used with H&B Lox-All® adjustable joint reinforcement systems for tying brick veneer to masonry backup walls. • Compressed legs fi t snuggly into eyelets tying both wyths together. • 3/16” diameter 2X-HOOK maintains a standard mortar bed while compressed legs provide over 100% greater strength. • Vertical adjustability of up to 1¼” for maximum alignment between backup and veneer.
• 2X-HOOK can be installed with legs pointing
UP or DOWN.
• Available in Truss or Ladder confi gurations.
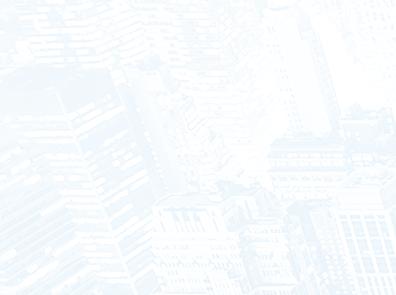
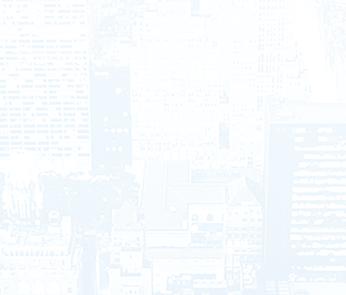
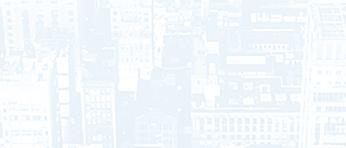
We Anchor The WORLD



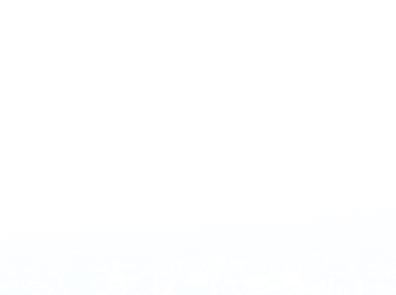
2-SEAL™ THERMAL WING NUT ANCHOR WING NUT ANCHOR
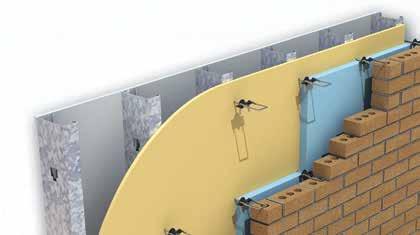
This innovative “wing anchor” features a steel reinforced wing that will not fail due to fi re like typical plastic only clip-on wings! • Dual-diameter barrel seals both the insulation and the air barrier. Dual-diameter barrel seals both the insulation and the air barrier. • Threaded wing body rotates for up to ½” inward adjustability, ” inward adjustability, accounting for variations in wall thickness and to easily orient accounting for variations in wall thickness and to easily orient 2X-HOOK with masonry joints. • Stainless steel barrel transfers one-seventh the thermal energy the thermal energy of standard zinc barrels.
• Steel reinforced wing maintains integrity Steel reinforced wing maintains integrity during NFPA 285 testing. during NFPA 285 testing.
• U.S. Patents: 7,415,803; 8,613,175; 8,910,445;
D702,544; D706,127
Other Patents Pending.
STEEL REINFORCED WING
HB-213 SERIES VENEER ANCHOR
HB-213 Series are adjustable veneer anchors designed to accommodate various wallboard and insulation combinations up to 6” thick while providing over 200 lb. wallboard and insulation combinations up to 6” thick while providing over 200 lb. working loads in tension or compression. • L-shaped plate with 9/32” diameter holes to accept mounting hardware. • Slot allows for pintle insertion and is sized to prevent in-and-out Slot allows for pintle insertion and is sized to prevent in-and-out movement beyond allowable tolerances. • U.S. Patents: 8,613,175. Other Patents Pending.
visit www.h-b.com or call 1-800-645-0616 TODAY!
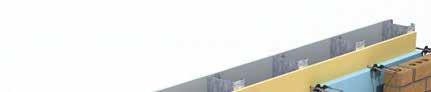
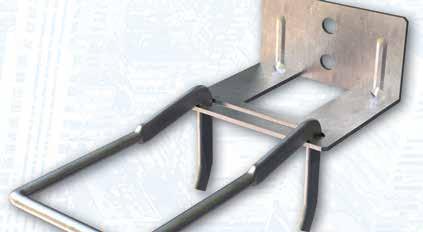
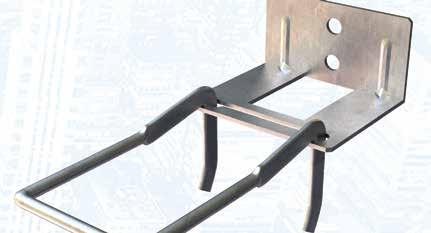
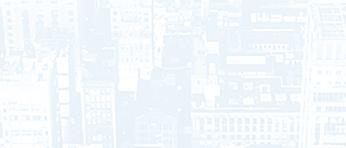
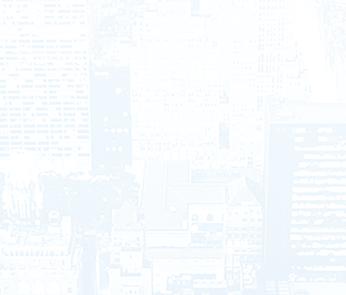
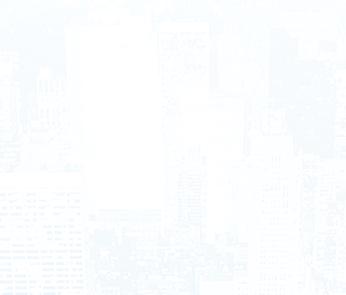
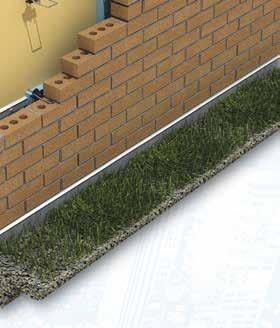
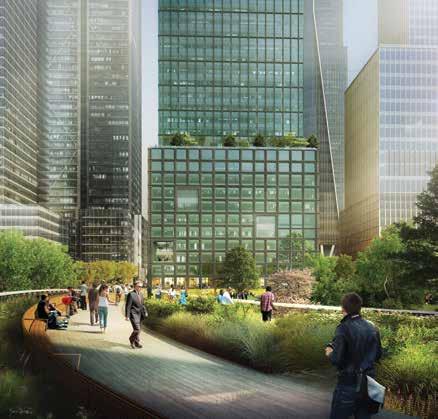
55
HUDSON YARDS
By Jeff rey Smilow, P.E., F.ASCE, Ahmad Rahimian, Ph.D., P.E., S.E., F.ASCE, and Lan-Cheng (Peter) Pan, Ph.D., P.E. Hudson Yards, located on the west side of Manhattan, is a 14-acre area experiencing a major expansion. is renewed interest in the parcel is spearheaded by Related/ Oxford Properties, who acquired the overbuild rights of the yards of the Long Island Railroad. Considered the largest private real estate development to date in the United States, the Related/ Oxford Properties Hudson Yards development will house about 19 million square feet of retail, commercial, residential, and mixed use spaces over the course of the next decade. Working on new projects within urban areas is always a challenge considering the numerous existing constraints, including subway infrastructure, underground utilities, neighboring buildings, and diffi cult construction logistics. Although the 55 Hudson Yards site initially appeared to be a sparsely developed block, in reality, the site presents complex and diffi cult site conditions. It partially sits on top of the 34th Street-Hudson Yards subway station for the new No. 7 New York City subway and is occupied by a 6-story subway ventilation building. Another unique aspect of the 55 Hudson Yards structure was the use of a post-tensioned lightweight concrete fl at slab fl oor system for a high rise commercial offi ce building.
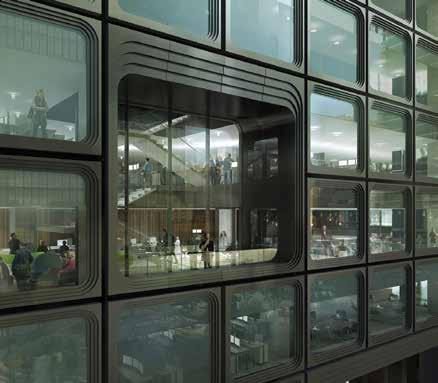
Project Evolution
e developer behind 55 Hudson Yards, Related/Oxford Properties, acquired the 55 Hudson Yards site from another major New York City (NYC) developer in early 2000. While the initial developer pursued potential tenants, early designs for a steel diagrid building were proposed, and negotiations with the Metropolitan Transit Authority (MTA) took place to accommodate the infrastructure for the new No. 7 subway line. e negotiations resulted in an agreement to build the 34th Street-Hudson Yards subway station with portions directly below the offi ce tower, including two major entrance escalators and a 6-story ventilation building. e agreement included a provision for the ventilation building and the below-grade subway station to have adequate capacity to support the proposed vertical and lateral loads imposed by the commercial offi ce tower. Towards the end of 2007, construction of the station and adjoining ventilation building began and, by the fall of 2015, they were in service. During the construction of the MTA infrastructure below and next to 55 Hudson Yards, the original developer sold the property and air rights to Related/Oxford Properties, which was constructing high-rise buildings adjacent to the 55 Hudson Yards site. Related/Oxford Properties immediately pursued the modifi cation of the original tower design to meet their vision of a 51-story tower with 1.3 million square feet of gross area for commercial use. ese changes resulted in architectural and
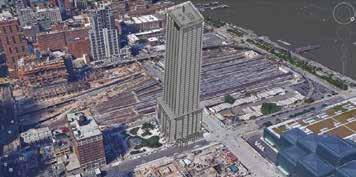
structural modifications specific to the tenant base considered by the new developer. The redesign of the 55 Hudson Yards tower included two key structural considerations: • Use of alternative structural materials, namely cast-in-place concrete, to meet the financial requirements of Related
Properties. (This original concept was converted into lightweight concrete to reduce the loading upon the existing subway structure.) • Redirecting the loads of the new tower to meet acceptable vertical and lateral load limits of the already built underground subway station and the 6-story subway ventilation building.
Project Description
The 55 Hudson Yards project is located in the western half of the block limited by West 34th and West 33rd streets to the north and south, respectively. To the east, the project neighbors Hudson Park, where the main entrance of the 34th Street-Hudson Yards subway station is located (Figure 1). Furthermore, the new building neatly extends above and along the north side of the existing 6-story subway ventilation building in the southwest corner of the lot. The architects of the project are Kohn Pedersen Fox and Kevin Roche (John Dinkeloo and Associates). WSP USA Inc. provided integrated engineering services for structural and MEP systems. The building is 51 stories high, divided into a 10-story podium and a 41-story tower, reaching 760 feet. The geometry of both the podium and the tower is approximately rectangular with column-free spans reaching almost 50 feet in the podium and more than 40 feet in the tower. The combination of site-related constraints triggered by the MTA infrastructure and the limitations associated with the original design posed engineering challenges addressed by unique and highly innovative structural solutions.
Foundation System
The main objectives of the foundation system envisioned for 55 Hudson Yards were to address the large interaction with existing MTA underground facilities and to minimize the impact in terms of loads transmitted to those facilities. The vertical load demands of the building were divided into three major components to provide adequate load-carrying capacity. Approximately 20% of the vertical load was transferred to the rock substrate by means of a reinforced concrete mat and footing foundations, about 40% was transferred using 10 reinforced concrete drilled caissons, ranging from 4.5 to 5.5 feet in diameter, and the remaining 40% was carried by the existing 6-story MTA ventilation building. The caissons were the primary support system for the concrete core and shear walls, carrying loads ranging from 10,000 to 33,000 kips. Complicating the foundation design was the fact that the location of the caissons was limited to the area between the two previously constructed subway entrance escalators (Figure 2). The acceptable diameter and location for the caissons required the reinforced concrete core and shear walls to cantilever beyond the caissons, resulting in a 20-foot deep caisson cap. All caissons utilized 12,000-psi grout and were embedded into rock sockets below the escalator base to adequately transfer their loads into the rock below the escalator shafts (Figure 3). The length of rock sockets ranged from 35 feet to approximately 100 feet. To limit the caissons diameter and maximize their load-carrying capacity, No. 28 reinforcing bars with 75-ksi yielding strength were used. In exceptional cases, a solid steel section was provided in lieu of reinforcing bars.
Superstructure
The superstructure of the 55 Hudson Yards project is based on a central reinforced concrete shear wall core and exterior moment frames connecting perimeter columns with a spandrel beam around the slab edge of the tower. It is complemented by a partial outrigger and belt system at the top (Figure 4, page 38). The structural solution provided by WSP addressed three main design objectives: • To follow the main architectural intent, which required longspan, column-free spaces. • To minimize the structural weight and thus meet the loadcarrying limitations of the existing 6-story MTA ventilation building and the underground subway station. • To provide flexibility for future modification to the floor plans, specifically allowing for additional slab openings in selected locations.
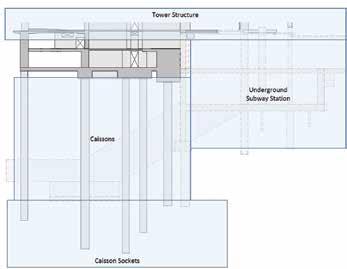
Figure 2. Foundation overview with detail of caissons between escalators.
continued on next page
Figure 4. Structural overview. 3D view from Northwest.
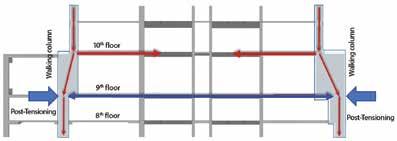
Figure 5. Example of 3D laser scan of as-built post-tensioning layout in a podium floor.
Figure 6. Load transfer mechanism using walking columns and post-tensioned slab. The floor system was solved using a post-tensioned lightweight concrete flat slab. Floor slab thickness was primarily 9 inches in the tower and 10 inches in the podium, with 18-inch thick floors in areas of major load transfers. The compressive strength of concrete for slabs was increased during the construction phase from 7,000 psi, as required by structural analysis and design, to 8,500 psi to accommodate the required construction schedule by applying post-tensioning as early as possible. The increase in compressive strength allowed for the early strength required prior to the post tensioning operation. Despite the use of a post-tensioned system, which is somewhat unusual in the New York City market, this solution was more attractive financially than the original steel frame solution. It also was able to accommodate both the load limitations imposed by the existing subway structure and the floor plan flexibility for tenants. The post-tensioned concrete slabs utilized blended lightweight concrete, which went through an extensive pre-construction sample mix design study to assure its suitability, pumpability, and constructability under the project conditions. Three-dimensional laser scans were carried out at each floor level to determine the precise final location of post-tensioning strands (Figure 5) to address floor plan flexibility for future tenants. The post-tensioned slab utilized a banded system, thereby grouping the post-tensioning bands on either side of potential future slab openings. The 9th floor is used as the primary mechanical floor and also serves as the interface between the podium and the tower floors. At this level, all the perimeter columns of the tower were transferred from a smaller tower footprint to the larger podium footprint. The transfer was accomplished with a walking column system where the 10th-floor slab carries in-plane compression loads, and the 9th-floor level carries in-plane tension loads. The 9th-floor tension forces were efficiently counteracted with undraped horizontal post tensioning (Figure 6). Finally, to limit lateral deflections and accelerations due to wind events, a full-story height, partial outrigger and belt system connecting the central shear wall core with a number of perimeter columns on the north end of the tower was incorporated at the 51st floor of the tower.
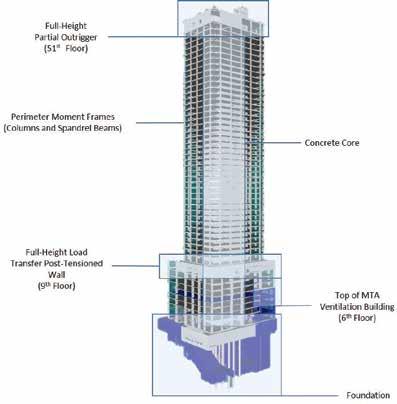
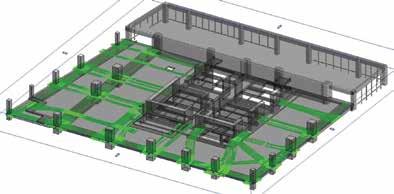
Wind Tunnel Testing
Comprehensive and project-specific wind tunnel tests were performed at the boundary layer wind tunnel lab at RWDI Consulting Engineers to determine direct wind pressures and wind-induced building accelerations. The industry-recommended comfort level for human occupancy in terms of acceleration due to wind for office buildings was achieved.
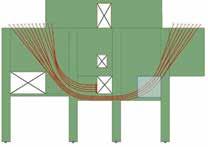
Structural Modeling and Innovation in Construction Sequencing
The structural modeling of 55 Hudson Yards included full three-dimensional sequential construction analysis with consideration to time-dependent changes in material properties and loading demands. This analysis was necessary to assure the short-term and long-term loading on all existing subway foundation elements was kept within acceptable limits. Two unique methods of load redirection were used to remove excessive loads from existing foundation elements or superstructure components of the subway structure. The
Figure 8. 55 Hudson Yards tower under construction as of May 2017. first method was the innovative use of construction sequencing devised to prevent overstressing of critical elements of the MTA structure. A multi-strand, grouted posttensioned system was used as a transfer wall to bridge loads away from the MTA structure (Figure 7). The second method employed temporary loadpath bridging to avoid specific locations with limited support capacity. After the appropriate amount of load had bypassed a specific location, the supporting structure was engaged using steel plate shims which then created a new continuous load path through the initially bypassed support points.
Material and Construction Innovations
High-performance structural materials were used in 55 Hudson Yards to provide adequate stiffness, strength, and resilience. For columns and shear walls, a concrete compressive strength of 12,000 psi was used in all podium floors, which gradually reduced to 7,000 psi at the 51st floor. Considering the load-carrying capacity limitation of the existing 6-story MTA ventilation building, it was crucial to reduce the weight of the structure without jeopardizing its structural integrity. With the use of 9-inch thick blended lightweight concrete slabs, the appropriate building weight was attained. The concrete supplier was able to develop, test, and successfully produce a mix design for a pumpable, lightweight concrete with a specified compressive strength of 8,500 psi at 56 days. The blended lightweight concrete was produced using both traditional lightweight and smaller diameter coarse aggregates and had a volumetric weight of approximately 130 pcf instead of the 150 pcf associated with normal weight concrete. In conclusion, this project demonstrates unique and innovative solutions to overcome existing site constraints. These constraints are becoming more prevalent in cities as developers seek out previously undeveloped land within urban centers. Also, the use of post tensioning, coupled with light weight concrete, a rarity on commercial high rises, is proven to be an economical alternative to structural steel framing. See Figure 8 for an overall view of the project under construction as of May 2017.▪
Jeffrey Smilow, P.E., F.ASCE, is Executive Vice President and USA Director of Building Structures at WSP USA, Principal in Charge of the project. Ahmad Rahimian, Ph.D., P.E., S.E., F.ASCE, is Technical Director and USA Director of Building Structures at WSP USA. Lan-Cheng (Peter) Pan, Ph.D., P.E., is Senior Associate of Building Structures at WSP USA, Project Manager.
ADVERTISEMENT–For Advertiser Information, visit www.STRUCTUREmag.org
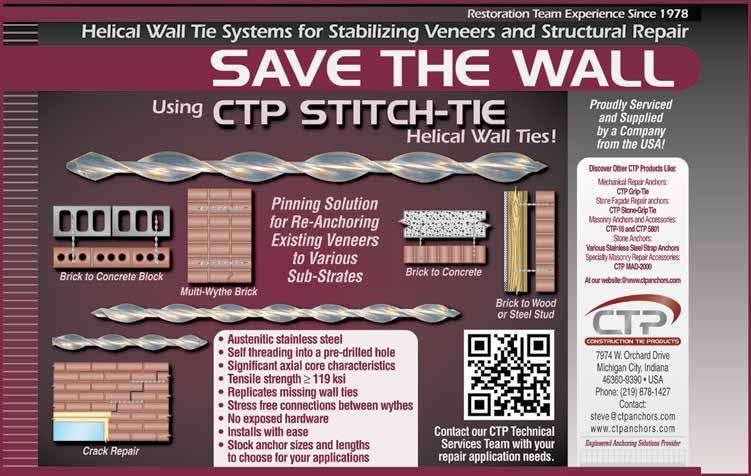