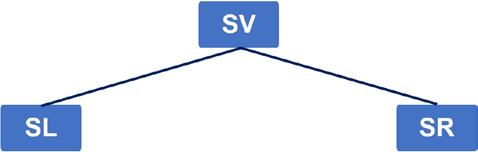
5 minute read
SWIMMING TECHNIQUE
BY ROD HAVRILUK
Advertisement
Many swimmers attempt to swim faster by modeling the technique of the fastest swimmers. Using champions as models is an archaic approach of painstakingly slow, trial-and-error that risks adopting technique limitations. A far superior approach is to apply mechanical principles that eliminate uncertainty and accelerate the skill-learning process. Unfortunately, modeling champions is well entrenched in swimming history.
EARLY HISTORY OF APPLYING SCIENCE
In his 1963 book, Coach Forbes Carlile’s chapters on “experiments” with style clearly documented the trial-and-error (i.e., non-scientific) process of improving swimming technique. However, his chapters on the technique of each of the four competitive strokes also included a number of technique elements that are clearly consistent with scientific principles. In spite of that, Carlile ultimately concluded that coaching technique “is more an art than a science,” stating that “we do not have hard and fast scientific principles to guide us.”
In contrast to Carlile, Coach Doc Counsilman applied many “hard and fast scientific principles” to improve technique in his 1968 book. The very first chapter was on “mechanical principles” and included buoyancy, Newton’s Law of Action-Reaction, the relationship of resistance and velocity, continuous source of propulsion, conservation of angular momentum and transfer of momentum. Each of these principles can be used to determine the most effective elements of swimming technique.
A SCIENTIFIC MODEL FOR PERFORMANCE
Doc’s application of the principles of physics to swimming is just as relevant, appropriate and accurate today as it was over a half century ago. As opposed to modeling the fastest swimmers, the application of mechanical principles has the advantage of certainty. The principles provide equations that definitively explain the relationships of component variables to allow the development of a performance model.
A “deterministic” model (Hay, 1983) begins with the relationship of the criterion measure (i.e., swimming velocity, SV) with its component variables (see Fig. 1). Swimming velocity is determined by the product of stroke length (SL) and stroke rate (SR). Adding variables to the model explains how a swimmer can benefit the most from both stroke length and stroke rate. A CONVENTIONAL APPROACH TO BREASTSTROKE BREATHING
The rationale for using the traditional approach of modeling champions is that a swimmer with a world-record performance must have exceptional technique. Superficially, the logic makes sense. In reality, countless analyses have shown technique limitations in every top swimmer.
A classic and very noticeable example of champion performance is the breaststroke breathing position (see Fig. 2). Elite swimmers typically have the head, chest and upper arms above the water surface. Science, however, disputes that this breathing position is the most effective.
A SCIENTIFIC APPROACH TO BREASTSTROKE BREATHING
Science suggests a different approach to breaststroke breathing based on the concepts of buoyancy and velocity fluctuations. Because of buoyant force, a human floats with about 6% of the body volume above the surface. A breaststroker in a typical breathing position often has the head, chest and upper arms above the surface for a total of 36% of the body volume. To elevate the additional 30% of body volume above the surface, a swimmer must generate considerable vertical force that does not contribute to horizontal propulsion.
Consistent with the conventional breathing position is another factor that makes a compelling argument against elevating body segments above the surface. A review of typical body velocity changes within a stroke cycle shows a severe loss in velocity during the breathing phase. The graph in Fig. 3 shows the mean body velocity for nine adult-male, elite breaststrokers. As the swimmers changed their head positions to breathe, their velocity decreased almost 2 meters per second. For about 10% of the stroke cycle, their bodies were barely moving forward. The graph pinpoints the stroke phase that seriously limits performance.
The scientific approach suggests that keeping the body submerged and extending at the neck to position the mouth above the surface (see Fig. 4) is more effective. A more submerged position greatly minimizes the downward force necessary to counter the loss in buoyancy (from 36% to 9%) and would also minimize the loss in body velocity.
SERIES ON MECHANICAL PRINCIPLES
Breaststroke breathing is just one example of applying science to determine effective technique. There are many other mechanical principles that can likewise verify or dispute technique elements:
• The Drag Equation provides variables related to stroke length to maximize swimming velocity. The equation includes the Drag Coefficient, which provides the overall best measure of technique effectiveness to quantify improvement.
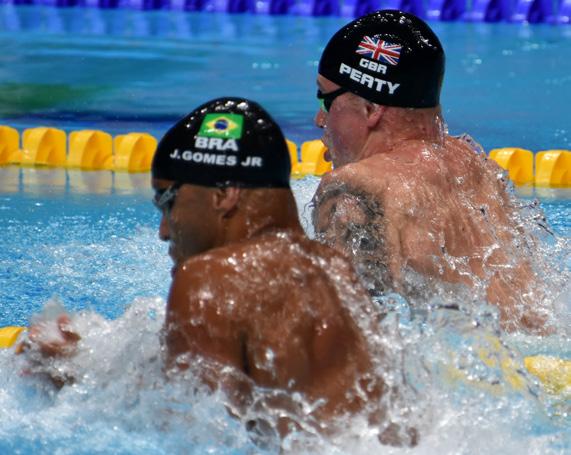
FIG. 2 > Breaststrokers in a typical breathing position with head, chest and upper arms above the surface.
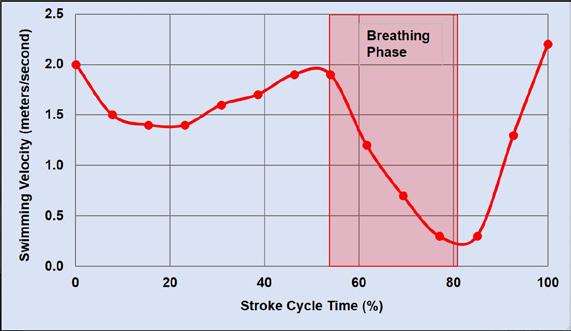
FIG. 3 > Breaststroke swimming velocity plotted against stroke cycle time. • Leverage determines the strength of the relative position of body segments and identifies ways to increase propulsion.
• Conservation of Angular Momentum
explains the impact of an arm recovering above the surface.
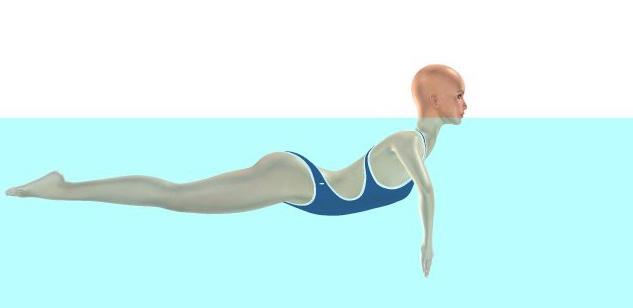
• Conservation of Energy explains how variations in intracycle body velocity affect performance. • Summation of Forces explains the direction and timing of successive body segments to maximize propulsion in a motion like flutter kicking.
Application of these principles provides the equations to accurately assess factors such as body position, joint angles and the coordination of limb movements. The purpose of the following series of articles is to provide information about the mechanical principles and related research so that coaches and swimmers can make informed decisions about technique elements.
Next month: “Swimming Velocity Performance Model (Part 1),” which explains how stroke length and stroke rate vary, and how stroke time provides insight into maximizing swimming velocity.
Dr. Rod Havriluk is a sport scientist and consultant who specializes in swimming technique instruction and analysis. His newest ebooks in the “Approaching Perfect Swimming” series are “Optimal Stroke Technique” and “Swimming Without Pain,” and are available at swimmingtechnology. com. Contact Rod through info@ swimmingtechnology.com. All scientific documentation relating to this article, including scientific principles, studies and research papers, can be provided upon demand.
SUMMARY
The conventional, laborious and outdated approach to improve swimming technique is to model a champion. The main problem with the conventional approach is that every top swimmer has technique limitations. When modeling a champion, a swimmer risks adopting his/her limitations. Using a scientific approach, a swimmer can be certain to adopt only beneficial technique elements.
TOTAL ACCESS MEMBERS CLICK HERE TO LEARN MORE ABOUT THE REFERENCES FOR THIS ARTICLE.
NOT A TOTAL ACCESS MEMBER?
YOU’RE JUST A CLICK AWAY: SWIMMINGWORLD.COM/VAULT