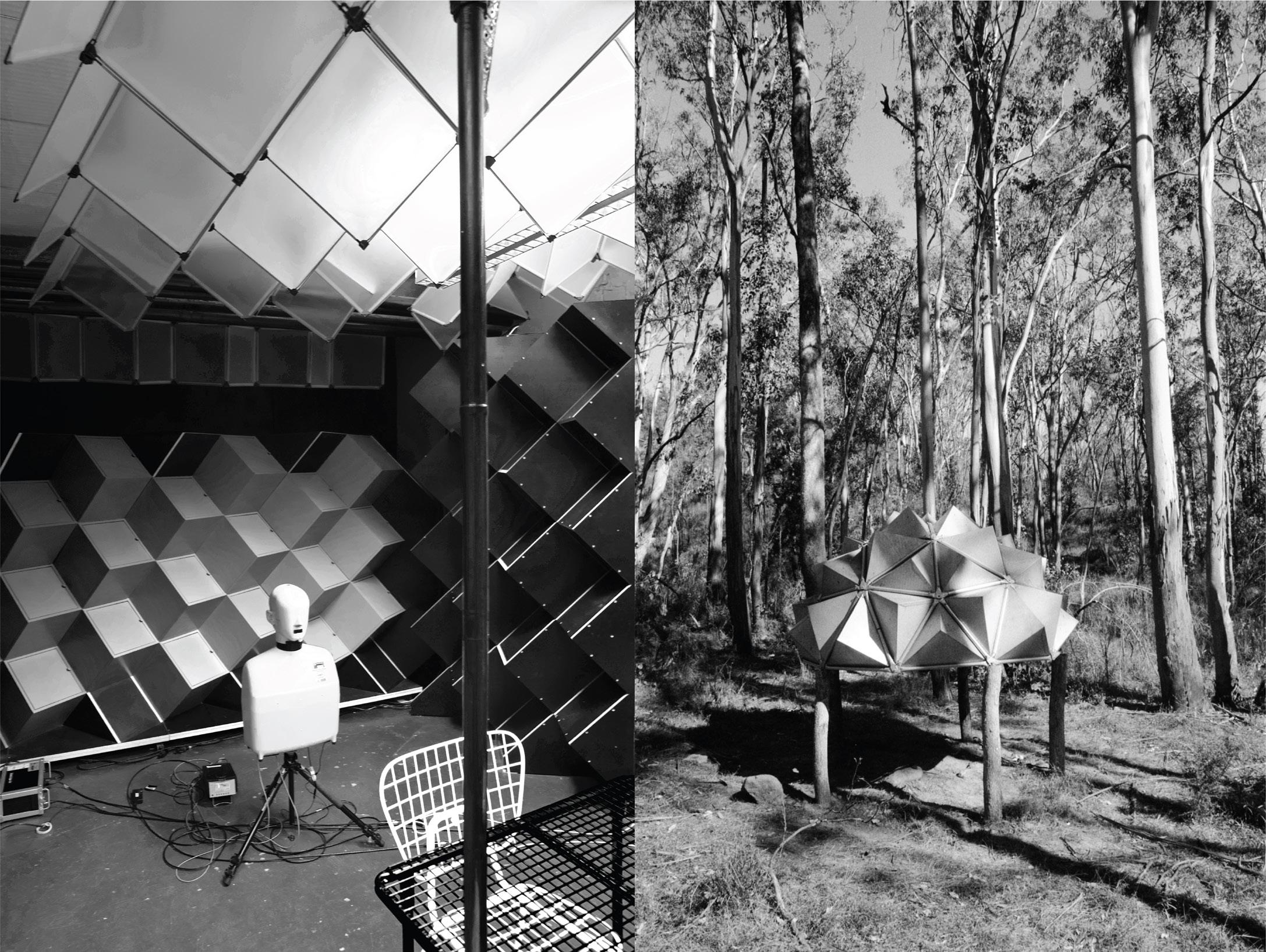
5 minute read
A Reflection on Acoustic Retroflection in Architecture Densil Cabrera
A Reflection on Acoustic Retroreflection in Architecture
Densil Cabrera Associate Professor
Advertisement
Architectural acoustics is known for its surprises and delights, as much as for the mundane headaches of the sound environment. Over recent years my colleagues and I have been experimenting with something that we consider to be a delight: acoustically retroreflective surfaces. Just as a bicycle reflector reflects light back in the direction from which it came, architectural surfaces can be designed to reflect sound back to the source, wherever that source is.
But sound is different to light in many ways, both physical and perceptual. The physical wavelengths of sound are on a human scale (ranging between 17 m to 17 mm – shorter wavelengths for higher frequencies), whereas wavelengths of light are miniscule. This comes into play when we design surfaces to reflect sound – facets must be large enough for the relevant waves. Furthermore, people engage with sound differently to light: speaking and hearing are very different to seeing. So retroreflection of sound is designed on a different scale to optical retroreflection, and is phenomenologically distinct. In these respects, we are not strictly designing acoustic bicycle reflectors.
There are various ways by which acoustically retroreflective surfaces can be made, but an array of concave right-angle corners is certainly the easiest. The intersecting faces that form the corners need to have edge lengths in the 0.2 – 1 m range to have an appreciable audible effect on one’s own voice. There is a trade-off here between reflector size, number of reflectors, and distance – which affects the frequency range over which they are effective, along with the overall strength of the reflection onto the source. Reflectors that are too small or too distant will only affect very high frequencies, perhaps too high to affect the audible quality of the space.
My colleagues and I have designed and built numerous prototypes, and in our more mature work have created a room 50% covered with acoustic retroreflectors. It seems likely that this is the world’s first intentionally designed, predominantly acoustically retroreflective room. Nevertheless, acoustic retrore-
flection is found elsewhere in architecture. It occurs incidentally in certain building facades (e.g., the Ports 1961 Flagship Store in Shanghai), but also in more conventional facades. It is also seen incidentally in some Indian stepwells (e.g., Chand Baori in Abhaneri, Rajasthan). It is used intentionally in certain auditoria, where right-angle reflectors are used to help support the musical performers. Interestingly, the Sydney Opera House’s concert hall, in its original construction, is a case in point – but its retroreflective treatment (in the form of right-angle saw-tooth surfaces) was removed because of the perceived over-emphasis of high frequencies in the reflections.
Retroreflective surfaces reflect back to a person as they speak. They create a crisp sound for one’s own voice. When speaking, I particularly hear consonants like /s/, /sh/, /ch/, /k/ and /t/ very strongly. These phonemes are energetic in the high frequency range. To me this crisp characteristic is not disturbing – rather I find that it contributes to speaking comfort.
Research literature on acoustic voice support has repeatedly shown that people find acoustically supportive rooms (in which they hear their own voice strongly) to be comfortable for speaking in, and that this leads to more relaxed voice projection. This has been studied particularly in classrooms, where sufficient acoustic voice support reduces teacher voice strain. Our laboratory research into the type of voice support offered by retroreflective treatment (i.e., restricted to high frequencies) has shown that it too leads people to instinctively speak in a more relaxed manner.
My impression is that acoustically retroreflective treatment creates a particularly intimate acoustic quality. The room sounds like a smaller space than its physical size, and I think that this adds to the comfortable impression of the room’s acoustics. This auditory spatial intimacy might be explained from the fact that smaller rooms tend to be louder and less reverberant, both of which are achieved by the retroreflective treatment.
My colleague Mary Rapp (musician and PhD student at USYD) has designed and built a retroreflective dome (2m diameter) which is installed in the remote Burragorang Valley. She uses this for singing practice, based on the Korean tradition of P’ansori. The dome provides an extraordinarily high level of acoustic support, allowing Mary to focus on details of quiet singing, in contrast to her powerful singing practice at a nearby waterfall.
A less esoteric potential practical application for retroreflective acoustics might be multi-talker environments. For example, in open-plan offices, distraction from other people’s speech is a notorious and widely researched problem. When people can hear their own voice better, they speak more quietly. Hence a retroreflective ceiling will concentrate the sound around a talking person, reducing both the voice projection used by the person and the relative transmission of sound across the room. Further experiments are needed to see whether this actually ameliorates the problem of speech distraction.
Why are retroreflective treatments not in the standard repertoire for architectural acoustic design? A practical reason might be the relatively large surface modulations that are needed to create simple retroreflectors – which may be unwanted in many practical situations. However, there are alternatives to the arrays of right-angles that we have been using in our experiments – and perhaps it is possible to create surfaces that are more spatially efficient and less visually striking. A deeper reason is that the commonly used theories of room acoustics, and the widely used acoustic simulation software, only account for two types of reflection (specular reflections and scattering). The issues with such simulation software come from ray-based algorithms of sound propagation that might be thought of as having blind-spots to retroreflection and other anomalous reflections. These tools close off opportunities and limit the acoustic designer’s imagination.
Fortunately, present day computational capacity makes wave-based acoustic simulation accessible, allowing us to simulate the effects of retroreflective surfaces – at least in the university context. Increasingly this will become accessible to professional designers.
Acoustically retroreflective surfaces for architectural acoustics need more research. One area that we have been exploring is the optimization of surfaces, so that they reflect even more sound back to the source. This is done by curving the surfaces to provide a quasi-focus onto the target area of a room – using 3D parametric modelling coupled with acoustic wave-based simulation. Our prototypes work well. Another challenge is to make visually innocuous and spatially compact retroreflectors, which can be done using other retroreflection techniques. Beyond passive acoustics treatment, we have also been thinking about active techniques for retroreflection, including the fascinating potential of time-reversal acoustics processing. Regardless of the techniques used, we need to develop a deeper understanding of how people respond to retroreflective treatments, though large-scale prototype installations.