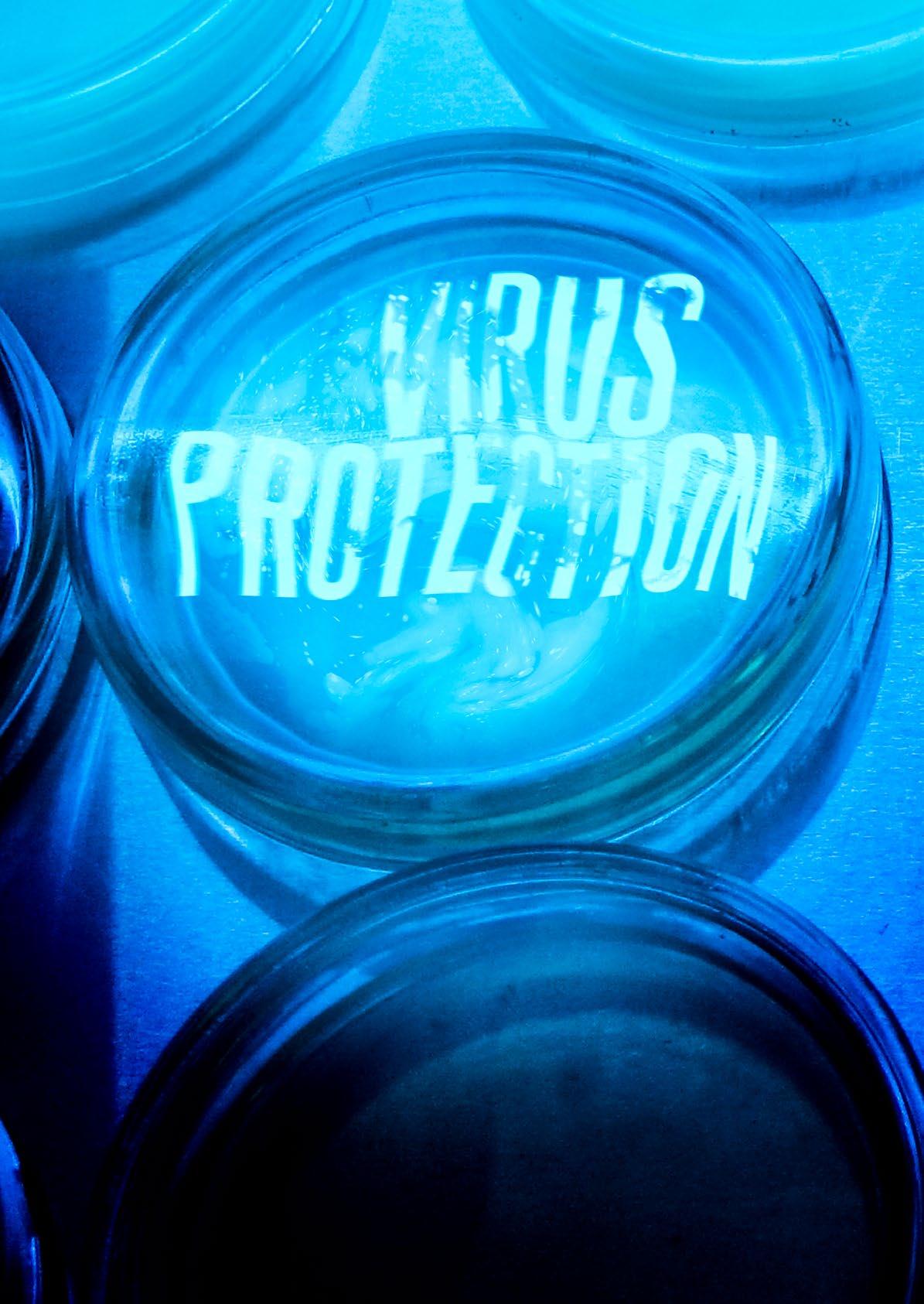
15 minute read
VIRUS PROTECTION
from Lighting Journal April 22
by The ILP
The Covid-19 pandemic has put a spotlight like never before on the role of ultra-violet light for germicidal irradiation. With so many claims and counter-claims for what works and what doesn’t, Mike Simpson explains what you need to know
By Mike Simpson
Ultra-violet light
When discussing ultra-violet light for germicidal irradiation, especially after the two years of pandemic we’ve just been through, let’s start with a few facts you might not like to learn about:
• Some cold and flu viruses can survive on surfaces for up to 72 hours • Supermarket fridge doors have 1,235 times more bacteria than the surface of your mobile phone • Supermarket trolley handles can have 361 times more bacteria than a bathroom doorknob
We have lived with viruses and bacteria for many years, but the current pandemic has of course thrown this into a new light.
In the early stages of the pandemic, there was a lot of focus on cleaning surfaces and washing hands. However, by the middle of 2021 it was realised that the predominant method of transmission was through the air.
As the World Health Organization put it: ‘The virus can also spread in poorly ventilated rooms and/or crowded settings where people tend to spend longer periods of time. This is because aerosols remain in the air or travel further than 1m.’ [1]
Or equally, take these comments from the Centers for Disease Control and Prevention in the US last year: ‘Breathing in air when close to an infected person who is exhaling small droplets and particles that contain the virus is a key way the virus is transmitted.’ [2]
Similarly, the use of ultra-violet radiation for disinfection is not new. The germicidal effects of short wave ultra-violet (UVC) light were first discovered in 1877 and the 1903 Nobel Prize for Medicine was awarded to Niels Finsen for his use of UV-C against tuberculosis of the skin. Using UV-C for disinfection of drinking water dates from 1910 in Marseille and between 1937 and 1941 upper-room UV-C was used in suburban Philadelphia schools to prevent the spread of measles.
UV-C has of course gained new attention during the pandemic as a way of reducing the effects of the Sars-CoV-2 virus. Ultra-violet germicidal irradiation (UVGI) can disable the structural bonds in the DNA of the virus preventing it from reproducing, as shown in figure 1 below.
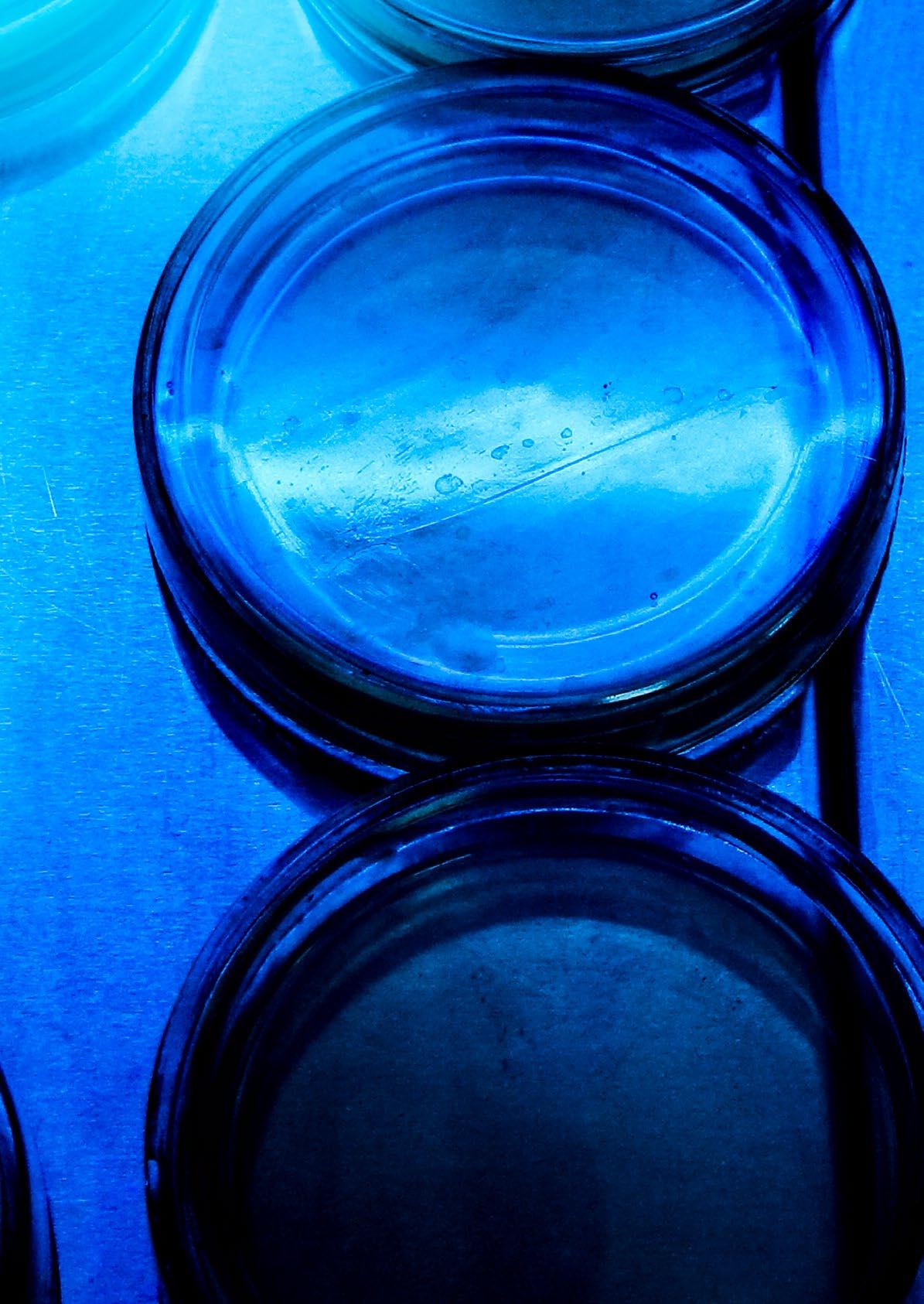
UNDERSTANDING UV-C
Ultra-violet C (UV-C) is ultra-violet radiation with wavelengths between 200nm and 290nm. The most common sources of UV-C are gas discharge lamps in various formats. The most used look like traditional fluorescent lamps without the phosphor coating, which produce radiation at a wavelength of 254nm. Other types (such as KrCl) produce radiation at 222nm and there are developments in LED technology that will also produce UV-C.
The amount of UV-C required to disable the virus is called ‘the Dose’, measured in Joules/m2. This is the product of the irradiation and the time. The susceptibility of the virus to the UV-C also has to be taken into account by what is known as its k(λ) factor, which varies from virus to virus, and whether it is on a dry surface of in suspension in droplets (ISO15714) [3]. It is also affected by the wavelength of UV-C used. For lighting people, think of the irradiation as lux but without the eye sensitivity component. Then multiply by the time to get the Dose. For the irradiation of surfaces, it is possible to use standard lighting software but
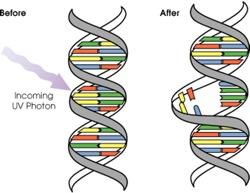
Figure 1. Action of UV-C on DNA
Ultra-violet light
with units re-calibrated into Wm-2 instead of lux. For disinfection in air, it is rather more complex, as we have to think in terms of ‘Spherical Irradiance’, which has the term ‘Fluence Rate’ and the Dose is termed ‘Fluence’.
To calculate the Fluence we need to know how long a virus suspended in a droplet will be in irradiated. This means we have to predict the air movement within a space. Whilst this can be done by computational fluid dynamics, the technique involves complex modelling.
So, when designing air disinfection systems, we usually just calculate the Fluence Rate together with an understanding that there is air movement and mixing in the room.
Another term used is the ‘log’ reduction. This is simply the % reduction in the virus over a given time. A log1 reduction is 90%, log2 is 99%, log3 is 99.9% and so on. With a grasp of Irradiance, Fluence, Fluence Rate and Log reduction the lighting designer can transition into a UV-C designer.
For the lighting designer, the shift to calculating with UV-C will see them using similar techniques as they do for light with a few differences. When calculating the surface irradiance, we are looking for the minimum not the average as we want to ensure we can eliminate viruses in all locations.
Also, the reflection properties of surfaces to UV-C are quite different to light and depend on the material. A dark surface, for example, might have a higher UV-C reflection compared to a light one. The table below (figure 2) shows typical values for different surfaces.
There has been much research into the effect of UV-C on viruses such as tuberculosis. Since the start of the pandemic, new research has been carried out to confirm that the Sars-CoV-2 virus responds to UV-C in the same way as other viruses.
For example, research conducted by The National Emerging Infectious Diseases Laboratories (NEIDL) at Boston University demonstrated that a UVC dose of 290 Jm-2 could achieve a log4 reduction of the Sars-CoV-2 virus on surfaces [4]. Other research has also shown a room with an average Fluence Rate of 40 mWm-2 gave a log4 reduction in airborne viruses in ten minutes [5].
REFLECTION FACTORS FOR UV-C RADIATION
MATERIAL REFLECTANCE %
Aluminium: untreated surface 40-60
Aluminium: sputtered on glass 75-85 Stainless steel / Tin plate 25-30 Chromium plating 39 Various white oil paints 03-10 Various white-water paints 10-35 Aluminium paint 40-75 Zinc oxide paint 4-5 Glass 2
White plastering Painted Wall 40-60
5-15
Calcium carbonate 70-80
Figure 2. Some typical reflectance values for a range of different surfaces APPLYING TECHNOLGY IN PRACTICE
So how is this technology deployed in practice? For surface disinfection we can install UV-C in battens over the area to be disinfected.
However, only the surfaces in direct sight of the UV-C source will be treated, so anywhere where there are shadows will be missed. It is better to use fewer smaller sources that will create fewer shadows than a single larger one.
Once this has been calculated, we can work out the duration of the exposure to give the Dose. Disinfection chambers can be used for small items such as keys and phones.
There are various ways that air can be treated by UV-C:
• Upper-room UVGI where a narrow beam of UV-C is projected onto the air above head height to deactivate viral material, relying on air mixing in the space. • In-duct UVGI where UV-C lamps are
installed in the return or supply air ducts of mechanical ventilation systems to disinfect the air. • UVGI air cleaners where UV-C lamps are mounted inside an enclosure, with a fan to draw air through.
One important factor in the design of a UVGI system is safety and we need to consider the effects both on the skin (erythema) and the eye (photokeratitis).
Penetration into the epidermis layer of the skin is wavelength-dependent, so maximum dose differs depending on the source technology. ISO 15858 allows a maximum dose of 60 Jm-2 per day for a wavelength of 254nm [6].
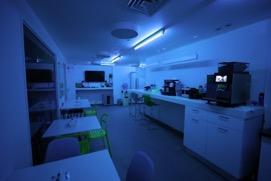
Surface disinfection from UV-C battens in a laboratory
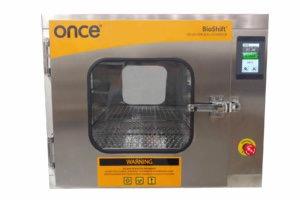
A chamber for disinfection of small objects SURFACE VERSUS AIR SOLUTIONS
Bear in mind that most systems for surface disinfection would exceed this limit, so the design should take into account the time required to achieve the correct dose and ensure that no access can be made into the room while the UV-C is turned on.
In practice this solution is most commonly used in specialised areas where the risk of contamination is highest or where surfaces are frequently touched by different people (for example, washrooms). With the correct Dose it is possible to achieve disinfection in a few minutes so a simple interlock with the door will stop entry.
Upper-air solutions require the system to be operating while the room is occupied to disinfect the air at source. This means that, whilst a high irradiation is required at ceiling height, it must be limited at head height (1.83m). These units typically produce a narrow beam, in the order of a few degrees parallel to the ceiling.
In these situations, the reflection of the UV-C from the ceiling must be considered

Upper-air UVGI, as shown in a recent Signify partnership with building technologies firm Honeywell (and see panel at the end for more on this). The small amount of visible blue light in the beam shows where the UV-C is
Ultra-violet light
as it can make significant contribution to the UV-C at head height. A calcium whitener in a ceiling tile, for example, will cause it to be highly reflective to UV-C, whereas a white acrylic paint will hardly reflect any.
With upper-air solutions, you should also consider how the room is being used. For example, in a typical office it is unlikely that anyone will be standing in the exact spot where the maximum irradiance occurs for eight hours. So, a calculation is also made for the time spent sitting and the total daily dose calculated. For upper air systems we have to calculate both the Fluence Rate, which gives us the effectiveness of disinfection, and check the safe Dose for exposure.
It is recognised that air movement in a room will help disperse airborne viruses and often this is related to the air changes per hour (ACH).
The basic ventilation rate required for fresh air, low CO2 and odor levels is mostly accomplished with 1-6 ACH, sometimes up to 8 ACH.
Opening windows gives 1-2 ACH, maybe higher, but with discomfort. Increasing the basic ventilation rate significantly, for example to ACH 15-20, is a well-known method to reduce pathogen levels for disinfection, especially in healthcare applications.
Experiments with upper-air UVGI solutions have shown that the disinfection achieved can be equivalent to up to 50 ACH (eqACH), providing there is basic air movement in the room to start with. Equivalent ACH is related to average Fluence Rate by the following formula:
eqACH = Eem x 3600 x k(λ) /1000
where
k(λ) is the spectral susceptibility factor of airborne pathogens (m2 J-1) Eem is the average Fluence Rate (mW m-2)
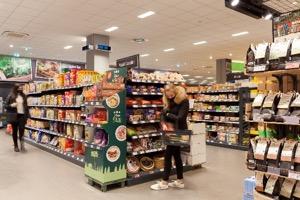
In his paper Nardell proposes that the economic efficiency of UVGI is 9.41x that of HVAC for the same level of disinfection [7].
Calculating for surface disinfection can be done with any standard lighting software. You just need to use units of mW instead of lumens and you will get an irradiance in mWm-2. Then multiply by time to get the dose. Opposite (right) are two images for a washroom application.
The grey scale shows the visualization of the space, the colours represent the irradiance values going from yellow (highest) to black (lowest). From this it is easy to see what areas are receiving sufficient irradiation and what are in shadow.
Calculating for air disinfection requires a 3D grid of points in the volume of the room with spherical irradiance calculated at each. These are then averaged to give the Fluence Rate.
A separate calculation is made on a horizontal plane(s) at a height of 1.83m* and 1.3m to check maximum the exposure is not exceeded [8].
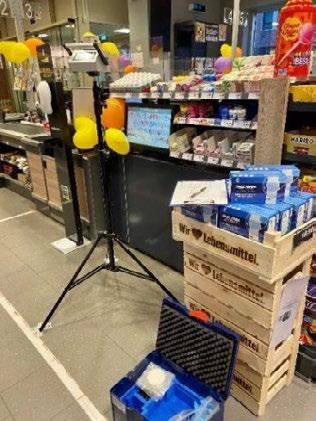
Above: 3D model created in design software Right: 3D pseudo colour representation of irradiance
Above: 3D grid of calculation points in a room Right: 2D grids at heights of 1.83m and 1.3m to check safe exposure
An Edeka store, with UVGI mounted on columns
Edeka air sampling in situ
Samples showing with (left) and without (right) UV-C
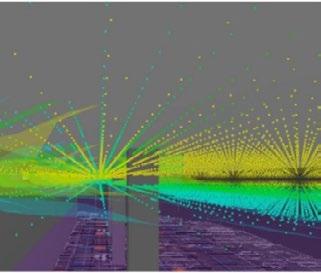
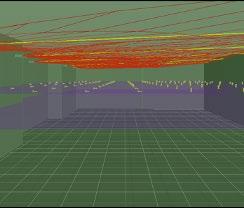
GERMAN SUPERMARKET EXPERIMENT
We have looked at the theory of how UV-C works and how you design an installation. But does it work in practice, can you measure the effect?
It’s obviously impossible to pump the Sars-CoV-2 virus into an occupied room, but you can measure the effect on naturally occurring fungi and bacteria and predict the effect for Sars-CoV-2.
The German food chain Edeka installed upper-air UVGI followed by independent in store tests by the Fraunhofer Institute [9]. Air measuring devices were located around the store and the UVGI switched on and off on alternate days over a ten-day period.
During the time with highest customer use, a fungal reduction of 50% was observed. Given that the susceptibility of Sars-CoV-2 is 20 times lower, Fraunhofer estimated that 99.99% of the virus would be inactivated. The images opposite help to illustrate this.
Ultra-violet light
We have also seen the rise in popularity of free-standing UVGI cleaners which are portable and can be easily moved into rooms. These have internal fans to move the air past UC-V lamps inside. The level of disinfection will depend on the dose, which in turn depends on the air speed, irradiance and length of the unit.
This is usually quantified by the ‘Clean
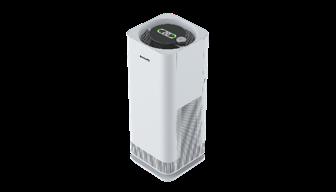
A free-standing UVGI disinfection unit
Air Delivery Rate’, defined for HVAC systems by EN1822-1 [10]. The effectiveness of such units can also be equated to an ‘eqACH’, which determines the number of units needed for a specific room size. A target of six eqACH is minimum for the mitigation of risk. Another approach, using EN16798-2, is to ensure a clean air delivery of 25 m3h-1 per person [11].
CONCLUSIONS
UVGI is not a new technology but is being rediscovered in the current pandemic as an effective solution, alongside other measures, to reduce the risk of infection.
It is now being deployed in a wide range of applications, including schools, offices, gyms, hospitality and so on. So, what about the future?
The current pandemic has highlighted the fact that clean air and good circulation will contribute to a reduction in the transmission of airborne diseases.
UVGI is another tool an important weapon in our armoury in the fight against the spread of viruses. Although today the focus has been on Sars-CoV-2, UVGI works on a wide range of pathogens including seasonally recurring flu.
UVGI should therefore not be thought of as just a solution for now but as a permanent solution to help create healthy places where we can all work, rest and play.
Mike Simpson FREng CEng FCIBSE FSLL FILP FIET is global application lead at Signify
UV-C PARTNERSHIP
As Mike Simpson has shown in this article, the appetite for UVGI, though not a new technology, is being rediscovered in the pandemic because it can be an effective solution to reduce the risk of infection, writes Nic Paton.
This commitment to UV-C disinfection lighting has been illustrated in a recent partnership between Signify and building technologies firm Honeywell.
The collaboration integrated Signify’s Interact connected lighting system and software, plus its UV-C disinfection lighting, with Honeywell’s building management systems and its Honeywell Forge enterprise performance management platform (as shown in the image opposite and on page eight).
The intention is that the combined offerings will enable the management of energy consumption while factoring in occupancy along with air quality indicators, such as temperature and humidity. Signify’s lighting will also complement Honeywell’s ‘Healthy Buildings’ air quality solutions.
‘Increasingly we see lighting systems playing a critical role in buildings to improve occupant comfort, wellbeing and productivity as well as to help meet energy savings goals. We anticipate this trend will continue to grow,’ said Vimal Kapur, president and CEO of Honeywell Building Technologies.
‘There are known benefits of how lighting can improve occupant experience and wellbeing,’ agreed Harsh Chitale, leader of Signify’s Digital Solutions Division. ‘Many of our customers expect our solutions to deliver value beyond the scope of lighting.’
The two companies have recently installed the combined offering at a series of eye clinics in Malaysia run by ophthalmology firm Optimax, with a priority to help the organisation improve its air quality and surface disinfection.
Honeywell and Signify are also deploying Signify’s Philips UV-C disinfection upper-air luminaires in several Honeywell global offices.
[1] ‘Coronavirus disease (COVID-19): How is it transmitted?’, World Health Organization, December 2021, https://www.who.int/news-room/questions-and-answers/item/coronavirus-disease-covid-19-how-is-it-transmitted [2] ‘How Covid-19 spreads’, CDC, https://www.cdc.gov/ socialmedia/syndication/405380/403327.html [3] BS ISO 15714:2019: Method of evaluating the UV dose to airborne microorganisms transiting in-duct ultraviolet germicidal irradiation devices, ISO 2019, https://www.iso.org/standard/67814.html [4] Storm, N, McKay, L G A., Downs, S N et al (2020). ‘Rapid and complete inactivation of SARS-CoV-2 by ultraviolet-C irradiation’. Scientific Reports 10, 22421 (2020). Available online at: https://doi.org/10.1038/s41598-020-79600-8 [5] ‘Efficacy of a wall-mounted UV device against aerosolized SARS-CoV-2’, Innovative Bioanalysis, March 2021. Available online at: https://www.assets.signify.com/is/content/Signify/Assets/philips-lighting/global/20210301-innovative-bioanalysis-report-sars-cov-2.pdf [6] ISO 15858:2016 – UV-C Devices – safety information, https://www.iso.org/standard/55553.html [7] Nardell E A (2021). ‘Air Disinfection for Airborne Infection Control with a Focus on COVID-19: Why Germicidal UV is Essential’, Photochemistry and Photobiology, 2021 May;97(3):493-497. Available online at: https://pubmed.ncbi.nlm.nih.gov/33759191/ [8] Bear in mind, the standard here was originally written in imperial units where 1.83m = 8’ [9] ‘Study on the evaluation of the efficiency of UV-C radiation as an air disinfectant during operating hours in a supermarket’, Signify, May 2021, https://www.signify.com/global/our-company/blog/innovation/versatility-ofUVC-air-disinfection?linkId=132626199 [10] BS EN 1822-1:2019. ‘High efficiency air filters (EPA, HEPA and ULPA) Classification, performance testing, marking’, https://www.en-standard.eu/bs-en-1822-1-2019-high-efficiency-air-filters-epa-hepa-and-ulpa-classification-performance-testingmarking/ [11] BS EN 16798-5-2:2017. ‘Energy performance of buildings. Ventilation for buildings Calculation methods for energy requirements of ventilation systems’, https://www.en-standard.eu/bs-en-16798-5-2-2017-energy-performance-of-buildings-ventilation-for-buildings-calculationmethods-for-energy-requirements-of-ventilation-systems-modules-m5-6-m5-8-m6-5-m6-8-m7-5-m7-8-method-2-distribution-and-generation/