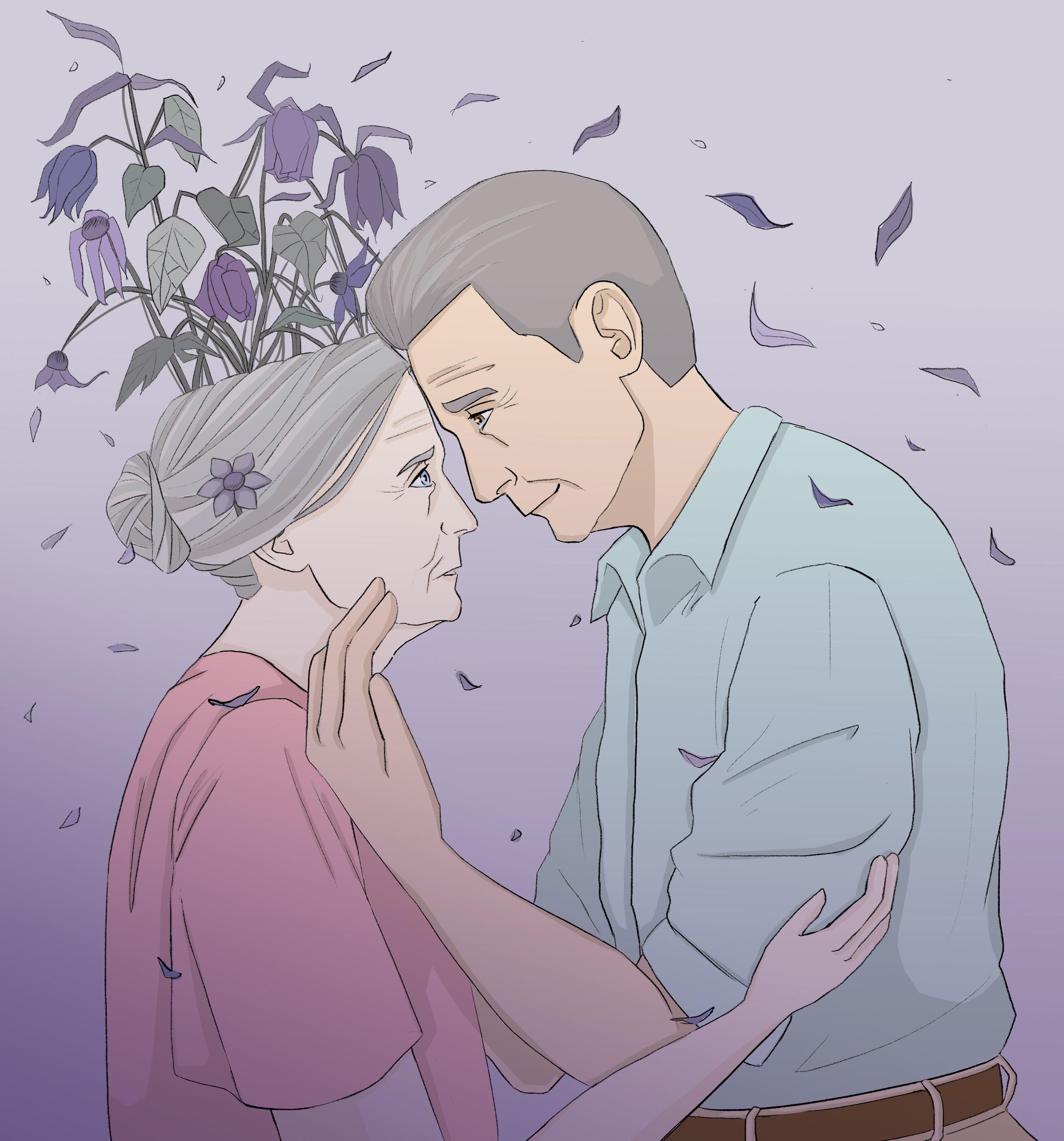
33 minute read
ALZHEIMER’S
from Issue 37
Tau and beta-amyloid in Alzheimer’s disease: Theories, treatments strategies, and future directions CRITICAL REVIEW
ABSTRACT Alzheimer’s disease is a severe neurodegenerative disease characterized by the deposition of neuritic plaques on neuronal membranes and the formation of neurofibrillary tangles within neurons. Several proteins, such as amyloid beta and tau, play major roles in the pathophysiology and progression of Alzheimer’s disease and are important factors to consider when developing novel therapeutics. The following review outlines the current understanding of the role these peptides play in Alzheimer’s and the state of therapeutics that target them. Contrary to previous theories, it is now understood that soluble amyloid beta and tau proteins are more neurotoxic than the insoluble aggregates they form. Treatments that target the individual biosynthetic pathways of these neurotoxic proteins have been ineffective. Thus, new therapies must overcome challenges associated with pharmacokinetics and clinical research design.
Advertisement
INTRODUCTION Alzheimer’s disease (AD) is a neurodegenerative condition resulting in ~3.5% of all human deaths — a statistic projected to triple by 2050 as a result of aging populations. 1-3 While it is typically characterized by severe memory loss, AD also results in linguistic, visuospatial, and cognitive deficits, primarily due to damage of the hippocampus. 4 AD commonly affects those greater than 65 years old. 5 Therapies have focused on inhibiting the accumulation of amyloid and tau proteins, but these treatments have been ineffective. 6-8 The objective of this review is to discuss the current understanding of the roles and mechanisms of key proteins and oligomers in the pathophysiology of AD, address the shortcomings of current treatment strategies, and offer insight into future research and therapeutic directions.
AMYLOID PROTEINS Amyloid beta (Aβ) peptides are among the most studied proteins implicated in the pathophysiology of AD. They form the basis of one of the most popular hypotheses regarding AD pathology, the amyloid cascade hypothesis, which suggests that Aβ proteins aggregate into plaques on neuronal membranes, leading to a series of pathological changes that ultimately result in the clinical symptoms of AD. 7 These proteins are produced through a series of biosynthetic secretory pathways beginning with the proteolytic cleavage of the amyloid precursor protein (APP), encoded by a highly conserved gene located on chromosome 21. 9,10 APP is an integral protein with a single transmembrane domain that is normally cleaved by α-secretase, an enzyme that releases the soluble extracellular domain of APP. 11 This pathway is non-amyloidogenic, as APP is cleaved at a residue contained within the Aβ primary sequence. 12 In AD, however, APP is instead cleaved extracellularly by β-secretase and in the transmembrane region by γ-secretase, an oligomeric enzyme complex composed primarily of presenilin proteins. 13,14 These presenilin proteins and the transmembrane APP cleavage for which they are responsible are essential in the development of AD. 15 The reason for this remains elusive. This abnormal pathway is generally understood to be the mechanism by which Aβ proteins are derived from APP. 4 Aβ exists in several forms, the most toxic of which contain 40 and 42 amino acids, known as Aβ40 and Aβ42, respectively. These forms are present in both human blood and cerebrospinal fluid (CSF). 16
Soluble Aβ oligomers form fibrils and plaques through a well-described process known as nucleation-dependent polymerization (Figure 1). Initially, individual monomers interact with each other through hydrogen bonding and Van der Waals forces, gradually forming larger polymers. Since this nucleation phase is thermodynamically unfavourable, it is the rate-limiting step in the polymerization process. After a nucleus of adequate size has formed, monomers can interact at several sites allowing a complete fibril to form rapidly. 17 The rate-limiting step can be overcome by adding alreadyformed nuclei, known as seeds, to a solution of Aβ monomers, thus significantly accelerating fibril polymerization. 18,19 These polymers grow continuously, eventually adhering to neuronal membranes in large, complex structures known as neuritic plaques.
Several mechanisms have been proposed to explain the neurotoxicity of Aβ. While it was once thought that plaques were the primary toxic species in AD, it has recently been demonstrated that soluble Aβ40 and Aβ42 oligomers are in fact more responsible for cell death than their aggregated counterparts. 20,21 These soluble Aβ species exhibit a secondary structure consisting of either two hydrophobic β-pleated sheet regions or the formation of a β-barrel, which cause cell death by inserting into neuronal membranes and destabilizing them. 22,23 Additionally, it has been proposed that Aβ oxidatively damages membrane lipids, prematurely triggers neuronal apoptotic mechanisms, and opens calcium channels in neuronal membranes leading to electrical imbalances and subsequently cell death. 24-26 Ultimately, fewer neurons result in fewer synapses and decreased cognitive function.
In summary, Aβ is a class of proteins produced by proteolytic cleavage of the transmembrane APP by β- and γ-secretases. Through the formation of fibrils, plaques, and soluble oligomers, Aβ plays a major role in neuronal cell death and is one of several proteins implicated in AD.
TAU PROTEINS In addition to Aβ, tau proteins have been significantly studied and play a more important role in AD. Tau describes a family of six protein isoforms that result from alternative splicing of the MAPT gene located on human chromosome 17. 27,28 These isoforms may vary, with an additional 29- or 54-amino acid sequence found at the N-terminus of certain isoforms implicated in AD. 27
1. Mahanani WR (2016). Global health estimates 2016: deaths by cause, age, sex, by country and by Region, 2000- 2016. World Health Organization. Dataset, Excel Sheet. Available from: https://www.who.int/healthinfo/global_bur- den_disease/GHE2016_Deaths_WBInc_2000_2016.xl [accessed 2019 Dec 23] 2. Hebert LE, Weuve J, Scherr PA, Evans DA. Alzheimer dis- ease in the United States (2010–2050) estimated using the 2010 census. Neurology. 2013 May 7;80(19):1778. Available from: doi:10.1212/WNL.0b013e31828726f5. 3. Brookmeyer R, Gray S, Kawas C. Projections of Alzhei- mer’s disease in the United States and the public health impact of delaying disease onset. Am J Public Health. 1998;88(9):1337–42. Available from: doi:10.2105/ AJPH.88.9.1337. 4. Jameson JL, Fauci AS, Kasper DL, Hauser SL, Longo DL, Lo- scalzo J. Harrison’s principles of internal medicine. 20th ed. New York, NY: McGraw-Hill Education; 2018. 4048. 5. Kawas C, Gray S, Brookmeyer R, Fozard J, Zonderman A. Age-specific incidence rates of Alzheimer’s disease. Neu- rology. 2000;54(11):2072. Available from: doi:10.1212/ WNL.54.11.2072. 6. Ballatore C, Lee VM-Y, Trojanowski JQ. Tau-mediated neuro- degeneration in Alzheimer’s disease and related disorders. Nat Rev Neurosci. 2007;8(9):663. Available from: doi:doi. org/10.1038/nrn2194. 7. Hardy JA, Higgins GA. Alzheimer’s disease: the amyloid cascade hypothesis. Science. 1992;256(5054):184–6. Available from: https://go.galegroup.com/ps/anony- mous?id=GALE%7CA12207965&sid=googleSchol- ar&v=2.1&it=r&linkaccess=abs&issn=00368075&p=HR- CA&sw=w [cited 2019 Dec 23]. 8. Haas C. Strategies, development, and pitfalls of thera- peutic options for Alzheimer’s disease. J Alzheimers Dis. 2012;28(2):241–81. Available from: doi.org/ 10.3233/ JAD-2011-110986. 9. Kang J, Lemaire H-G, Unterbeck A, Salbaum JM, Masters CL, Grzeschik K-H, et al. The precursor of Alzheimer’s dis- ease amyloid A4 protein resembles a cell-surface receptor. Nature. 1987;325(6106):733–6. Available from: doi. org/10.1038/325733a0. 10. Goldgaber D, Lerman MI, McBride OW, Saffiotti U, Gaj- dusek DC. Characterization and chromosomal localization of a cDNA encoding brain amyloid of Alzheimer’s disease. Science. 1987;235(4791):877–80. Available from: doi. org/10.1126/science.3810169. 11. Weidemann A, König G, Bunke D, Fischer P, Salbaum JM, Masters CL, et al. Identification, biogenesis, and local- ization of precursors of Alzheimer’s disease A4 amyloid protein. Cell. 1989;57(1):115–26. Available from: doi. org/10.1016/0092-8674(89)90177-3. 12. Esch F, Keim P, Beattie E, Blacher R, Culwell A, Oltersdorf T, et al. Cleavage of amyloid beta peptide during constitutive pro- cessing of its precursor. Science. 1990;248(4959):1122. Available from: doi.org/10.1126/science.2111583. 13. van Maanen EMT, van Steeg TJ, Ahsman MJ, Michener MS, Savage MJ, Kennedy ME, et al. Extending a systems mod- el of the APP Pathway: separation of β- and γ-secretase sequential cleavage steps of APP. J Pharmacol Exp Ther. 2018;365(3):507. Available from: doi.org/10.1124/ jpet.117.244699. 14. Herreman A, Serneels L, Annaert W, Collen D, Schoonjans L, De Strooper B. Total inactivation of γ–secretase activity in presenilin-deficient embryonic stem cells. Nat. Cell Biol. 2000;2(7):461–2. Available from: doi. org/10.1038/35017105. 15. Zhang Z, Nadeau P, Song W, Donoviel D, Yuan M, Bernstein A, et al. Presenilins are required for γ-secretase cleavage of β-APP and transmembrane cleavage of Notch-1. Na- ture Cell Biology. 2000;2(7):463–5. Available from: doi. org/10.1038/35017108. 16. Seubert P, Vigo-Pelfrey C, Esch F, Lee M, Dovey H, Davis D, et al. Isolation and quantification of soluble Alzheimer’s β-pep- tide from biological fluids. Nature. 1992;359(6393):325–7. Available from: doi.org/10.1038/359325a0. 17. Chothia C, Janin J. Principles of protein–protein recogni- tion. Nature. 1975;256(5520):705–8. Available from: doi. org/10.1038/256705a0. 18. Jarrett JT, Berger EP, Lansbury PT. The carboxy terminus of the beta-amyloid protein is critical for the seeding of amyloid formation: implications for the pathogenesis of Alzheimer’s disease. Biochemistry. 1993;32(18):4693–7. Available from: doi.org/10.1021/bi00069a001. 19. Niewold TA, Hol P, Lutz E, Gruys E. Enhancement of amyloid induction by amyloid fibril fragments in hamster. Lab Invest. 1987;56(5):544–9. Available from: https://europepmc. org/article/med/3553738. [cited 2019 Dec 22]. 20. Larson ME, Lesné SE. Soluble Aβ oligomer production and toxicity. J Neurochem. 2012;120(s1):125–39. Available from: doi.org/10.1111/j.1471-4159.2011.07478.x. 21. Viola KL, Klein WL. Amyloid β oligomers in Alzheimer’s disease pathogenesis, treatment, and diagnosis. Acta Neuropathol. 2015;129(2):183–206. Available from: doi. org/10.1007/s00401-015-1386-3. 22. Ahmed RT, Akcan M, Khondker A, Rheinstadter M, Boz- elli Jr J, Epand RM, et al. Atomic resolution map of the soluble amyloid beta assembly toxic surfaces. Chem Sci. 2019;10:6072-82. Available from: doi.org/10.1039/ C9SC01331H. 23. Ngo ST, Nguyen PH, Derreumaux P. Impact of A2T and D23N Mutations on Tetrameric Aβ42 Barrel within a di- palmitoylphosphatidylcholine lipid bilayer membrane by replica exchange molecular dynamics. J Phys Chem B. 2020;124(7):1175-82. Available from: doi:10.1021/acs. jpcb.9b11881. 24. Koppaka V, Axelsen PH. Accelerated accumulation of am- yloid β proteins on oxidatively damaged lipid membranes. Biochemistry. 2000;39(32):10011–6. Available from: doi. org/10.1021/bi000619d. 25. Demeester N, Baier G, Enzinger C, Goethals M, Vande- kerckhove J, Rosseneu M, et al. Apoptosis induced in neuronal cells by C-terminal amyloid ß-fragments is cor- related with their aggregation properties in phospholipid membranes. Mol. 2000;17(4):219–28. Available from: doi. org/10.1080/09687680010015838. 26. Kawahara M, Kuroda Y, Arispe N, Rojas E. Alzheimer’s β-amyloid, human islet amylin, and prion protein fragment evoke intracellular free calcium elevations by a common mechanism in a hypothalamic GnRH neuronal cell line. J Biol Chem. 2000;275(19):14077–83. Available from: doi. org/10.1074/jbc.275.19.1407. 27. Himmler A. Structure of the bovine tau gene: alternative- ly spliced transcripts generate a protein family. Mol Cell Biol. 1989;9(4):1389. Available from: doi.org/10.1128/ MCB.9.4.1389. 28. Neve RL, Harris P, Kosik KS, Kurnit DM, Donlon TA. Iden- tification of cDNA clones for the human microtubule-as- sociated protein tau and chromosomal localization of the genes for tau and microtubule-associated protein 2. Mol Brain Res. 1986;1(3):271–80. Available from: doi. org/10.1016/0169-328X(86)90033-1. 29. Schweers O, Schönbrunn-Hanebeck E, Marx A, Mandelkow E. Structural studies of tau protein and Alzheimer paired he- lical filaments show no evidence for beta-structure. J Biol Chem. 1994;269(39):24290–7. Available from: http:// www.jbc.org/content/269/39/24290.short. 30. Mukrasch MD, Bibow S, Korukottu J, Jeganathan S, Bi- ernat J, Griesinger C, et al. Structural polymorphism of 441-residue tau at single residue resolution. PLoS Biol. 2009;7(2):e1000034. Available from: doi.org/10.1371/ journal.pbio.1000034. 31. Weingarten MD, Lockwood AH, Hwo SY, Kirschner MW. A protein factor essential for microtubule assembly. Proc Natl Acad Sci USA. 1975;72(5):1858. Available from: doi. org/10.1073/pnas.72.5.1858. 32. Hu X, Viesselmann C, Nam S, Merriam E, Dent EW. Activi- ty-dependent dynamic microtubule invasion of dendritic spines. J Neurosci Res. 2008;28(49):13094. Available from: doi.org/10.1523/JNEUROSCI.3074-08.2008. 33. Margolis RL, Wilson L. Opposite end assembly and dis- assembly of microtubules at steady state in vitro. Cell. 1978;13(1):1–8. Available from: doi.org/10.1016/0092- 8674(78)90132-0. 34. Cleveland DW, Hwo S-Y, Kirschner MW. Purification of tau, a microtubule-associated protein that induc- es assembly of microtubules from purified tubulin. J Mol Biol. 1977;116(2):207–25. Available from: doi. org/10.1016/0022-2836(77)90213-3. 35. Drechsel DN, Hyman AA, Cobb MH, Kirschner MW. Mod- ulation of the dynamic instability of tubulin assembly by the microtubule-associated protein tau. Mol Biol Cell. 1992;3(10):1141–54. Available from: doi.org/10.1091/ mbc.3.10.1141.
Due to its mostly hydrophilic nature, tau does not exhibit a compact secondary structure similar to many other proteins and is naturally unfolded. 29,30 These physical properties make tau unique among proteins and are important to consider when developing a biochemical understanding of AD.
Unlike Aβ, the role of tau proteins in healthy physiology is well understood. These proteins are essential for the stabilization of microtubules in neurons, which facilitate intracellular transport and neurotransmission. 31,32 Microtubules are polymers of the dimeric protein tubulin, and are regulated through two distinct phases: a shrinking phase known as catastrophe, whereby tubulin subunits are removed from one end, and a growth phase known as rescue, whereby tubulin subunits are added to the opposite end. 33 Tau slows the rate of catastrophe and accelerates rescue by binding to individual tubulin dimers and inducing conformational changes that strengthen interactions between the various tubulin units. 34-36 This allows microtubules to grow to considerable lengths, which is particularly important in neurons, where microtubules must facilitate the transport of vesicles over great distances down the axon.
The role of tau in AD has been widely studied and involves several different signalling pathways. Under normal conditions, tau and other microtubule-associated proteins are phosphorylated to decrease their affinity for tubulin, thereby detaching them from microtubules. This is advantageous in certain circumstances since microtubuleassociated proteins often serve as obstacles for motor proteins as they transport cargo over microtubules. 37 Tau in AD is hyperphosphorylated at several sites, which excessively decreases its affinity for tubulin and limits its ability to stabilize microtubules. 38-40 The shift of this balance towards the hyperphosphorylated state has been observed in vivo, suggesting that this process in AD may be the result of an imbalance between phosphatases and kinases. 41,42 Tau in AD is also subject to cleavage by several proteases which releases protein fragments that may further decrease the ability of tau to stabilize microtubules, resulting in neuronal death. 43,44
Both hyperphosphorylation and proteolytic cleavage of tau cause it to be detached from microtubules and increase its propensity to aggregate into insoluble structures known as neurofibrillary tangles (NFTs). 45 NFT formation is similar to Aβ aggregation in several ways: it follows a nucleationdependent mechanism that can be accelerated through the addition of seeds, and it is likely that these large aggregates are less neurotoxic than the soluble oligomers and monomers from which they are formed. 46-49
Tau, like Aβ, is an important protein implicated in the pathophysiology of AD due to its ability to form aggregates via hyperphosphorylation. However, there are significant differences between NFT and Aβ processes, such as the fact that NFTs are cytosolic while Aβ aggregates are extracellular. 50 The exact mechanisms of tau toxicity are unclear, although it is likely that hyperphosphorylated and aggregated tau causes neuronal death by failing to support microtubules. 51
FIGURE 1. A simple nucleation-dependent polymerization mechanism.
Kn
LAG TIME NUCLEUS
kg
kg
Kg
SEEDED GROWTH
Monomers (depicted as violet spheres) are in equilibrium with small oligomers in the thermodynamically unfavourable nucleation phase shown on the left (K n <<1). When a nucleus of adequate size forms, monomers can interact at various sites and the process becomes favourable (K g >>1), leading to a period of rapid growth, shown on the right. 17 The rate limiting step can be skipped entirely by adding exogenous nuclei to a solution of monomers. 18,19
TREATMENT APPROACHES Several therapies have been developed that attempt to disrupt the protein pathways involved in AD (Table 1). The amyloidogenic pathway is frequently the target of such therapies, many of which inhibit β- and γ-secretase. Initial studies on AD in β-secretase knockout mice models showed significantly reduced blood and CSF Aβ concentrations. However, neuronal myelination in these mice was significantly impaired, suggesting that β-secretase plays a role in healthy physiology. 52,53 In spite of this, pharmacological β-secretase inhibitors have been developed, which have proven promising in vitro but do not translate to clinical benefits. 54-57 Similarly, γ-secretase inhibitors have demonstrated significant in vitro reduction of Aβ, but have shown no clinical benefit despite decreasing plasma Aβ concentration. 58-61 This points to a more complex role of Aβ in AD that is not yet fully understood.
Some drugs adopt the opposite approach; rather than inhibit β- and γ-secretases, they stimulate α-secretase. As described above, APP can be cleaved through an amyloidogenic pathway that involves β- and γ-secretase or a non-amyloidogenic pathway that involves α-secretase. 12,13 By activating protein kinase C, drugs such as bryostatin and semagacestat enhance α-secretase activity, leading to decreased Aβ production in vitro. 62,63 Early clinical trials demonstrate significant cognitive improvement and no adverse side effects, but also report potential bioaccumulation at higher doses. 64 Therefore, α-secretase stimulation is a promising avenue of research and warrants further exploration. Tau, however, has not been a therapeutic target in AD, likely because research focused on Aβ for several decades without considering the possibility that tau may be a key driver. The development and testing of novel treatments for AD currently face many challenges. Firstly, any drug that aims to treat AD must cross the blood-brain barrier (BBB), a tight membrane of epithelial cells that cover much of the brain and spinal cord. 65 A great deal of research is focused on bypassing the BBB, employing strategies such as encapsulating drugs in modified red blood cells or creating openings with ultrasound. 66,67 Additionally, given that current AD diagnosis is based primarily on cognitive tests and that AD progresses slowly over an extended period, designing effective clinical trials is difficult. 68 Challenges associated with drug delivery, AD diagnosis, and clinical research design combined with the complexity of AD pathophysiology make drug discovery challenging.
CONCLUSION Tau, Aβ, APP, and the various secretases, proteases, and kinases that regulate their synthetic pathways have all been investigated as potential drug targets in AD, with limited success. The chemical processes of this disease, such as the nucleation-dependent polymerization of both tau and Aβ proteins, the destabilizing effects these processes have on neuronal membrane, and their related neurotoxicity, are crucial concepts in understanding AD. Novel therapeutics will need to address the relationships between these complex pathways, as well as overcome difficulties associated with drug delivery, clinical research, and the inherent complexity of AD pathophysiology.
TABLE 1. Summary of AD therapies targeting protein pathways Treatment Mechanism Trial Stage Reference
LY2886721 BACE1 inhibitor Phase I: Safety and efficacy May 2015 54
Verubecestat β-secretase inhibitor Phase I: Safety and efficacy Egan 2019 56
Lanabecestat β-secretase inhibitor Phase I: Safety and efficacy Wessels 2019 57
Semagacestat γ-secretase inhibitor Phase III: Superiority Doody 2013 59
LY450139 γ-secretase inhibitor Phase II: Tolerance Fleischer 2008 60
LY450139 γ-secretase inhibitor Phase II: Tolerance Siemers 2006 61
PEER REVIEWED BY: DR. JOHN PAUL OLIVERIA
Dr. Oliveria is an Assistant Clinical Professor at McMaster University and a postdoctoral scholar at Stanford University, as well as a member of the McMaster Institute for Research on Aging. He studies the molecular mechanisms of Alzheimer’s disease and the immunology underlying allergies.
36. Panda D, Goode BL, Feinstein SC, Wilson L. Kinetic sta- bilization of microtubule dynamics at steady state by tau and microtubule-binding domains of tau. Biochemistry. 1995;34(35):11117–27. Available from: https://pubs.acs. org/doi/pdf/10.1021/bi00035a017. 37. Mandelkow E-M, Thies E, Trinczek B, Biernat J, Mandelkow E. MARK/PAR1 kinase is a regulator of microtubule-dependent transport in axons. J Cell Biol. 2004;167(1):99–110. Avail- able from: doi.org/10.1083/jcb.200401085. 38. Köpke E, Tung Y-C, Shaikh S, Alonso A del C, Iqbal K, Grund- ke-Iqbal I. Microtubule-associated protein tau. Abnormal phosphorylation of a non-paired helical filament pool in Alzheimer disease. J Biol Chem. 1993;268(32):24374–84. Available from: http://www.jbc.org/con- tent/268/32/24374.short. 39. Brandt R, Lee G, Teplow DB, Shalloway D, Abdel-Ghany M. Differential effect of phosphorylation and substrate modula- tion on tau’s ability to promote microtubule growth and nu- cleation. J Biol Chem. 1994;269(16):11776–82. Available from: http://www.jbc.org/content/269/16/11776.short. [cited 2019 Dec 11]. 40. Illenberger S, Zheng-Fischhöfer Q, Preuss U, Stamer K, Baumann K, Trinczek B, et al. The endogenous and cell cycle-dependent phosphorylation of tau protein in living cells: implications for Alzheimer’s disease. Mol Biol Cell. 1998;9(6):1495–512. Available from: doi.org/10.1091/ mbc.9.6.1495. 41. Härtig W, Stieler J, Boerema AS, Wolf J, Schmidt U, Weißfuß J, et al. Hibernation model of tau phosphorylation in ham- sters: selective vulnerability of cholinergic basal forebrain neurons – implications for Alzheimer’s disease. Eur J Neuro- sci. 2007;25(1):69–80. Available from: doi.org/10.1111/ j.1460-9568.2006.05250.x. 42. Planel E, Richter KEG, Nolan CE, Finley JE, Liu L, Wen Y, et al. Anesthesia leads to tau hyperphosphorylation through in- hibition of phosphatase activity by hypothermia. J Neurosci Res. 2007;27(12):3090. Available from: doi.org/10.1523/ JNEUROSCI.4854-06.2007. 43. Horowitz PM, Patterson KR, Guillozet-Bongaarts AL, Reyn- olds MR, Carroll CA, Weintraub ST, et al. Early N-terminal changes and caspase-6 cleavage of tau in Alzheimer’s dis- ease. J Neurosci Res. 2004;24(36):7895. Available from: doi.org/10.1523/JNEUROSCI.1988-04.2004. 44. Guo T, Dakkak D, Rodriguez-Martin T, Noble W, Hanger DP. A pathogenic tau fragment compromises microtubules, dis- rupts insulin signaling and induces the unfolded protein re- sponse. Acta Neuropathol Commun. 2019;7(1):2. Available from: doi.org/10.1186/s40478-018-0651-9. 45. Mandelkow E-M, Mandelkow E. Biochemistry and cell biology of tau protein in neurofibrillary degeneration. Cold Spring Harb Perspect Med. 2012;2(7):a006247. Available from: doi.org/10.1101/cshperspect.a006247. 46. Congdon EE, Kim S, Bonchak J, Songrug T, Matzavinos A, Kuret J. Nucleation-dependent tau filament formation the importance of dimerization and an estimation of elementary rate constants. J Biol Chem. 2008;283(20):13806–16. Available from: doi.org/10.1074/jbc.M800247200. 47. von Bergen M, Friedhoff P, Biernat J, Heberle J, Mandelkow E-M, Mandelkow E. Assembly of τ protein into Alzheimer paired helical filaments depends on a local sequence motif (306VQIVYK311) forming β structure. Pc Natl Acad Sci US. 2000;97(10):5129. Available from: doi.org/10.1073/ pnas.97.10.5129. 48. Cowan C, Mudher A. Are tau aggregates toxic or protective in tauopathies? Front Neurol. 2013;4:114. Available from: doi. org/10.3389/fneur.2013.00114. 49. Lasagna-Reeves CA, Castillo-Carranza DL, Sengupta U, Sarmiento J, Troncoso J, Jackson GR, et al. Identification of oligomers at early stages of tau aggregation in Alzheimer’s disease. FASEB J. 2012;26(5):1946–59. Available from: doi.org/10.1096/fj.11-199851. 50. Bancher C, Brunner C, Lassmann H, Budka H, Jellinger K, Wiche G, et al. Accumulation of abnormally phosphorylated τ precedes the formation of neurofibrillary tangles in Alz- heimer’s disease. Brain Res. 1989;477(1):90–9. Available from: doi.org/10.1016/0006-8993(89)91396-6. 51. Lei P, Ayton S, Moon S, Zhang Q, Volitakis I, Finkelstein DI, et al. Motor and cognitive deficits in aged tau knockout mice in two background strains. Mol Neurodegener. 2014;9(1):29. Available from: doi.org/10.1186/1750-1326-9-29. 52. Hu X, Hicks CW, He W, Wong P, Macklin WB, Trapp BD, et al. Bace1 modulates myelination in the central and peripheral nervous system. Nat Neurosci. 2006;9(12):1520–5. Avail- able from: doi.org/10.1038/nn1797. 53. Willem M, Garratt AN, Novak B, Citron M, Kaufmann S, Ritt- ger A, et al. Control of peripheral nerve myelination by the ß-Secretase BACE1. Science. 2006;314(5799):664. Avail- able from: doi.org/10.1126/science.1132341. 54. May PC, Willis BA, Lowe SL, Dean RA, Monk SA, Cocke PJ, et al. The potent BACE1 inhibitor LY2886721 elicits robust central Aβ pharmacodynamic responses in mice, dogs, and humans. J Neurosci Res. 2015;35(3):1199. Available from: doi.org/10.1523/JNEUROSCI.4129-14.2015. 55. May PC, Dean RA, Lowe SL, Martenyi F, Sheehan SM, Boggs LN, et al. Robust central reduction of amyloid-β in humans with an orally available, non-peptidic β-Secretase inhibitor. J Neurosci Res. 2011;31(46):16507. Available from: doi. org/10.1523/JNEUROSCI.3647-11.2011. 56. Egan MF, Kost J, Voss T, Mukai Y, Aisen PS, Cummings JL, et al. Randomized trial of verubecestat for prodromal Alz- heimer’s disease. N Engl J Med. 2019;380(15):1408–20. Available from: doi.org/10.1056/NEJMoa1812840. 57. Wessels AM, Tariot PN, Zimmer JA, Selzler KJ, Bragg SM, Andersen SW, et al. Efficacy and safety of lanabecestat for treatment of early and mild Alzheimer disease: the AMARANTH and DAYBREAK-ALZ randomized clinical trials. JAMA Neurol. 2019; Available from: https://doi. org/10.1001/jamaneurol.2019.3988. 58. Elvang AB, Volbracht C, Pedersen LØ, Jensen KG, Karlsson J, Larsen SA, et al. Differential effects of γ-secretase and BACE1 inhibition on brain Aβ levels in vitro and in vivo. J Neurochem. 2009;110(5):1377–87. Available from: doi. org/10.1111/j.1471-4159.2009.06215.x. 59. Doody RS, Raman R, Farlow M, Iwatsubo T, Vellas B, Joffe S, et al. A phase 3 trial of Semagacestat for treatment of Alzheimer’s disease. N Engl J Med. 2013;369(4):341–50. Available from: doi.org/10.1056/NEJMoa1210951. 60. Fleisher AS, Raman R, Siemers ER, Becerra L, Clark CM, Dean RA, et al. Phase 2 safety trial targeting amyloid β production with a γ-secretase inhibitor in Alzheimer disease. Arch Neurol. 2008;65(8):1031–8. Available from: doi. org/10.1001/archneur.65.8.1031. 61. Siemers ER, Quinn JF, Kaye J, Farlow MR, Porsteinsson A, Tariot P, et al. Effects of a γ-secretase inhibitor in a random- ized study of patients with Alzheimer disease. Neurology. 2006;66(4):602. Available from: doi.org/10.1212/01. WNL.0000198762.41312.E1. 62. Ibarreta D, Duchén M, Ma D, Qiao L, Kozikowski AP, Etche- berrigaray R. Benzolactam (BL) enhances sAPP secretion in fibroblasts and in PC12 cells. NeuroReport. 1999;10(5). Available from: https://journals.lww.com/neuroreport/Full- text/1999/04060/Benzolactam__BL__enhances_sAPP_ secretion_in.26.aspx [cited 2019 Dec 23]. 63. Garrido JL, GODOY JA, Alvarez A, Bronfman M, Inestrosa NC. Protein kinase C inhibits amyloid β peptide neurotox- icity by acting on members of the Wnt pathway. FASEB J. 2002;16(14):1982–4. Available from: doi.org/10.1096/ fj.02-0327fje. 64. Nelson TJ, Sun M-K, Lim C, Sen A, Khan T, Chirila FV, et al. Bryostatin effects on cognitive function and PKC in Alz- heimer’s disease phase IIa and expanded access trials. J Alzheimers Dis. 2017;58(2):521–35. Available from: doi:10.3233/JAD-170161. 65. Brunton LL, Hilal-Dandan R, Knollmann BC. Goodman & Gilman’s: the pharmacological basis of therapeutics. 13th edition. New York, NY: McGraw-Hill Education; 2017. 1808. 66. Himbert S, Blacker MJ, Kihm A, Pauli Q, Khondker A, Yang K, et al. Hybrid erythrocyte liposomes: functionalized red blood cell membranes for molecule encapsulation. Adv. Biosyst. 2020;1900185. Available from: doi.org/10.1002/ adbi.201900185. 67. Kinoshita M, McDannold N, Jolesz FA, Hynynen K. Targeted delivery of antibodies through the blood–brain barrier by MRI-guided focused ultrasound. Biochem Bioph Res Co. 2006;340(4):1085–90. Available from: doi.org/10.1016/j. bbrc.2005.12.112. 68. McKhann GM, Knopman DS, Chertkow H, Hyman BT, Jack CR, Kawas CH, et al. The diagnosis of dementia due to Alzhei- mer’s disease: recommendations from the National Institute on Aging-Alzheimer’s Association workgroups on diagnostic guidelines for Alzheimer’s disease. Alzheimers Dement. 2011;7(3):263–9. Available from: doi.org/10.1016/j. jalz.2011.03.005.
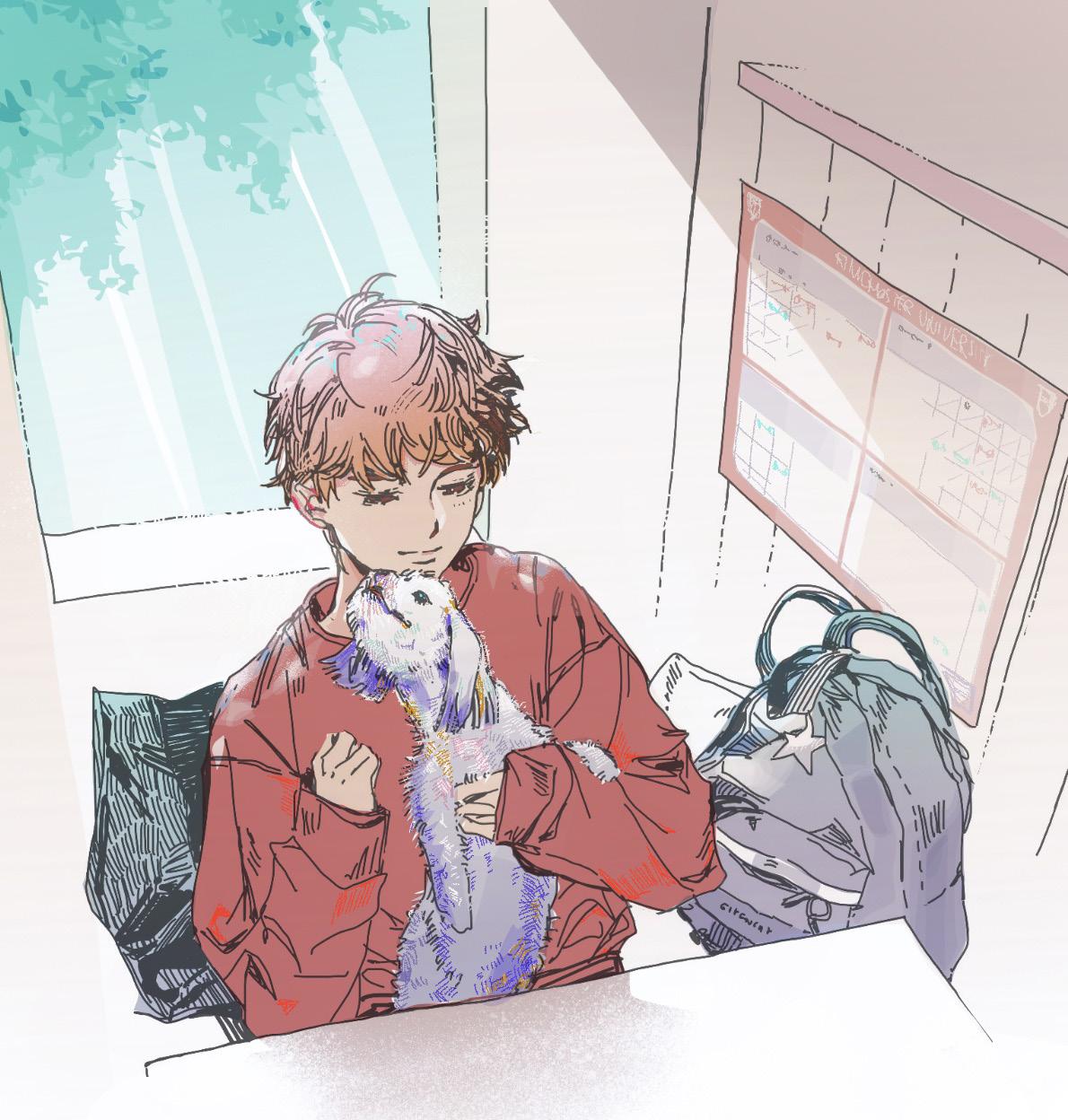
Animal-assisted activities: Justifying a mental health program at McMaster University CRITICAL REVIEW
Bachelor of Health Sciences (Honours) Class of 2020, McMaster University lit60@mcmaster.ca Bachelor of Science in Nursing Class of 2020, McMaster University cheunl6@mcmaster.ca TERRY LI LELAND CHEUNG doi:10.35493/medu.37.16
ABSTRACT For many students at McMaster University, adequate mental health is an elusive luxury. This mental health crisis has called for the inception of multiple programs to ensure the wellbeing of the student body. Animal-assisted activities (AAA), such as “PAWSS” or “Dogs at Mac,” are such types of programs that aim to relieve student stress through wholesome interaction with animals. Given that McMaster University has finite resources dedicated to improving student mental health, it is important to evaluate whether these programs are truly beneficial. This review has found literature to support McMaster’s use of AAA. The sessions offer the potential for mental health benefits at an extremely low cost. Additionally, positive university student perceptions toward animal-assisted interventions indicate a clear public interest in AAA events. The schedule of the animals used in AAA also upholds their welfare, which helps mitigate any ethical concerns regarding animal-assisted interventions. While the true efficacy of AAA has not yet been determined due to mixed research literature around the topic, no studies have identified detrimental effects resulting from positive humananimal interactions. This paucity suggests that there is very little risk associated with using animals in a therapeutic setting when one accounts for its potential benefit.
CONTEXTUALIZING MENTAL HEALTH AT MCMASTER Mental health issues often act as barriers in the lives of postsecondary students in Ontario. The Council of Ontario Universities reported that 65% of students experienced overwhelming anxiety in 2016, presenting a 7% increase from 2013. 1 Within this period, the Council also reported a 6% increase in depression, from 40% to 46%. 1 These increasing trends have spurred Ontario universities to prioritize addressing mental health issues and invest in a collection of initiatives to support student mental well-being. McMaster University has launched a number of programs to improve student mental health, including programs which use animals within a therapeutic context: Pups Advising within Social Sciences (PAWSS) and Dogs at Mac. 2,3 Both organizations utilize certified therapy dogs at informal, drop-in sessions around campus during which students can freely interact with the dogs. 2,3 These events are scheduled during exam season and other stressful periods of the school year, and aim to improve student mental health outcomes. 2,3 Attempts to define interventions that incorporate animals have been ongoing since the 1980s, with widely used definitions including animal-assisted therapy (AAT) and animal-assisted activities (AAA). 4 AAT utilizes animals to deliver individualized therapies led by a licensed professional, while AAAs are interventions involving animals that are often not individualized for a specific client. 3,4 Based on these definitions, the services offered by PAWSS and Dogs at Mac fall under the AAA classification. The purpose of this paper is to determine whether AAAs are an effective use of university resources for improving student mental health. To provide a holistic evaluation of AAA services, several factors will be examined: the mental health benefits associated with AAAs, perceptions towards animals in therapeutic settings, ethical considerations, and cost-effectiveness. Overall, it can be hypothesized that AAA use at the university is justifiable.
EVALUATING THE EFFECTIVENESS OF AAAS Research studies evaluating AAA-associated mental health benefits have produced mixed results. Brooks et al. conducted a systematic review of published and grey literature up until March 2017 and found 17 quantitative studies exploring the effects of human-animal interactions on mental health. 5 Some of the studies correlated animal companion interactions with reduced feelings of loneliness, depression, and anxiety. 5 Through actions such as petting and sharing close contact with animals, participants experienced improvements in depression and mood. 5 In contrast, other studies reported neutral effects; for example, depression was found to be as common in pet owners as in non-pet owners. 5 These mixed research findings may be due to several factors, as reported by Serpell et al. 6 Most studies that evaluated animal-assisted interventions had methodological flaws, including small sample sizes, poorly defined research questions, a lack of standardized measures, and non-randomized assignment of participants to interventions. 6 These methodological issues make it challenging to accurately evaluate the effectiveness of AAAs. While the findings were mixed, none of the studies included in the systematic review by Brooks et al. found positive humananimal interactions, such as petting, playing, and hugging, to be detrimental to mental health. 5 This paucity indicates there is minimal risk associated with using animals in a therapeutic setting. 5 However, it is important to acknowledge that the review evaluated the benefits of human-animal interactions in the context of animal ownership, and the weaker emotional bonds between students and animals would therefore likely result in diminished health benefits. 5 Nonetheless, the human-animal interactions during play at the McMaster AAA sessions should be similar to those between an animal and its owner, meaning that the minimal risk associated with using animals in Brook et al.’s therapeutic setting should also apply to these sessions. 5 Therefore, while findings made by Brooks et al. do not fully represent McMaster’s AAA services, they are promising in terms of support for the initiative. 5
MEDUCATOR | MARCH 2020 1. Council of Ontario Universities. Mental Health [Internet]. Ontario’s Universities. 2019 . Available from: https://ontari- osuniversities.ca/issues-priorities/stu- dent-supports [cited 2019 Dec 9]. 2. McMaster University. PAWSS [Internet]. McMaster Faculty of Social Sciences. 2019 . Available from: https://social- sciences.mcmaster.ca/pawss [cited 2019 Dec 9]. 3. McMaster University. Dogs at MAC. 2019. Available from: https://dogsatmac. mcmaster.ca [cited 2019 Dec 9]. 4. Jones M, Rice S, Cotton S. Incorporating animal-assisted therapy in mental health treatments for adolescents: A systematic review of canine assisted psychotherapy. PLOS ONE. 2019;14(1):e0210761. Available from: doi:10.1371/journal. pone.0210761. 5. Brooks H, Rushton K, Lovell K, Bee P, Walker L, Grant L et al. The power of support from companion animals for people living with mental health problems: A systematic review and narrative synthesis of the evidence. BMC Psychiatry. 2018;18(1). Available from: doi:10.1186/s12888- 018-1613-2. 6. Serpell J, McCune S, Gee N, Griffin J. Current challenges to research on ani- mal-assisted interventions. Appl Dev Sci. 2017;21(3):223-233. Available from: do i:10.1080/10888691.2016.1262775. 7. Irvine L. Sociology and anthrozoology: Symbolic interactionist contributions. Anthrozoös. 2012;25(Supplement 1):123-137. Available from: doi: https://doi.org/10.2752/17530371 2X13353430377174. 8. Fine AH, Mokhatab S. Handbook on ani- mal-assisted therapy: Foundations and guidelines for animal-assisted interven- tions. 4th ed. Cambridge: Academic Press; 2015. 21-40 p. 9. Beetz A, Uvnäs-Moberg K, Julius H, Kotrschal K. Psychosocial and psychophysiological effects of human-animal interactions: The possible role of oxytocin. Front Psychol. 2012;3. Available from: doi:10.3389/fpsyg.2012.00234. 10. Guéguen N, Ciccotti S. Domestic dogs as facilitators in social interaction: An evaluation of helping and courtship behaviors. Anthrozoös. 2008;21(4):339-349. Available from: doi:10.2752/175303708X371564. 11. Koller D, Goldman R. Distraction techniques for children undergoing procedures: A critical review of pediatric research. J Pediatr Nurs. 2012;27(6):652-681. Available from: doi:10.1016/j.pedn.2011.08.001. 12. Pritchard, PA. “Take a paws” with therapy dogs and make it a howling success. In: Sittler RJ, Rogerson TJ. (eds.) The Library Outreach Casebook. Chicago: Association of College and Research Libraries; 2018. p.119-128. 13. Zents C, Fisk A, Lauback C. Paws for intervention: Perceptions about the use of dogs in schools. J Ment Health. 2016;12(1):82-98. Available from: doi: 10.1080/15401383.2016.1189371. 14. Schoenfeld-Tacher R, Hellyer P, Cheung L, Kogan L. Public perceptions of service dogs, emotional support dogs, and therapy dogs. Int J Environ Res Public Health. 2017;14(6):642. Available from: doi:10.3390/ijerph14060642. 15. Glenk L. Current perspectives on therapy dog welfare in animal-assisted interven- tions. Animals. 2017;7(12):7. Available from: doi:10.3390/ani7020007.
THEORETICAL BENEFITS OF AAA Despite the uncertainties surround-ing AAA effectiveness, several theories have attempted to explain why human-animal interactions may benefit mental health. Numerous theories focus on how this interaction operates, including those which examine the relationship through a symbolic interactionist perspective or through the perspective of therapy animals. 7,8 However, this paper examines biopsychosocial theories that focus more on the mental health benefits associated with AAA. One theory is the “social catalyst effect,” where the presence of animals may indirectly stimulate positive social interactions and relationships with other humans. 6,9 Experiments by Guéguen and Ciccotti demonstrated that dogs may help facilitate social interactions as people tended to act more friendly to those who had dogs compared to those who did not (p < 0.005). 10 In the case of the AAA sessions at McMaster, the therapy animals may help facilitate positive social interactions between students attending the event. A second theory takes a more biological approach, as Beetz et al. have tried to explain the psychophysiological benefits of AAA by outlining how human-animal interactions release oxytocin. 9 This hormone is able to reduce epinephrine and norepinephrine levels while also decreasing depressive mood. 9 A third theory claims that animals can act as a distraction from negative mental states. 8 Koller et al. found that distracting images, sounds, and activities can be used to reduce anxiety and other forms of emotional distress. 11 Several preliminary studies have also suggested that animals may be intrinsically effective at attracting and holding human attention. 8 Most notably, human infants have been found to have a significant preference for images of animals compared to images of motor vehicles, toys, or other objects. 8,11 While all three theories provide reasonable explanations to justify the benefits of AAA for mental health, they have yet to be validated in clinical research studies.
JUSTIFICATIONS FOR AAA USE AT MCMASTER UNIVERSITY As no definitive conclusions can currently be drawn about the effectiveness of AAA, and no strongly substantiated theories support the mental health benefits of AAA, it may be difficult to justify utilizing these services at McMaster University. However, the AAA sessions offered by organizations such as PAWSS and Dogs at Mac have a high potential for benefit and are extremely costeffective. A paper by Pritchard described a “Take a Paws” therapy dog event that was introduced at a University of Guelph library to relieve exam stress. 12 The library partnered with the St. John Ambulance Therapy Dog program which limited costs of executing the event to those of parking, bottled water, and a package of puppy pads. 12 Health and safety services were provided by unpaid student volunteers from the University of Guelph’s First Response team. 12 The therapy animal sessions run by McMaster University closely mirror the events hosted at the University of Guelph. For example, partnerships with local organizations such as the Hamilton-Burlington Society for the Prevention of Cruelty to Animals (SPCA) allow for inexpensive access to therapy dogs. Furthermore, McMaster’s Emergency First Response Team (EFRT) is comprised of volunteers who are on campus to ensure the health and safety of students during AAA sessions. Therefore, the cost-efficient nature of running events by PAWSS and Dogs at Mac would counteract the uncertainty concerning the benefits and effectiveness of AAA.
There is also evidence that students generally accept the use of animals to improve mental health in an academic setting. A study by Zents et al. evaluated the efficacy of therapy animals in improving mental wellbeing within a school setting. 13 The study collected data regarding the perceptions of 196 students and 105 faculty members to determine attitudes towards four therapy dogs. 13 It was found that students strongly supported the use of therapy animals in schools. 13 However, the majority of the study participants were in the sixth to eighth and eleventh to twelfth grades. This major limitation prevents study conclusions about student perceptions from being completely applicable to McMaster’s demographic. A study by Schoenfeld-Tacher et al. used online surveys to collect data regarding perceptions of assistance animals held by individuals ranging from ages 18 to 45. 14 This demographic more closely simulates
the population at McMaster University. The study concluded that 56.3% of respondents agreed that therapy animals should be used if they had the potential to be helpful. 14 In the absence of research literature evaluating university student perceptions of AAA, the papers by Zents et al. and Schoenfeld-Tacher et al. provide insight into the positive perceptions of students and adults towards animal usage within therapeutic settings. It is not unreasonable to assume that these perceptions will be reflected in the university student population.
ETHICAL CONSIDERATIONS The final consideration for AAA use is whether McMaster University’s current use of animals in AAA sessions can be ethically justified. A paper by Glenk reviewed current literature that followed therapy animals’ welfare during animal-assisted interventions. 15 The review concluded that implementing animals into therapeutic environments was considered not particularly stressful for participating animals. 15 However, some isolated cases of teasing and mistreatment of visiting therapy animals were reported. 15 To avoid situations that would cause work-related strain and decrease animals’ quality of life, guidelines for animal use in therapeutic settings should be followed. 15 Current recommendations include monitoring for signs of fatigue, giving animals ample opportunities to rest, limiting the duration of therapy sessions to 60 minutes, and limiting the number of visits to 3 sessions per week. 15 It appears that the services offered by PAWSS and Dogs at Mac follow these guidelines as AAA sessions tend to be less than 60 minutes and only run a handful of times during the school year. Therefore, the welfare of the animals used in AAA is being upheld.
CONCLUSION Several considerations help justify AAA use at McMaster University. AAA sessions are extremely cost-effective as they offer the potential for mental health benefits at a very low cost. Additionally, the positive university student perceptions toward animal-assisted interventions suggest a clear demand for AAA events. By following a schedule which upholds the welfare of animals used in AAA, the use of these interventions can be deemed ethical and therefore morally acceptable. Finally, no studies have identified detrimental effects resulting from positive human-animal interactions, suggesting that there is very little risk associated with using animals in a therapeutic setting. While the factors outlined above support the use of AAA at McMaster, the true efficacy of AAA has not yet been determined, as demonstrated by mixed findings in the existing literature. To attain a more definitive conclusion on whether McMaster’s AAA sessions are justified, additional studies utilizing stronger research methodologies are needed. Until then, these wonderful animals will continue to ease the stress of McMaster students.
REVIEWED BY: DR. MARK NORMAN
Dr. Mark Norman is a postdoctoral fellow in the Department of Health, Aging & Society at McMaster University. He completed his PhD in the Faculty of Kinesiology and Physical Education at the University of Toronto. His dissertation examined sport and physical culture in Canadian federal prisons. His research considers the social significance of sport, physical culture, and recreation in carceral settings, touching on questions of social control, agency, and resistance in and through physical activity. He also studies the significance of alternative prison activities, such as yoga and animal-assisted interventions.
EDITED BY: ALBERT STANCESCU AND JAMES YU
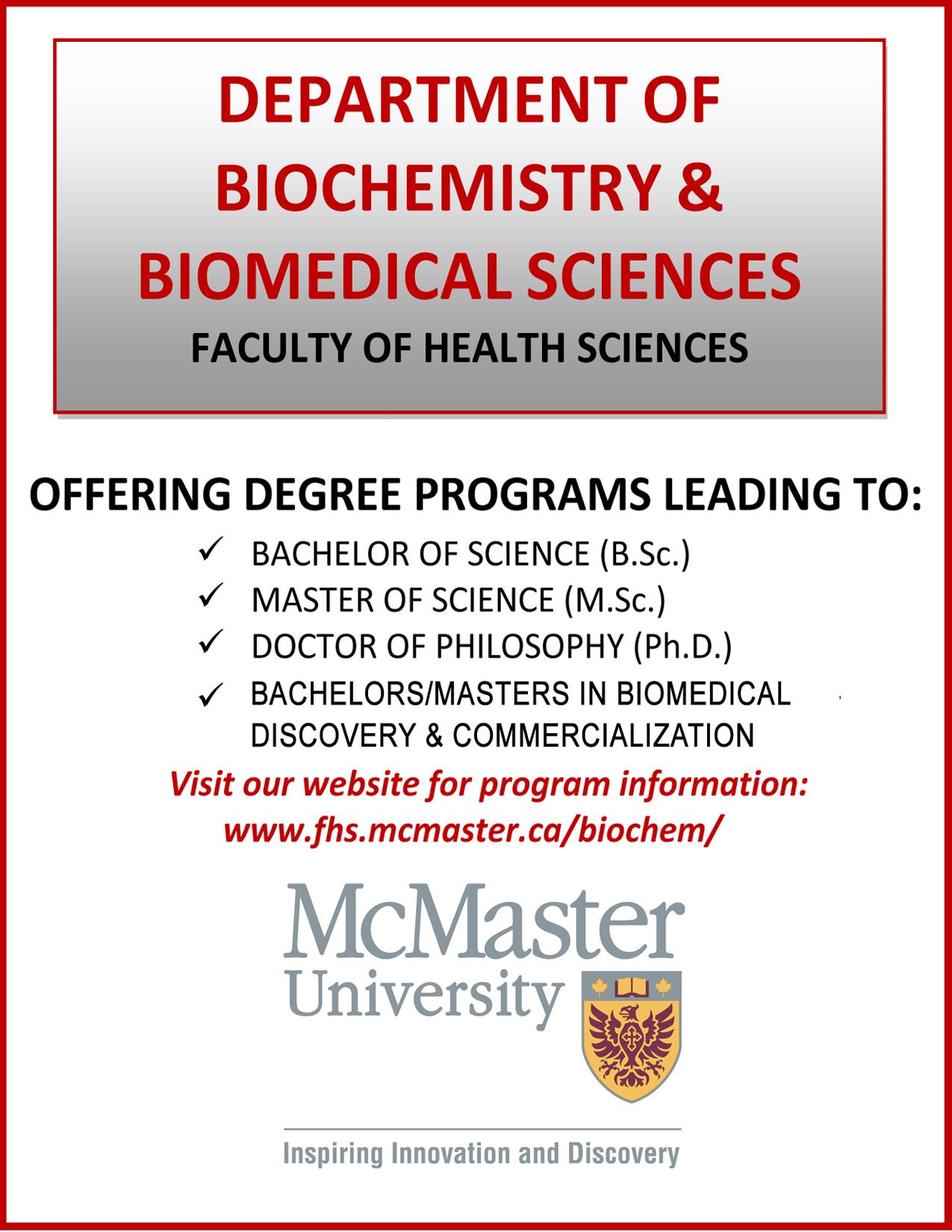