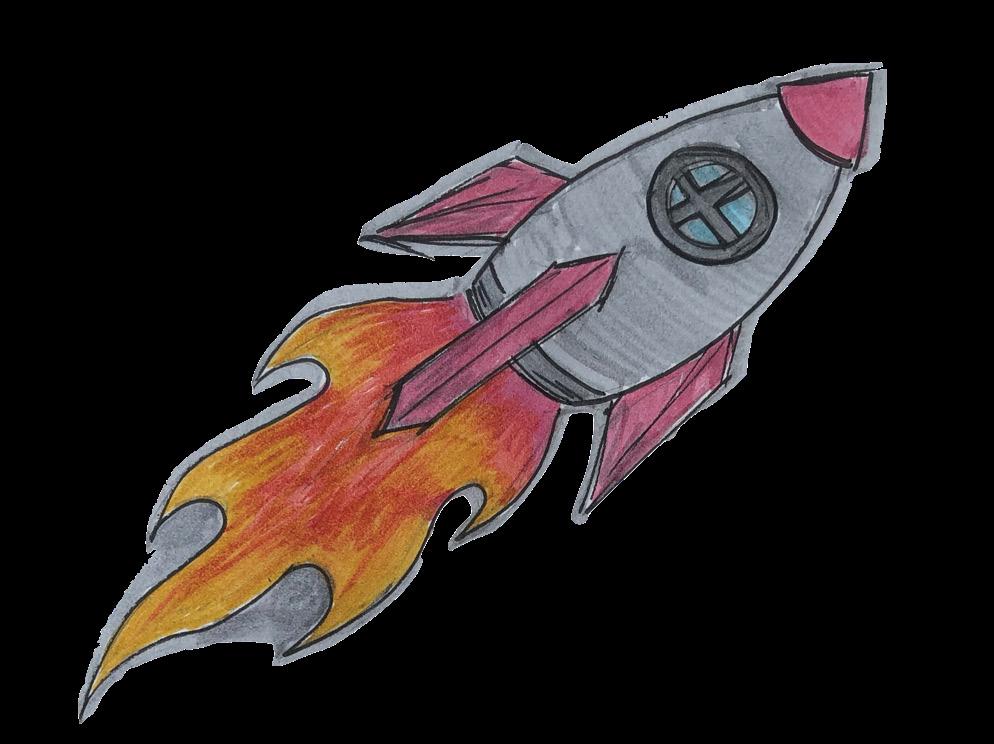
6 minute read
The Perseverance Rover’s Scientific Instruments
from PCR - Fall 2021
by Evan Xie (IV)
On February 18th, 2021, NASA’s Perseverance Rover successfully landed on Mars in the Jezero Crater. Following the Sojourner, Spirit, Opportunity, and Curiosity rovers, NASA’s previous Mars missions, Perseverance is tasked with four key missions: determine if life previously existed on Mars, identify the characteristics of past Martian climates, characterize the geology on Mars, and prepare for future human-led missions. With such difficult and important objectives to complete on a foreign planet, it should come as no surprise that this rover features some of humanity’s most advanced and revolutionary technology. Spending a great amount of time and $2.2 billion designing, prototyping, and building the rover, the scientists at NASA’s Jet Propulsion Lab (JPL) were able to pack an astounding seven scientific instruments, alongside a rock sampling system, a tiny helicopter, and more, within Perseverance’s 2260 pound, 10ft x 9ft x 7ft frame (Figure 1). Each scientific instrument combines different technologies to perform their respective experiments on Mars, which will hopefully broaden our understanding of the Red Planet.
First, Mastcam-Z is Perseverance’s intricate camera system that will capture pictures of the Martian surface and atmosphere while also helping scientists select rocks to core and study. Mastcam-Z is an upgrade from Curiosity’s camera system in almost every aspect: each individual camera can shoot 20-megapixel colored images, rather than 1-megapixel black and white images, contains powerful zoom capabilities to study smaller objects, and has a wider field of view providing Mastcam-Z with a full 360-degree perspective. With these features, Perseverance can photograph and record different terrain features, rocks, soil, and Martian phenomena (dust storms, cloud motions, etc), providing scientists with invaluable visuals to further their understanding of Mars.
Next, the Mars Environmental Dynamics Analyzer (MEDA) is a group of sensors responsible for measuring different aspects of the Martian environment —namely wind speed, temperature, humidity, dust particle size, and radiation levels. This data will be crucial for future manned missions, as weather predictions will protect astronauts in the dangerous Martian environment and weather. For example, intense dust storms can reduce the amount of sunlight reaching solar panels, decreasing energy production and causing equipment shut-downs, just as the Spirit and Opportunity rovers did. Being warned beforehand would allow astronauts to conserve some
Rocket by Ava Khan (III)
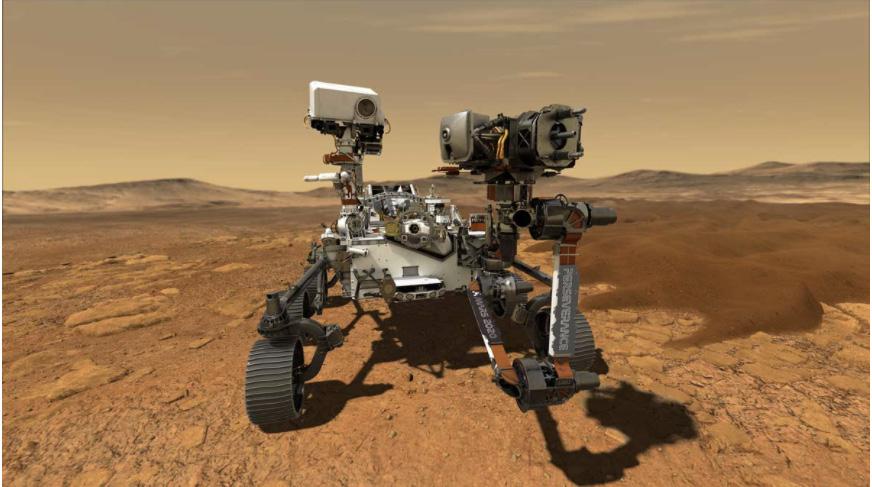
Figure 1: Image of the Perseverance Rover from the front
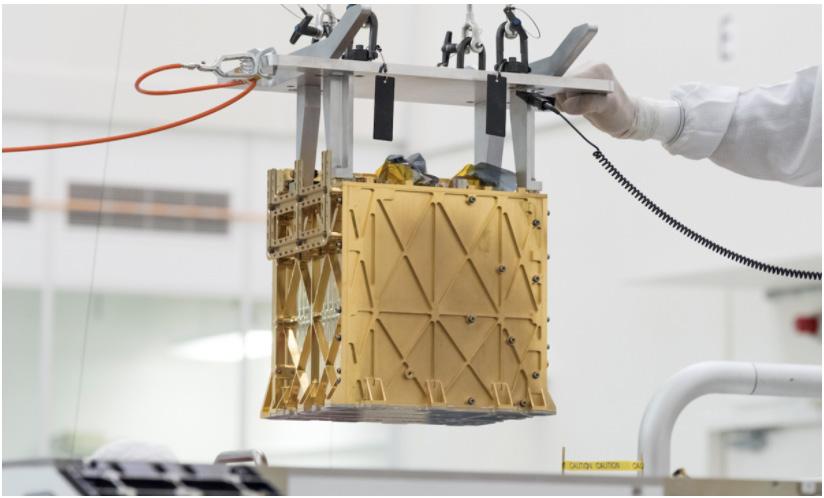
Figure 2: JPL scientists installing MOXIE (car battery sized) in the rover
energy and protect their equipment. Additionally, measurements of radiation levels would allow scientists to better understand signs of past life. As stronger solar energetic particles (SEPs) and galactic cosmic rays (GCRs), two sources of radiation in space, are capable of penetrating the Martian soil, any signs of life near the Martian surface could have been destroyed by gamma rays. If this is the case, scientists would have to collect samples from deeper in the Martian surface to determine if the planet ever housed living organisms. Possibly the most intriguing instrument of the seven, the Mars Oxygen In-Situ Resource Utilization Experiment (MOXIE) is a device theoretically capable of generating oxygen from Mars’s 95% carbon dioxide concentrated atmosphere (Figure 2). Using one-third of the rover’s power, MOXIE will use heat and electricity to split carbon dioxide (CO2) molecules into carbon monoxide (CO) and oxygen (O) molecules. Despite this device’s high energy consumption, it is only capable of producing 6 to 10 grams of oxygen per hour, which is not sufficient to support even a single human. Thus, this technology is currently only in a testing phase and much larger versions of MOXIE will be used for any manned missions. The Planetary Instrument for X-ray Lithochemistry (PIXL) uses a high-resolution camera and an X-ray fluorescence spectrometer to identify the small-scale composition of Martian materials. When PIXL shines a narrow, focused X-ray beam onto a tiny spot of rock or soil, the object emits a fluorescent X-ray. This can then be analyzed to determine the object’s elemental composition since each element produces its own unique fluorescent X-ray. With this technology, PIXL will be able to identify 26 different elements, including sodium, sulfur, and iron, allowing it to potentially identify signs of past life. The fifth instrument is the Radar Imager for Mars’ Subsurface Experiment (RIMFAX), a ground-penetrating radar (GPR) that can detect and map underground rock layers, water, and ice on Mars. All GPRs work in a similar fashion, sending a series of tiny electrical pulses into the ground and then recording the strength and return time of the reflected signal. After numerous pulses are released in a given area, the collected data can be input into special software that produces depth slices, or horizontal cross-sections, at specific depths underground. In the case of RIMFAX, the emitted electrical pulses vary from 150 MHz to 1.2 GHz, enabling a wide variety of scans; higher-frequency pulses can detect smaller targets but cannot penetrate as deep while the opposite is true for lower frequency pulses. In the end, RIMFAX is capable of scanning up to and potentially even past depths of 10m.
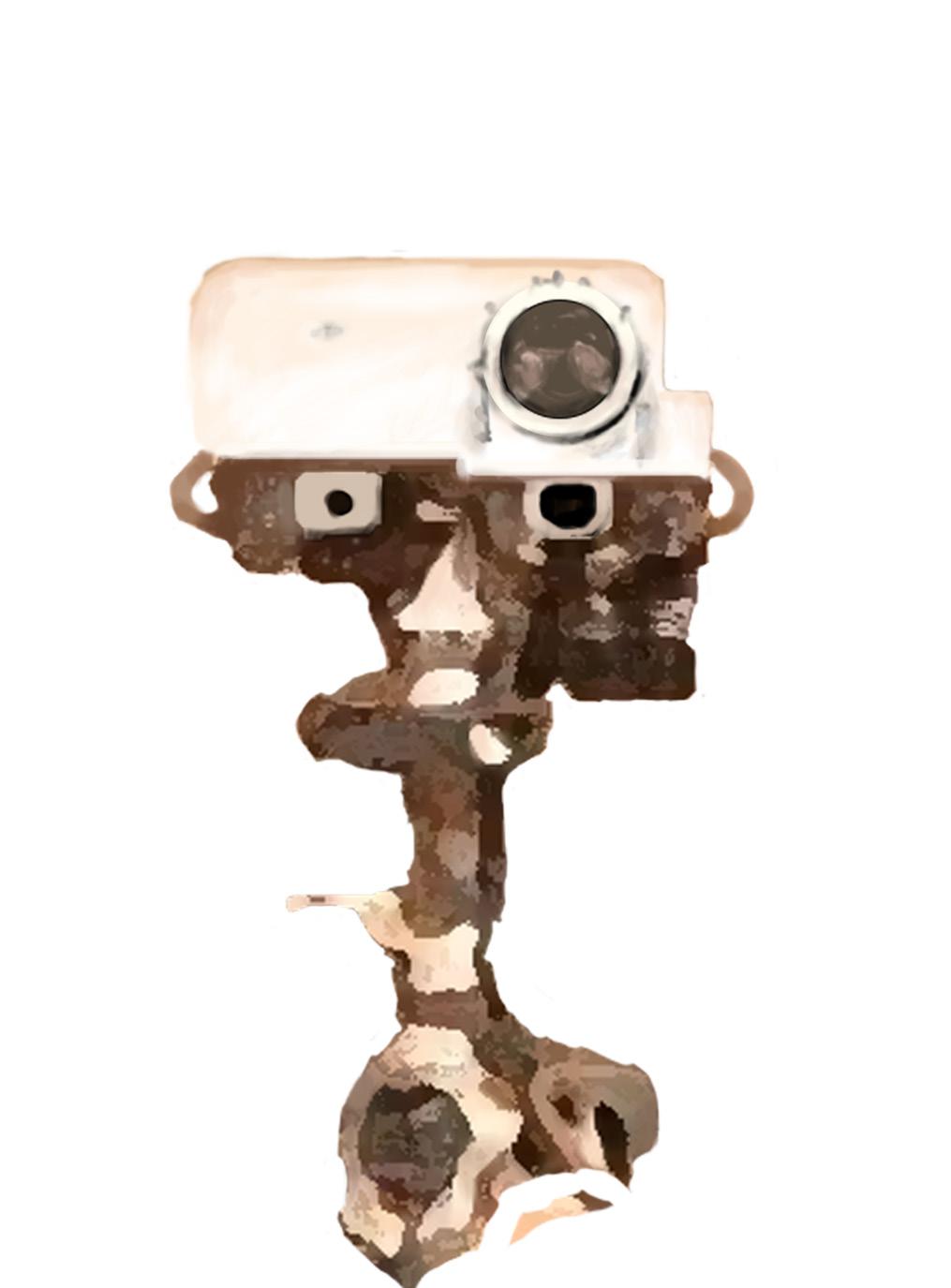
Perseverance Rover’s SuperCam by Allen Wu (VI)
The next instrument is quite similar to PIXL, in terms of both technology and purpose. SHERLOC, short for the Scanning Habitable Environments with Raman and Luminescence for Organics and Chemicals device, consists of a Raman and fluorescence spectrometer and a laser. Similar to fluorescence spectroscopy, Raman spectroscopy identifies an object’s chemical structure using a laser. Specifically, the laser light reflecting from that object is analyzed by a spectrometer — only the approximate 0.0000001% of light with different wavelengths to the original laser provides data — which is able to identify different bonds (C-C, C=C, N-O, etc) and groups of bonds (benzene rings, lattice modes, etc). Thus, SHERLOC can determine an object’s elemental composition and chemical structure, and this data can be used to detect minerals, organic materials, and potential biosignatures on Mars. Lastly, the SuperCam is a collection of instruments capable of identifying rock textures, atomic and molecular compositions, and chemical structures of different rocks and soils. Carrying technology from the Curiosity Rover’s ChemCam, the SuperCam can zap objects up to 20 feet away with a laser and analyze the resulting vapor to determine that object’s elemental composition. Furthermore, it can perform Raman spectroscopy to determine chemical structures, Time-Resolved Fluorescence spectroscopy (TRF) to identify intermolecular interactions and Visible and Infrared reflectance spectroscopy (VISIR) to classify the mineralogy of different soils and regoliths. Overall, these various instruments that constitute the SuperCam enable the rover to study the molecular structure of different samples as well as search for organic materials, all from afar. With all the data already sent back by Perseverance, it appears that the rover and its scientific instruments are functioning as planned. Now, it is the rover’s responsibility to explore Mars and complete its tasks as we patiently wait and watch. Undoubtedly, this mission will be vital for future Mars exploration, from increasing our understanding of the foreign Red Planet to preparing for future manned missions. Hopefully, Perseverance will be a major success that we can look back on in gratitude during future Mars missions.
Works Cited
1. Bell, J., Rodriguez-Manfredi, J. R., Hecht, M., Allwood, A., Hamran, S.-E., Luther Beegle, & Wiens, R. (n.d.). Instruments. NASA. Retrieved March 16, 2021, from https:// mars.nasa.gov/mars2020/spacecraft/instruments/ 2. Gifford, S. E. (2014, February 18). Calculated Risks: How Radiation Rules Manned Mars Exploration. Space. Retrieved March 16, 2021, from https://www.space. com/24731-mars-radiation-curiosity-rover.html 3. Mars 2020 Mission Contributions to NASA’s Mars Exploration Program Science Goal. (n.d.). NASA. Retrieved March 16, 2021, from https://mars.nasa.gov/mars2020/ mission/science/goals/#:~:text=The%20Perseverance%20 rover%20is%20designed,in%20which%20it%20was%20 formed 4. Redd, N. T., & Wall, M. (2021, March 2). Perseverance rover: NASA’s Mars car to seek signs of ancient life. Space. Retrieved March 16, 2021, from https://www.space.com/perseverance-rover-mars-2020-mission 5. Wood, C. (2021, March 1). With MOXIE, Perseverance will try to make oxygen on Mars. Popular Science. Retrieved March 16, 2021, from https://www.popsci.com/story/ science/moxie-instrument-on-perseverance-mars/#:~:text=Instead%2C%20MOXIE%20takes%20in%20all
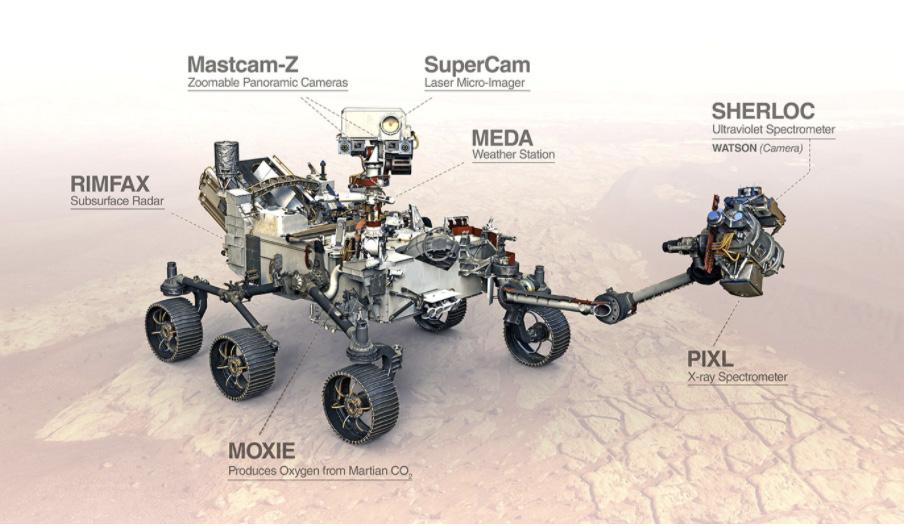
Diagram of the rover’s scientific instruments