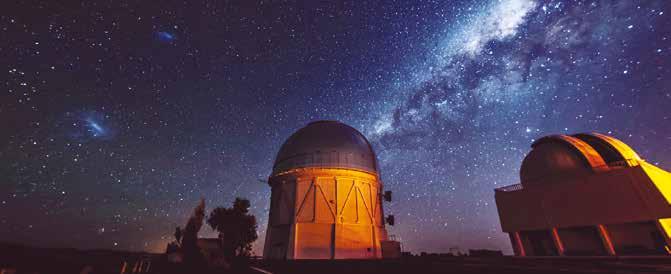
7 minute read
Shedding light on the Dark Universe
by Dr Alexandra Amon (e2022), Trinity Senior Postdoctoral Researcher
Our planet is an almost infinitesimal speck in the cosmos. It orbits the Sun, one of billions of ordinary stars, which hurtles through our galaxy at about half a million miles per hour. Beyond this, there are billions of galaxies in our Universe, each boasting their own hoard of stars and planets.
In humans’ insignificant snippet in the grand story of the Universe, we have honed a standard cosmological model. It serves as an undeniable success story: with just six parameters, we can describe the evolution of our Universe over cosmic time, from a soup of primordial particles to the web of galaxies in our night sky today. Remarkably, this model has survived rigorous testing by a plethora of cosmological observations – exploding stars as cosmic candles; the distribution of galaxies; and temperature fluctuations mapped in the first light of the Universe. It may sound like we cosmologists have the Universe sussed, but
Blanco Telescope, Cerro Tololo, Chile, for the Dark Energy Survey, under the Milky Way. the elephant in the room is that the cornerstone to this theory is a Universe dominated by dark matter and dark energy. Indeed, just five percent of our Universe is made up of particles whose physics we understand.
Dark matter neither emits nor interacts with light. Despite being invisible, and capable of evading particle physicists’ giant underground detectors, it plays a crucial role in the story of our Universe. More than three quarters of the mass in galaxies, including our Milky Way, is dark matter, rather than stars or planets. It exerts a large gravitational effect that glues the stars of galaxies together – dark matter is the invisible scaffolding of our cosmos.
Dark energy is even more mysterious. It drives the accelerated expansion of our Universe. That is, our observations reveal that while stars stay tightly bound in galaxies, as cosmic time marches on the galaxies themselves are moving further away from each other at a rate which is getting faster and faster. Our current understanding holds dark energy responsible, but the prediction of its nature by quantum physics is starkly wrong.
© REIDAR HAHN, FERMILAB/DARK ENERGY SURVEY
My job, therefore, is to study the invisible. While we cannot see these entities directly, we infer their existence from their influence on things we can see. It is my immense privilege to play a leading role in the Dark Energy Survey (DES)1, an international team of over 400 scientists. We use the Blanco telescope, nestled on a mountain top in Chile, to scan our night sky and harness data from the cosmos. With these spectacular images, we make measurements, and use them to address the Big Questions – What is our Universe made of? How did it evolve to the way we observe today? We are not the first to ask these questions – we know that even ancient civilisations looked up at the night sky and pondered these grand ideas. We have come a long way with our understanding, pillared by landmark moments that drastically altered the way we view our place in the Universe – from Copernicus, Einstein, Trinity’s own Eddington and Newton – but it’s clear we still have a long way to go. As the standard model has held up to whatever cosmological measurements have been thrown at it, the game is to keep throwing: to find new tests and conduct more precise measurements, with ever-better instruments, to finally reveal the true nature of these unknowns.
One of the most powerful tools to do so is weak gravitational lensing. When we observe a distant galaxy, we collect its light in our telescopes after it has journeyed for billions of years across the Universe. According to our theory of gravity, General Relativity, dark matter, like any massive structure, warps the space-time fabric of the Universe. As such, the path that the light travels along is altered and the image of the galaxy that we capture appears slightly distorted. The gravity due to any dark matter along the line of sight has the effect of lensing the galaxy – making it appear more stretched in our images and inducing a coherent alignment among nearby galaxies. The stronger the average galaxy ellipticity in a patch of sky, the more dark matter there is in that region of the Universe, assuming those galaxies are, in reality, randomly oriented. Therefore, the induced distortion of the galaxies is a faint signature of dark matter inscribed throughout the Universe. We measure this alignment to extreme precision and map the matter lying between us and the distant galaxies. As part of the Dark Energy Survey, over three years we imaged 1/8th of our night sky and catalogued the positions, shapes and distances to more than 100 million galaxies. With this, we conducted the most rigorous statistical analysis of its kind, testing against the predictions from standard model2 .
This is no easy feat – while gravitational lensing is a powerful cosmological technique, it is extremely technologically challenging. The typical distortion
The largest dark matter map of our Universe
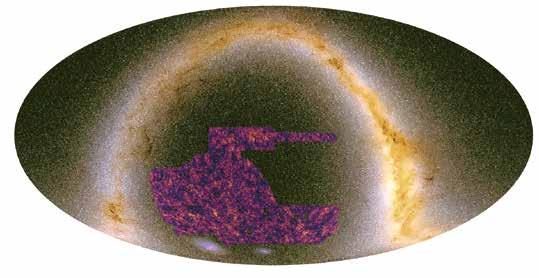
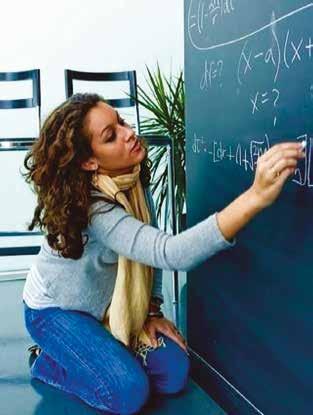
As a student on the summer programme at the Perimeter Institute, Canada.
© PERIMETER INSTITUTE
© N. JEFFREY; DARK ENERGY SURVEY COLLABORATION
induced by dark matter is less than a 1% alteration to the observed shape. As the lensing effect is weak, in order to detect it we need very large samples of galaxies. This data challenge necessitates rapid processing of petabytes of data. A scientific hurdle arises as the weak lensing distortions are significantly smaller than the distortions that arise in the last moments of the light’s journey. Due to the effect of the Earth’s atmosphere, even in the exquisite conditions of the Coquimbo mountains, and our imperfect telescopes and detectors, our galaxies aren’t the picturesque spirals you’d imagine, instead fuzzy blobs spanning only a few pixels. In order to isolate the dark matter signature, these nuisance distortions are mathematically modelled to high precision, allowing an accurate recovery of the cosmological signal. With a new machine-learning technique, we infer galaxy distances – an effort that I led with my colleagues at Stanford University. Further complications arise due to the intrinsic properties of galaxies and their effect on the surrounding matter that needs to be modelled. To minimise our human bias, we do all this ‘blindly’ – using intentionally tampered data throughout three years of testing, until we are convinced of our calibration and model choices and are ready to reveal what the Universe is really like.
It is a particularly exciting time for cosmology. Let me set the scene: there are several competing teams performing weak lensing measurements independently. We use much larger datasets than ever before, desperate to see if what we find is consistent with our model or, perhaps, if we find new cracks in the grand picture. Finally, we see hints of them. Like our ESO Kilo-Degree Survey and Japanese Hyper Supreme Camera colleagues, DES found that weak lensing predicts a slightly less clumpy Universe than would be expected based on the prevailing model of cosmology. This suggests a need for either new physics, a new problem in the analysis to be solved, or perhaps just more data. It is exciting to think that it is time again for a new landmark moment – a call for some modification to our peculiar dark matter/ dark energy-dominated model. With my fingers crossed for new physics, I co-coordinate the weak lensing science team and we are in the heat of analysing the latter three years of DES data.
It is a humbling field to be working in that asks what the Universe is made of and how its structure evolved with the formation of galaxies and our own existence. Our ongoing experiments act as training grounds for an epic decade for cosmology. We are at the dawn of several major international projects that will survey the sky to greater depths and resolution than ever before. The Vera Rubin Observatory will image the entire Southern sky every few nights, building the deepest and largest map of our cosmos, and the Euclid satellite will survey the sky from a vantage point in space, eradicating the worry of Earth’s atmosphere.
There is an exceedingly bright future ahead, bringing us closer to fathoming our Dark Universe.
1: darkenergysurvey.org 2: nature.com/articles/d41586-021-01466-1
Trinidadian cosmologist Dr Alexandra Amon is Senior Kavli Fellow at the Kavli Institute for Cosmology, Cambridge, and she is passionate about science outreach and making STEM fields more accessible and welcoming to everyone. After her undergraduate degree and PhD at the University of Edinburgh, Alexandra was a Fellow at Stanford’s Kavli Institute for Particle Astrophysics and Cosmology. In addition to her role at Trinity, she is Paczynski Visiting Fellow at Princeton.
amonalexandra.com @astroalexamon @astroalexamon
The Blanco telescope, with me for scale!