Innovation Projects
A taste off our cutting edge energy technologies
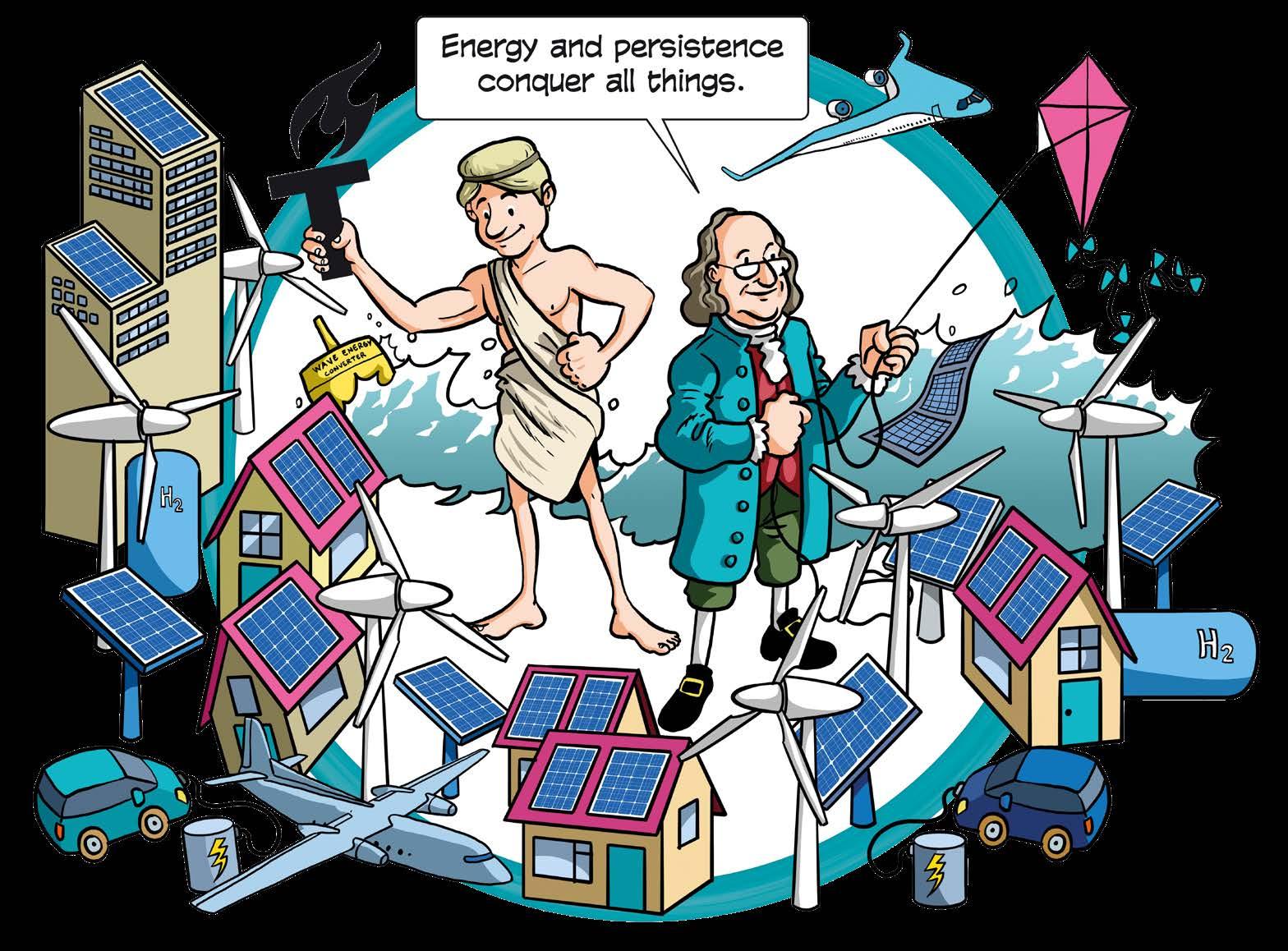
This table of contents is clickable, allowing you to navigate directly to the desired page. To easily return to this page, click on this symbol at the bottom of the page.
This table of contents is clickable, allowing you to navigate directly to the desired page. To easily return to this page, click on this symbol at the bottom of the page.
Our mission ‘impact for a better society’ is more appropriate than ever when it comes to one of the major challenges we are working on at the TU Delft: the energy transition and the shift towards a sustainable society.
At TU Delft, around a thousand scientists are working on technological innovations, covering the entire spectrum of the energy chain. This involves both generating renewable energy, both offshore and onshore, and developing an energy system that is suitable to handle these new forms of renewable energy. But we also look beyond that: we explore how our buildings can be made more sustainable and we are heavily involved in industry and the transport sector.
The challenges we face and the technological solutions required are of such complexity that profound collaboration will be essential in ensuring success.
The TU Delft Innovation & Impact Centre is instrumental in fostering such collaborations through diverse channels, including setting up field labs in which companies can work together with scientists, researchers, startups and the public in testing, validating and further developing new innovations.
At the Green Village, the field lab for sustainable innovation on TU Delft Campus, we are resolutely committed to reducing CO2 emissions. The 24/7 Energy Hub project is about developing a local, CO2-free energy system for the built environment, including hydrogen infrastructure. Another excellent example of collaboration is the ESP Lab, developed in cooperation with grid operator TenneT. Within this lab, a complete digital replica of the Dutch electricity grid is being built, thus facilitating its future-proofing in a secure and pragmatic manner.
In our effort to accelerate innovation we are also working hard on the development of other field labs, in areas like floating renewables and e-refinery.
This booklet is intended to give you an impression of the innovation projects that we actively develop and implement at TU Delft, often with the help of partners, that focus on the energy transition and a sustainable society. Together, all of these projects provide excellent examples of the wide variety of research and innovation at TU Delft and within our innovation ecosystem. The projects have been captured in exciting cartoonish visualisations and rated according to their technology readiness level. All of the information can also be found online here.
Do you also share our ambition for a more sustainable society? Let this publication be your guide and we will be happy to join forces with you in working towards a sustainable future.
Sincerely,
Kemo Agović of the Innovation & Impact Centre… an environment where products and services consume high amounts of energy and end-users struggle to make their behaviour more sustainable. Our linear economy leads to depletion of natural resources and exhaustion of scarce materials.
… an energy system designed for centralised energy production, one-directional energy distribution, and human decision-making. It can’t facilitate large numbers of decentralised renewable energy production and storage facilities.
… a society that relies on fossil energy sources for producing electricity, fuelling transport, and feeding chemical industries. Today, there are no effective solutions to fully mitigate their CO2 emissions.
… a sustainability-aware society where green products and services are available to everyone, powered by efficiency and circularity.
… a reliable, safe, cost-effective, fair energy system that balances supply and demand through intelligently controlling energy production, distribution, storage, and usage at all scales and all times.
… a carbon-negative society where clean energy is abundant and industry uses fossil-free sources of carbon such as CO2, biomass, or waste as feedstock.
Delft University of Technology is on a mission: “Impact for a better society.” Leveraging our strong scientific foundation in science, engineering, and design, we provide cutting-edge solutions to societal challenges. Our solutions are born from groundbreaking research, making us an ideal partner for knowledge institutes, society, and businesses.
We value our long-standing industry partnerships and are eager to welcome new collaborators. Whether through bilateral agreements or programs like Horizon Europe, national top sector funding, NWO, or regional funding, we’re open to forging new alliances.
In today’s rapidly evolving global market, speed is paramount. We believe that multi-party collaborations drive innovation. We invite businesses to join our community and collaborate with TU Delft and the parties in our TU Delft Campus ecosystem. Together, we’ll address your research and innovation challenges by connecting them with our network of academics, students, corporates and entrepreneurs.
Our goal is to elevate TU Delft Campus into an internationally significant public-private innovation hub. To achieve this, we seek strong connections with knowledge institutions, governmental bodies, corporations, SMEs, startups, scale-ups and investors.
In conclusion, we invite you all to join us in creating a brighter future for society and markets. Let’s collaborate and make an impact for a better society!
Zwanet van Lubek, Manager Corporate Innovation
Please click here to contact me.
Contact our innovation managers today to explore opportunities for collaboration:
Innovation Manager Field of Expertise
Friso Lippmann Offshore Renewables
Maxim Segeren Offshore Wind
Peter Lucas Hydrogen – Full Value Chain
Ronald Hemerik Hydrogen – Storage and Transport
John Nijenhuis e-Refinery
Lily Li Power Grids
Rosalie Driessen Batteries
Caroline Duterloo Energy Transition & Artificial Intelligence
Arnoud van der Zee Energy Transition & Built Environment
Liselotte de Vries Energy Transition & Agriculture-Food
Bas Venneman Energy Transition & Future of Work
Get in touch
Click to mail Friso
Click to mail Maxim
Click to mail Peter
Click to mail Ronald
Click to mail John
Click to mail Lily
Click to mail Rosalie
Click to mail Caroline
Click to mail Arnoud
Click to mail Liselotte
Click to mail Bas
In total eleven research themes have been used for a thematic categorisation of the innovative projects. Each research theme has been assigned its own colour. You can find the themes on top of each project page. Please also see index page 73 to search by research theme.
Delft University of Technology has eight faculties. For all projects we have indicated which faculties are involved using the following icons:
3mE Mechanical, Maritime and Materials Engineering
ABE Architecture and the Built Environment
AE Aerospace Engineering
AS Applied Sciences
CEG Civil Engineering and Geosciences
EEMCS Electrical Engineering, Mathematics & Computer Science
IDE Industrustrial Design Engineering
TPM Technology, Policy and Management
The University is full of interesting, innovative research within its own specific theme and stage of development. Finding a project that matches your interest can be complex without some guidance. We have listed the projects according to their TRL level.
How are you doing back there?
Great!
Much more effective!
Thanks, I feel so much more energetic!
After a wind turbine extracts energy from the wind, a slow-moving wind field known as ‘the wake’ lingers behind the turbine for quite some distance. Since wind turbines are usually closely spaced in farms, these wakes significantly affect downstream wind turbines’ production totaling up to 20% performance loss annually. The Helix approach is a novel control technology that reduces this negative effect by periodically moving the flow to create a helix-shaped wake, which allows more efficient mixing of the low-velocity wake with the high-velocity free stream. This method differentiates itself from the state-of-the-art by introducing dynamics while deviating only slightly from a turbine’s normal operational envelope. However, the biggest challenge is balancing the costs associated with the fatigue increase generated by this control approach with its production gain. In a commercial setting, a wind farm developer might choose to activate the Helix when the electricity prices are high to generate additional revenue, while it may decide to deactivate the Helix during periods of low electricity prices, to reduce the strain on the turbine.
Moving from bottom-fixed turbines in shallow waters to floating turbines in deep waters, Future developments could include co-design of floating wind turbines and wake mixing controllers. By leveraging the extra degrees of freedom of a floating turbine wake mixing may be instigated through movement of the turbine, reducing the demand of critical actuators. This would save significantly on actuator costs and improve the turbine’s lifetime, while still benefiting from wake mixing. Other developments include the synchronization of the wake mixing control action of the downstream turbine with the upstream turbine, such that the actuator costs and production gains can be optimized further.
Wake mixing controllers such as the Helix approach may revolutionize the wind energy sector and our journey towards a carbon-neutral future. Its strength lies in its simplicity. Without the requirement of conceptual changes, such as additional flaps or different rotor layouts, the Helix approach can readily be implemented in most existing commercial wind farms through a simple software update.
dr. ir. Axelle Viré prof. dr. Dominic von Terzi, prof. dr. ir. Jan-Willem van Wingerden, prof. dr. Andrei Metrikine, dr. ir. Delphine De Tavernier, dr. ir. Michiel Zaaijer Siemens Gamesa Renewable Energy, Offshore Renewable Energy Catapult, EireComposites, GDG, Ideol, MARIN, NREL, Politecnico di Milano, University College Cork, DTU Wind Energy, INSA CORIA
The idea of floating wind is that you put a wind turbine on a floating support structure so it can capture wind energy. The advantage is that you can install wind turbines almost everywhere in the ocean which opens up new markets for harvesting this resource. However, as the turbine is no longer fixed to the sea floor its behaviour is quite different due to the influence of wind and waves. With high fidelity modelling the lead researcher and her team are looking at all the conditions that need to be taken into account for building these floating turbines – the type of turbine and floater, and the environmental conditions. Through this way of modelling she can test the assumptions of the engineering model before a physical model is built to be put to the test.
Since the research is mostly model-based the next step is to build experimental facilities where the models can be physically tested. Parts of the facilities you would need to test floating wind are already on campus but there is need for smart way of connecting these. For instance, in the wind tunnel you would need a hexapod that moves mimicking the floating behaviour of the turbines. Another next step is joining the floating wind research with research on producing hydrogen far offshore so you don’t have to transport the electricity over huge distances.
There is a huge need for suitable locations to install wind turbines. Wind at sea is one of the preferred options for capturing wind energy compared to wind at land. The current wind farms at sea are fixed to the sea floor and hence installed in shallow seas. More space is available in deeper waters which can be captured by using floating wind turbines instead.
The potential of solar farms at sea is huge. The researchers work on designing and testing various types of solar structures that float. They look at lightweight modular structures that can move with the waves. This raises all sorts of questions about the hydrodynamic effects; on how do we prevent waves from washing over the floating structure but also on what happens to the waves while travelling underneath the structure. From the structural perspective, wrinkling and fatigue are but two of the topics that are investigated. And practically, how can the structure be installed and moored in the most effective way? The researchers are combining experiments in the towing tank on small scale models with numerical simulations to gather data for deeper insight into the physics and for further optimization of the design so it can be implemented at open sea.
The next step is to build the lab tested prototypes to full scale. A change of scale can have a huge impact on how the floating solar panels behave; what works at lab scale can be disastrous a full scale due to how the materials react. Another next step is to look at the interaction of the panels with life at sea. How fast will barnacles and algae use the floating panels as a substrate. And can the panels be designed and constructed in such a way that part of the light falls through the panels for under water growth.
With floating solar panels in between the wind turbines at sea we can harvest 3-5 more renewable energy at the same area than with the wind turbines alone. Solar panels require lots of space, which is scarce on land. With floating solar panels combined with offshore wind turbines, more renewable energy can be harvested and offshore electrical infrastructure can be used more efficiently.
Waves have a great energy potential as a renewable resource. The researcher is working on combining numerical wave modeling with mechanical engineering creating a system that is globally adaptable. On the one hand the research looks into how the waves change over the years with changes in the climate. Building models this gives information about the wave energy resource but also for coastal protection. On the other hand, studying the wave resource numerically allow us to know how to optimally design, how to optimally place a wave energy converter. The researcher can look into what is the optimum device you should be using, how to optimize wave energy converters in terms of operation and location. Another benefit is that with the models the researcher is looking at where you can place energy converters in array, not only making them more efficient but also as part of the coastal protection system.
The researcher is looking into how the different renewable resources can interact so that there will be less variability in the electricity that will reach people’s homes. Subsequently he is working on developing a real time hybrid testing methodology for optimising wave energy converters.
By adding wave energy to the renewable energy mix – besides solar & wind - you can get a better and more fluid production of energy. There is a delay between solar, wind and wave energy reducing the use for storing renewable electricity – which is very expensive. Besides wave energy has a great resource potential increasing the amount of renewable energy that can be generated.
Renewable energy can be converted and stored in the form of green hydrogen. One of its biggest benefits is that hydrogen can be stored in large scales (TWh), much beyond the scope of electricity-based batteries. This requires huge space, several billion cubic meters, as for its low volumetric energy content. The solution to this challenge is to utilize earth underground reservoirs. which offer giant capacities to store billions of cubic meters of hydrogen at high-pressures. Up till now little is known about how hydrogen behaves in the subsurface – the science is missing. In the newly established hydrogen research lab, the researcher is investigating not only how it behaves in the subsurface but also what type of subsurface would be the right reservoir to store it at a given quantity and scale. With a uniquely integrated multidisciplinary approach the researcher and his team are finding solutions to make this technology ready for field deployment within a few years.
The next step for the underground hydrogen storage research is to investigate, at real-field conditions, how hydrogen will interact with the reservoir and cushion gas fluids. Specially mixing and reactivite physics under cyclic transport of hydrogen is to be investigated.
Hydrogen is a very attractive renewable energy carrier. While we aim to scaleup renewable energy production, if we establish ways to store green hydrogen at large-scale (TWh) safe and pure in the giant underground reservoirs, we will certainly accelerate energy transition by several folds.
Hydrogen is created by putting electricity on a electrolysis cell filled with water. The water will be split into hydrogen and oxygen. Although this process is known for many years doing so on a large scale and in a sustainable way has many challenges. The first challenge is how to perform electrolysis with renewable energy. The supply of renewable energy fluctuates. For the longevity of the electrolyser you would want it to operate continuously. Research is being done on how this can be achieved. Seconly, for electrolysis you need critical materials for the electrodes which area scarce. Here the researcher is looking at how can we distribute this material as thinly as possible with nano layering technology so we can use it more efficiently and need less of the scares material per cell. The third challenge is to find smart ways to scale up the size of the electrolysers in 3 dimensions.
For hydrogen the next step is to build installations on industrial scale. Another next step is to see if a renewable fuel can by produced directly from CO2 using electrolyse technology or by combining hydrogen directly with CO2 using catalysts.
We need a conversion in order to store renewable energy. Batteries are very costly to store huge amounts of energy. So we are looking for methods to chemically store large amounts of energy of which Hydrogen is one of the possibilities.
Concrete is seen as an unfavourable material with regards to high CO2 emissions due to the manufacturing process. The researcher is focused on using 3D printing in combination with concrete to make novel materials which could improve that image. He came up with a type of concrete that is very deformable. With 3D printing he created specially shaped holes inside of the concrete that makes the normally so solid concrete more flexible. This shape together with a special fiber added concrete mixture allows it to move when under pressure and without damage to the material. The holes are perfect for adding piezo-electric sensors which in turn can harvest any form of kinetic energy and transform it into electric energy. This type of concrete could be applied in public buildings where you have a lot of pedestrians that make it a bit deformable.
The results on the energy capturing part are promising – the principle technology works. Currently the researcher is working on how to you optimize and how do you upscale. The researcher is also looking for industrial partners that are interested to collaborate. For instance on the electrical engineering part. Besides there are still some unknowns about the applicability of the technology in seawater conditions. For the concrete that will not be a problem but how will the metallic parts perform? Eventually the researcher is working towards doing pilot tests in a few years’ time.
With regard to sustainability people think about replacing concrete with more green building materials but the researcher believes it is not possible on the short or medium term. So he thinks we should look for way to get things back form concrete. He thinks he can contribute to getting back more than we do now in terms of energy par example.
Dr. Frans van de Meer
By combing their fields of expertise, the researchers have developed a novel approach to design mechanically intelligent structures for offshore and underwater applications. Using AI, the researchers have been able to invert the design process. Normally, one has to do a multifold of iterations or wait for a “Eureka!” moment to come up with a design with tailored properties. In contrast, AI can help quickly and efficiently reverse engineer the optimal design directly starting from the desired property of a material or structure. Using this novel method, they have been building prototypes of novel structures for maritime and offshore applications, where extreme environments impose atypical and case-specific design requirements for material and structural behaviors.
Subsequently, this novel design process is applied to smart materials giving access to functionalities that have not been achievable before. Now they can design novel metamaterials that have unique behaviors such as piezoelectricity-based energy harvesting capacity. Leveraging AI-driven acceleration, the researchers have been able to design and construct such exotic materials and structures from the micro to the macro scale in a much more efficient, creative and faster manner.
What’s next?
One of the next steps for the researchers is to explore new and more complex material spaces: from piezoelectric to magnetomechanical and shape memory materials. On the AI side, the researchers are developing fundamentally new AI methods for mitigating the data-hungry nature of such AI-based design frameworks. Additionally, designing on the computer is one thing, but when the structure is deployed in real life – how can AI design human-centric and safe structures that are robust and resilient to the uncertainties of extreme environments?
Offshore structures and machines can benefit from adapting to extreme offshore environments and utilize them for added functionalities in their performance. Our solution contributes to designs that can conquer the most remote offshore locations ultimately creating opportunities for offshore energy transition in the deepest corners of our oceans.
dr. Sid Kumar, dr. Jovana Jovanova
Designing mechanically intelligent structures with AI
Concrete is seen as an unfavourable material with regards to high CO2 emissions due to the manufacturing process. The researcher is focused on using 3D printing in combination with concrete to make novel materials which could improve that image. He came up with a type of concrete that is very deformable. With 3D printing he created specially shaped holes inside of the concrete that makes the normally so solid concrete more flexible. This shape together with a special fiber added concrete mixture allows it to move when under pressure and without damage to the material. The holes are perfect for adding piezo-electric sensors which in turn can harvest any form of kinetic energy and transform it into electric energy. This type of concrete could be applied in public buildings where you have a lot of pedestrians that make it a bit deformable.
The results on the energy capturing part are promising – the principle technology works. Currently the researcher is working on how to you optimize and how do you upscale. The researcher is also looking for industrial partners that are interested to collaborate. For instance on the electrical engineering part. Besides there are still some unknowns about the applicability of the technology in seawater conditions. For the concrete that will not be a problem but how will the metallic parts perform? Eventually the researcher is working towards doing pilot tests in a few years’ time.
With regard to sustainability people think about replacing concrete with more green building materials but the researcher believes it is not possible on the short or medium term. So he thinks we should look for way to get things back form concrete. He thinks he can contribute to getting back more than we do now in terms of energy par example.
Currently we drive monopiles into the ocean floor for the foundation of wind turbines at sea. Usually long steel cylinders are driven down by knocking at the top. New technologies have been developed where these piles are driven in the ground by shaking them vertically. This still produces quite some noise and once in a while the pile gets stuck before reaching the desired penetration depth. A novel method for driving piles is developed inspired by how we open champagne bottles. This method still uses a shaker for making the pile vibrate. However, not only does it vibrates vertically, but also a torsional motion is excited at a much higher frequency. This new shaker has been demonstrated to drive the piles smoothly and quickly and reduces the underwater noise emitted during pile driving as torsional vibrations emit very little noise into water.
As the next step the novel shaker is being upscaled for XXL monopiles. This is done by implementing new engineering solutions, which have never been used in pile driving in the past. In parallel, a sensor is being developed which will go onto the pile in order to automatically determine the amplitude and frequency for each of the two directions of motion for the shaker. Subsequently, research will be conducted to make this sensor contactless and dron-based.
This method piles will increase the installation speed of piles as it is more efficient. Importantly the same method can be used for removing the piles without disturbing the soil too much. Finally, that this pile driving method is more environmentally friendly than the conventional methods as it emits much less noise into water. This removes the necessity to employ very costly noise mitigation measures as the environmental impact of this driving method is expected to be within the allowable limits without any additional mitigation.
In the past we had large fossil fuel fed generators that produce a lot of energy on a stable, robust, controllable and predictable way. With the increased generation of renewable energy we are downsizing in terms of scale – there are many more units that feed into the network with increase variability and uncertainty. This causes power volatility, large frequency deviations and voltage regulation problems which are challenging to manage. The researcher aims to improve the technical characteristics of the renewables so they mimic the behaviour of a conventional generator – by developing ways in which they can aggregate the behaviour of many solar panels or wind turbines. With electrical power engineering, control theory, ICT, algorithms and other methodologies he is building controllers embedded with intelligence for the renewables. The biggest challenge is to develop controllers that can simultaneously do multiple things.
The next step is to move from the technical development into the more regulation and market developments. These controllers do not exist yet. They need to build these controllers at low cost And it would be preferred for the controllers to have a dimming function so the spilling of energy can be reduced developing new services.
With the development of these controllers the volatility of a renewably fed energy system can be more easily managed to ensure a steady supply of electricity to every socket in the network.
For the energy transition more and more renewable electric energy is being produced, transported and used. This increase is leading to the limits of the transportation capability of the electricity grid. The grid struggles to connect distributed energy resources like solar panels or electric vehicles through end-users. Solving this capability challenge by investing in new infrastructure alone will not suffice as the network expansion rate is limited due to workforce shortage. Together with distribution system operators Alliander this ICAI lab aims to enhance the transport capability of the distribution grid in The Netherlands by improving the grid operation on the on hand and looking at targeted infrastructure expansions on the other hand. The lab combines the expertise of Alliander, the latest digitalization programs that make grid data available, with the academic expertise on methods from artificial intelligence enabling the labs scientific success and broader global impact. However, developing and using AI methods for a real-world cyber-physical critical infrastructure is not straight-forward. For example, one of the five challenges the lab addresses is that the real grid has insufficient or false measurements, noise, unclear topology, and other data quality problems. Researchers will be working on how AI methods can be generalized for real-world power system operation and be made robust against unavoidable data quality issues. During pilots it will be tested how the state-estimator scores on accuracy, reliability, repeatability and resilience, and this will be compared with baseline models on fully measured parts of the grid. This increased insight will making the current grid to be used much more efficient, e.g. by lowering safety margins or implementing flex-markets to overcome overloads.
The beauty of this ICAI lab is that research results can directly be implemented by Alliander. The outcome will be that new solutions for congestion management, smart charging or flexible grid switching modes can be implemented much faster and with lower effort. As a result, the safety margins can be lowered, leading to a more efficient use of the power grid.
The work carried out in the ICAI lab contributes to a robust, reliable and resilient electricity grid. It will enhance the transport capability of the Dutch grid by improving operation while taking into account targeted infrastructure expansions.
In the future, the increase in generation of energy from renewable sources will intensify stability problems and will increase the uncertainties in planning and operation of electric power systems. Unexpected disturbances and inadequate system monitoring can cause catastrophic failures such as blackouts. Existing monitoring and control schemes often cannot cope with these problems due to the lack of coordinated control when the system is affected by large disturbances. The researchers aim to create a wide-area intelligent system, that empowers the future power grids by providing real-time information, quickly assessing system vulnerability, and performing timely corrective control actions based on system-wide considerations. The researcher developed algorithms that can help in deciding when and where to split parts of the grid in order to maintain the grid stability, prevent blackouts, and minimize the impact on the power consumers.
The innovative idea has been developed for monitoring and control of the stability of medium to large scale electric power grids up to the European scale. The next step could be to apply the found solution for protecting a realistic power grid. Furthermore, some enhancements in terms of modelling would be desirable to capture the full degree of complexity of large-scale power grids.
With a new way for timely corrective control the researchers aim to prevent catastrophic blackouts, independent of any future network generation mix, unpredictable topology and load profile.
With the rise of more solar panels, electric vehicles or even more houses or energy intensive commercial buildings there is an increasing stress on our electricity network. Situations where supply and demand are unbalanced can be resolved by increasing the capacity of the network or can be met by exploring smart energy management solutions. Against the backdrop of further urbanisation in the Amsterdam ZO-region, designing and operating urban energy systems in an inclusive is a major challenge of the energy transition. The researchers aim to develop a scalable energy exchange platform to resolve both grid challenges and foster the participation of local residents into energy challenges. Through the platform they want to engage both large customers and residential energy users. The insight into how the exchange and other energy services will work will be simulated by setting up a digital twin.
When the math core of the digital twin is active and people from the companies and neighborhoods can submit their data to it the next step is to start writing applications for it – open source – enabling a participatory urban energy system. This concept will be scaled up technically with heat network extensions and geographically targeting other areas such as Rotterdam Schiebroek.
With the development of a smart energy exchange platform the researchers aim to contribute to battling energy poverty and increase awareness of what the energy transition entails for the local stakeholders. The participation process allows the planning and development of grid-friendly and community inclusive energy innovations.
Adding batteries to the grid might be a way to mitigate the impact of the expected fluctuating energy supply when we rely and solely use renewable resources. This is not easy to achieve. Conventional batteries are very expensive and rules and regulations are missing to determine who can add and own such a battery to the network as consumers can become suppliers too. This makes the business case unclear. Developing and adding functionalities to the usage of the battery can help sort out the business case. The researcher aims to develop techniques and guidelines for the sizing, location, and control of battery energy storage systems in distribution grids and for the design of the power electronics converter used to interface the storage system with the network. For this he is developing a special power convertor and developing algorithms for the integration of the batteries in the grid.
For this innovative idea to work the next step would be to change policy so it would become easier for these batteries be deployed. These batteries open up new possible business models for storing and using the energy when needed. Another next step, technology wise could be to consider more applications for the deployment of batteries to the grid.
Transforming our energy system to rely solely on renewable energy can destabilize our grid, especially in cases of congestion or peak usage of electricity. By adding batteries to the grid local buffering reservoirs where energy can be stored and retrieved can be created. These batteries give the grid more stability and make it more healthy.
The way our electrical grid operates is a continuous balancing act - what is generated must be balanced with what is needed at any instant. This is done by transmission system operators. Fluctuating renewable energy causes imbalances between consumption and production of electricity. Modern renewable generators are connected to the grid through a power electronic converter. In a conventional electric system, the consequence is that inertia from a renewable generator is not transferred to the grid. In systems with zero or low inertia, power failures in the grid cause fast and large dynamic phenomena. The longer it takes to respond to these instabilities, the more energy is required to restore the system to its stable state. In the absence of inertia conventional control and protection equipment cannot work properly anymore, and therefore the grid stability is at risk. The researcher is working on developing new control principles for our electrical grid on a system level.
MIGRATE was mainly focused on onshore generation systems so a next step would be to look at the generation and control of offshore renewable systems. Another next step will be looking into new principles of control of the grid when you connect huge capacity electrolysers to the grid or what type of control is needed when different consumers and devices can provide support to stabilize the grid.
Our current electrical grid is very inert due to the decoupling of renewable power plants. The researcher works on new fundamental operational and control principles for complex, unpredictable, and inherently instable future offshore-onshore energy systems dominated by renewable energy sources.
The increased electrification of the built environment creates challenges of congestion. This fluctuation in available electricity creates problems for the operation and eventually the stability of the grid. The researchers aim to investigate scalable electrical flexibility services for consumers, business, and distribution system operators in order to postpone or even prevent such low-impact works. This flexibility can be created by shifting the charging time for electric cars or the utilisation of heat pumps to moments when people use less energy or by storing the surplus of energy in batteries when the sun shines bright. Through the development of special algorithms the researchers develop a mechanism which can decide when energy flows which way. For the development of such mechanisms they also include the willingness of companies and consumers to be flexible in their energy flow.
When it is possible to make the grid more flexible the next step is to develop applications that will automate the use and trade of electrical energy in a transparent and fair manner.
The fast electrification of our environment and the connected energy demand in the built environment necessitates electrical grid expansions. Grid congestion management is a key operational tool to smoothen the planning of such expansions and optimise the allocation of scarcely available technicians. This requires intelligent, scalable, and socially acceptable flexibility solutions borne by the complete energy supply chain.
Currently, the existing building management systems of office spaces follow fixed schedules that are ill-adapted to the actual, dynamic use of these buildings. The objective of Brains4Buildings project is to develop smart methods and algorithms to reduce energy consumption while allowing for maximised and customisable comfort. Even in the most modern utility buildings energy is wasted due to malfunctioning installations and unexpected user behaviour. This means that, without requiring physical changes to the building, a significant cost reduction can be obtained by making the building management systems smarter. By adding ‘Brains’ to a building, that is fed by data from smart meters, building management systems and the Internet of Things will be capable to make weighted decisions how to maximize energy efficiency and CO2-reduction, increase comfort, respond flexibly to user behaviour and local renewable energy supply and demand, and save on maintenance costs.
The research is organised around open living labs, that will develop and test the first prototypes of new smart algorithms, methods and interfaces in a protected environment. One of the main challenges, is to develop scalable affordable solutions. Moreover, B4B needs to convince managers of the benefits and start investing and using smart system. Lastly, the end users need to be involved to understand how they want to use their offices, to determine the extent to which building management systems should be adapted while balancing sustainability requirements.
By enabling a smarter use of existing offices and building management systems, energy consumption can be reduced by 20-30% without sacrificing comfort and usability.
Most of the energy we consume at our homes, comes from elsewhere and is mostly used as electrical energy and for heating or cooling. Innovative in this project is cross energy coupling -electrical energy and heatwith innovative way of electrical energy and heat storage. The energy neutral house can generate its own solar energy which can be stored in a battery, connected electrical vehicle and heat. The researchers aim to develop an integral system for the intelligent and integrated control and implementation of hybrid energy storage technologies in the built environment. They are working on making the collective heat supply and the general energy consumption more sustainable. This system of generating and storing energy will be managed through an intelligent management system that can switch between the various energy resources available at the house.
To further the innovation hydrogen storage will be added, more ancillary services for the grid have to be developed with corresponding regulations and business case.
Flexinet contributes to the transition as it demonstrates how existing houses can become energy neutral by having the cross coupling of energy usage, storing the needed power electronics underground and having different energy storing option including heat.
Solar panels are a well-developed technology for capturing renewable energy. One of the biggest challenges for installing these panels is finding enough and the right space to do so, especially in highly urbanized environments. Besides the space or location challenge, there are also a lot of unanswered questions about the stability of the grid when so many panels are installed and the development of green roofs. The researchers aim at developing a model that takes all these challenges into account but that can still create insight in the potential for placing solar panels on roofs. Through 3D modelling, simulations, and calculations on the possible yield in a given geographical area, the researchers have developed a prototype that combines different layers of information through which it can create such insights needed to further accelerate the deployment of more solar panels in cities.
The next step for this innovative idea is to offer a similar service to other cities and grid companies. For this purpose, the start-up company PV Works B.V. has been founded.
The system that is being developed allows municipalities, grid companies or house owners to get insight in the potential for capturing solar energy in an urban environment.
The usage of available renewable energy sources will be critical in the electricity-driven energy system of the future. Photovoltaic (PV) systems can pave every surface in the urban providing useful green electricity for the sustainable electrification of society. For this the researchers are combining expertise in material science; engineering hybrid tandem devices; PV powered multi-functional building elements and xiPV systems. Shade-resilient, high-performance modules integrated with storage of energy and communication capabilities will constitute PV-based intelligent energy agents. This new PV-based intelligent energy agents will also integrate sensing capabilities to identify the operation and environmental conditions. These PV-based intelligent energy agents will cover the whole conversion chain from photons to electrons to bits, marking the advent of photovoltatronics age.
The next step is to start incorporating as much PV systems in building and shaping our environment as possible.
The development of SMART photovoltatronics will enable society to turn as many surfaces as possible in energy capturing sites – generating high energy yields.
Current PV modules are composed of many in series connected solar cells. When part of the PV module is shaded, the shaded cells cannot generate the same current limiting the ability of the PV module to produce power. Parallel connection of the strings of solar cells could solve this issue. However, the drawback of connecting in parallel is that you will have higher losses. So, what if you could make the connections between the solar cells reconfigurable? Depending on the casted shade cast on the PV module, it will implement the most optimal connections between the cells. The researchers aim to do so by making the solar cells and modules smart by means of integrated electronics and novel algorithms. Through special electronics cells and modules can sense shade and take other measures which will help the module to make the optimal connections.
The new module has the electronics in place to reconfigure the electrical connections between the strings and to decide on the best configuration using internal electrical measurements. The next step is to design ad hoc power converters for reconfigurable PV modules and develop new algorithms that can not only increase the maximum energy output but also make decisions that are more oriented to the diagnosis or prognosis of the state of health of the panel to improve the PV module and system safety and facilitate operation and maintenance.
With reconfigurable PV modules more surfaces become suitable for installing PV panels, thus increasing the capacity for capturing solar energy. With this innovation we no longer have to omit partially shaded surfaces. Subsequently, this innovative idea will facilitate integration of PV in infrastructure and create new design opportunities for architects and building/city planners.
With a growing demand for green energy carriers it becomes more important to develop electrochemical systems that convert renewable electricity into useful products and fuels. For that, we need to understand the processes that occur in electrochemical flow cells in order increase the speed of the energy conversions that take place in such cells. The researcher is looking how liquid and gas bubbles can be transported quickly through flow cells such as electrolysers, flow batteries and methods for capturing and converting CO2. He develops new electrolyzers that rely on suspensions, and finding clever ways how the produced energy carriers can be (re)moved or separated. The challenge is to find such clever ways that are not only feasible but also interesting to the market.
With this new knowledge on the production rate in electrochemical flow cells can be enhanced, the next step is to see if these processes can be scaled to practical implementation of the innovation. The research expects to further advance the conversion steps making that process more efficient.
This research will enhance our understanding of the processes in electrochemical flow cells such as electrolysers, for capturing and converting CO2,and storing energy in flow batteries. With this new knowledge such processes can be further optimized and be made more efficient.
Society needs to recarbonize. The researcher is developing new ways to be able to capture & convert CO2 out of the air we breathe. This will create circular carbon. The technology is based on by flowing air containing CO2 through a special washer. The washing liquid will capture the CO2. The CO2 loaded washing liquid is subsequently used as an electrolyte in an electrochemical CO2 conversion process into valuable chemicals. These chemicals could be commodity chemicals such as ethylene and carbon monoxide. The researcher is developing equipment that can execute both steps - capturing & converting – at the same time. Next to the development of this special equipment another challenge is to design it in such a way that the electrolyte remains stable and the machinery is durable and sustainable.
The first system under development start from capturing and conversion of CO2 derived from flue gases. But initial research is going on the extrapolate towards air capture. The capture media is made more selective, due to the much lower concentration of CO2 in air (400 parts per million (ppm); 0,04%) compared with flue gases (4 to 20%). By first focusing on flue gas application creates a clear pathway forwards for implementation on the midterm, while air capture implementation is foreseen for the long term.
The energy transition is linked to many other transitions. One of the important aspects has to do with the question how we can create energy as cheaply as possible – not only economy wise but also taking people and planet into account. This can be achieved if we can capture energy where is its widely available. The electrochemical based conversion process depends strongly on the availability of cost effective green energy. This technology will help in transforming renewable energy (for instance in sun rich countries) into useful high value products that can transported to be used elsewhere.
The researcher focusses on electrocatalysts that are involved in electrochemical conversions that are relevant for renewable fuel production and the electrification of the chemical industry. Current catalysts exhibit high over potentials; meaning you have to invest more energy for the reaction to take place than theoretically needed. He tries to get a better understanding of how electrocatalyst work in order to develop novel catalysts He aims to develop novel stable, selective and cheap catalysts by manipulating the various atoms in catalytic particles. Besides designing catalysts he also aims to develop sequential catalytic processes to produce chemicals for which we currently don’t have an electrochemical process. The major advantage of turning thermochemical conversions into electrochemical ones is that you would only need electricity to run the process at an ambient pressure and temperature, leading to possibly smaller, more energy efficient and safer factories.
The next step for this research is to find the optimal process conditions for the developed catalysts and to subsequently integrate them into an (industrial) reactor for it. For a further scale-up of these reactors the research would benefit from more contacts with industry so they can be applied in a relevant environment.
With the help of mechanistical insights, modelling and theory predictions the researcher contributes to solving contemporary energy problems by developing new electrocatalytic systems and devices.
The energy transition is not only a technological challenge. It is also a societal challenges as the way we produce, transport, store and use energy has to transform. The researchers aim to take the whole value chain into account spurring innovation at different levels at the same time enhancing performance whilst reducing costs of large-scale energy storage systems, based on electrochemical conversion of electricity into molecules for short and long term energy storage. The project focusses on hydrogen production via water electrolysis, hydrocarbon production from CO2 electrolysis and redox flow batteries. With these technologies the project emphasizes that the energy transition is interwoven with the resource transition. It focuses on development of electrodes and membranes, reactor designs, process control and intermittency to integration with industrial processes, and social innovations such as feasible business models and fair governance arrangements.
Everybody feels the urgency of the energy transition. However, since a shift of an entire system is required nobody knows exactly how to do this. RELEASE takes a complete value chain into account, developing technologies, business models and societal implementation is parallel. The goal is to accelerate innovation.
This research contributes to the energy transition as it questions how we can make the transformation that is needed. By taking the whole value chain into account it tries to accelerate technical and social innovation at the same time.
ir. Ibrahim Diab dr. ir. Gautham Ram Chandra Mouli and prof. dr. ir. Pavol Bauer
The infrastructure of trolleybus grids is already part of our urban environment. The researcher is looking at how this grid can become an active part of the city’s electricity network. The into sections divided trolley grid has no single point of origin that electrifies this network. Each section is only being used when a trolleybus passes under it, and is overdesigned to handle the improbable worst-traffic case scenarios. Consequently, the trolley grid is both underutilized and oversized. The researcher is working on creating a smart and more sustainable trolleybus network for the future Through modelling, simulations, and a cluster of measured trolleybus and trolley grid data, the researcher is exploring various functionalities that could be added or linked to this transportation network such as storage, charging of electric vehicles and integrating solar panels and wind turbines.
It would be interesting to look at how In-Motion-Charging buses can fit into this grid as well. IMC buses are buses with a battery on-board that can be charged while the bus is moving, and then operate on batterymode when the bus leaves from under the catenary. They offer the advantages of both trolleybuses and electric buses. Also interesting would be to see if non smart-grid, city loads can be integrated into the trolleybus grid, such as street lighting. In larger cities, research can also be done on the combination of multiple transportation grids such as trams, buses, and metro’s.
Energy neutral flying is a huge challenge. There are many aspects of the aircraft that can be altered or changed in order to make flying more energy. The idea of the project was to design an aircraft that is as energy efficient as possible while being able to fulfill the same function as our conventional aircrafts. This has resulted in the flying V, a highly energy-efficient aircraft design for long distance flights. A completely new type of aircraft with a completely different plane configuration; this aircraft no longer has a tail, the passenger cabin is located in the wing, the aircraft is more aerodynamic and lower structural weight. However, it can still fulfill the same functions of carrying people and freight. A scale model prototype has been built to demonstrate the flying abilities and measure the flight dynamics and control of the aircraft.
The next step is to build an ecosystem Flying V. An ecosystem in which as many stakeholders as possible are engaged to further mature the design of the Flying V. With more industrial support we hope to be able to make some steps towards building a full-scale prototype
With this radically different aircraft configuration an efficiency of 20% compared with flying a conventional plane can be achieved without compromising in functionality. This is achieved by its improved aerodynamic shape and reduced weight.
Electrifying propulsion of huge ships can be done by using fuel cells on board. The researcher focusses on tailoring the system surrounding the fuel cell to the requirements of the maritime sector. The cell should be able to produce the needed power to move or maneuver a ship and be flexible enough to deal with variations in the power level. In this sector fuel cells are expected to run continuously, 24/7 for weeks on end. This raises questions on the wear or fatigue of such a cell. Fuel conversion to hydrogen and electrochemical oxidation of hydrogen take place simultaneously in the cell but how these process take place in in the cell, interact and affect the performance of fuel cells. The biggest challenge is to make these fuel cells smaller, cheaper, easier to operate and control, and more reliable and efficient.
The next step is to develop and validate models of individual components and integrate them to develop and test new system integration configurations and improved control algorithms.
Fuel cells are the next step for sectors in which batteries are insufficient or the direct use of renewable energy is unavailable Shipping, especially the powering of large ships is such a sector. This research contributes to the transition that it makes fuel cells more equipped for the heavy duty that is needed to power a ship. And at the same time they become more flexible to operate increasing their efficiency.
Current aircraft use kerosene for propulsion, which is stored in the wings. When you would want to fly on hydrogen, storage in liquid form within the wings is impossible. In order to store hydrogen onboard, you would need to find space in the fuselage of the aircraft, thereby changing the shape, drag and other specifics of the aircraft. The researchers try to design an aircraft that can carry and fly on both, hydrogen and kerosene as not every airport would have hydrogen available at the same time. In the new design, the aircraft has a fuselage tail mounted propeller with a third engine that can burn the different types of fuels in its dual-fuel combustion chamber. This new type of engine and propeller configuration enhances the efficiency of the aircraft and also allows for steeper take offs and descends.
The current configuration can store approximately 15 to 20% of the total energy in form of hydrogen, thereby reducing the aircraft emissions by around 25%. So for the next configuration, the researchers hope to bring increase the hydrogen percentage gradually to around 50 %, using the multi fuel combustor technology.
Whereas small aircraft can be electrified, this solution is not scalable. So for bigger aircraftas used in civil aviation, an alternative has to be found. With the APPU project – introducing the possibility to fly on an energy mix - allows for a scalable, feasible, producible and economical aircraft that will use hydrogen in a synergistic way along with other innovative technologies and fuels. This will make the aircraft more efficient and will cut emissions significantly.
The transport sector is also expected to make a transition becoming more green in the way it functions and operates. On the energy part the researchers are exploring the techno-economic competition between new fuels over time and the socio-technical impact of policy on the efficient transition to new fuels for green shipping including the conversion to different power trains and smart grids to support this. The project also looks at if most ships will be electrified what type of actions are then needed for balancing the electrical grid. Speeding up the implementation of sustainable energy and enhancing its roll-out on a larger scale is dependent on the development of new digital tools, new market mechanisms and non-technological frameworks. Another important aspect of the project is demonstrating autonomous barges and develop concepts for the autonomous loading and unloading of electrically powered autonomous barges.
The MAGPIE project aims to have zero emission transport in the port in 2050 It develops a master plan for ports to steer this implementation, that will not only be usable by the Port of Rotterdam, but in general by all Ports in Europa and to a large extend also outside of Europe and covers all transport sectors of rail, inland shipping, trucking and ocean shipping and all aspects including production, supply, bunkering and transshipment of cargo.
The project will create demonstration and pilot projects in the living laboratory environment of the Port of Rotterdam. It aims to advance the technological, operational, digital and organisational aspects of energy use in ports.
Sustainably produced ammonia is an interesting alternative to replace fossil fuel to power a ship. However, using ammonia raises a lot of questions related to the ship’s design and operation. The researcher is looking at what the impact of using ammonia will be on the ship. Various aspects are considered.
For the burning of ammonia a second fuel is needed. The researcher is looking into how this second fuel can be freed by coupling the engine with an ammonia fuel cell. The beauty of this coupling is that hydrogen is released from the ammonia in the fuel cell. Moreover the coupling can also be used for dealing with quick variation in power that is needed for maneuvering; an option that is not allowed by fuel cell only power options.
Once we have demonstrated that using ammonia for fueling ships is feasible the next step is to implement this system. For that we need ship designers and engineers that will build these ships and ship owners who are willing to use these ships.
Ships also need to find alternatives for their use of fossil fuels. The energy demand to move a ship is enormous when sailing intercontinentally. This constant high energy demand excludes batteries as an option, resulting in the exploration of energy storage in chemicals that can easily be transported. Hydrogen, Methanol and Ammonia are possible options with their specific pro’s and con’s; ammonia seems to be the best option for large ocean-going vessels. Using these alternative fuels will also require different engines and power plant designs; AmmoniaDrive is one of the most promising candidates.
Developing a robust grid that is fed with fluctuating renewable resources is a major challenge. Additionally, making everything that can be attached to the grid smart enhances the complexity of the integration. It is hard to say what will happen when we electrify our entire society. The ESP lab is a unique facility at the TU Delft campus where all sorts of electrical energy technologies can be researched, developed and demonstrated. There is even a super computer and a control room that can be used for running grid simulations. At the lab different applications or real devices can be added to the (simulated) grid in order to investigate their impact or to train human operators on future scenarios. These simulations help to make the grid more resilient, efficient, flexible, safe, and reliable.
With so many different electrical engineering disciplines gathered in one location the next step is to work on integrating these new technologies, principles, methods, and components to the electricity grid – to make it future proof.
The Energy transition is full of uncertainty – nobody can foresee which technology or development will make the biggest impact. Planning, designing, and operating complex systems requires robust decision making. The ESP lab is a unique facility – a single location where different types and even combinations of innovations can be researched, experimented and tested. Innovations that need to hit the market quickly to accelerate the change of our grid and the way how we produce, distribute and use electrical energy.
The 24/7 energy lab is located at The Green Village at TU Delft campus. At this site all components that are needed to solely use renewable energy for everything that you would do at home is integrated into one experimental energy system. This house has no cables or pipes that connects it to the external grid. It will demonstrate that with the developed technologies it can function totally autonomous. With PV panels renewable solar energy is converted into electricity which can be used either directly or can be stored in batteries and/or as hydrogen. Subsequently the hydrogen can be used in a fuel cell to generate electricity when there is not enough sun or wind available. This basic set up of the autonomous energy system can be extended with other technologies and devices over time.
With the realization of the 24/7 energy lab the next step is to learn from what happens when different types of technologies and devices are integrated or connected to the site. Users, construction companies, legislators, municipalities, grid companies and researchers will work together to learn and create new knowledge about how this sustainable energy system can be realized and will function. This learning process will raise new research questions about how to design and expand the system and how it works in a real-life working setting. This will also include legal, socio-economic and governance aspects.
The realization of this site contributes to the energy transition by demonstrating the integration of the various renewable energy conversion and storage components on residential level. By making households and/or residential areas energy autonomous, fully relying on renewable energy sources, the pressure to expand or replace the underground local grid with thicker cables will be reduced.
24/7 energy lab: A local, CO2-neutral energy system for the built environment
dr. Phil Vardon, prof. dr. David Bruhn
dr. Susanne Laumann, dr. Lora Armstrong, prof. dr. ir. Kees Wapenaar, dr. ir. Guy Drijkoningen, dr. Auke Barnhoorn, dr. Hemmo Abels, prof. dr. ir. Evert Slob, dr. Denis Voskov, prof. dr. ir. Jan Dirk Jansen, dr. Maren Brehme, dr. Tobias Schmiedel, dr. Kees Weemstra, dr. ir. Deyan Draganov, dr. ir. Martin Bloemendal
TU Delft Campus Real Estate, Hydreco Geomec, EBN and Shell Geothermal.
Geothermal energy is a fairly well developed technology but there are still many unknowns. The construction of a deep geothermal well serves to do further research into geothermal energy and to supply part of the buildings with low carbon heat. This deep geothermal project will have two wells of 2 to 2.5 km deep. The walls of the wells will be lined with different materials transforming them essentially into a plumbing system to transport warm water upwards and cool water downwards. Each of the wells will be fitted with a lot of sensors over the complete length of the wells and in monitoring stations at the surface. Through the sensors the researchers can gather data on how the wells interact with the environment. This project is unique as it will be constructed in an urban environment. This will generate valuable knowledge about how future geothermal projects can be done in other urban locations.
The current plan is to start installing the wells in 2022. There are a lot of remaining research questions that the researchers want to address with this project on site and several PhD projects are underway. A subsequent step is to look into high temperature heat storage so that heat that is brought to the surface when it is not needed, i.e. during the summer, can be stored to be used at an another moment in time (e.g., in winter).
Part of the Dutch mission for the energy transition is to create more geothermal wells. To better understand such systems will allow more efficient and more reliable use of the geothermal resource, without unwanted impacts. Geothermal energy has the potential to contribute several tens of percent of the heat demand, and will need to be constructed and operated in urban environments to effectively deliver heat to where it is demanded.
The potential for capturing renewable energy at sea is huge. Floating energy devices – wind turbines, solar panels, wave energy convertors – are key enablers to harness renewable energy in deep sea. However, they combine many different research disciplines that need to be addressed in a coupled way to efficiently design and engineer the next-generation of floating offshore renewable energy systems. The Floating Renewables Lab brings together unique facilities and researchers in different fields to achieve this goal. A number of facilities will be connected virtually using a so-called ‘hardware-in-the-loop’ set-up. This bridges physical experiments with numerical modelling. Complex aerodynamics, floater stability, sea-keeping system, and even storage and transportation of energy are interesting challenges that can be addressed at the Floating Renewables Lab. Through the combination of these facilities and expertise, the engaged Principle Investigators aim to accelerate floating renewable innovations, research and education.
The first step is to virtually connect and upgrade all the facilities that play a role in developing floating renewable energy at TU Delft. The next steps are to build new facilities – including an extension to full scale measurements at sea.
Offshore renewable energy harnessed by wind turbines, solar panels, and wave capturing devices offer great potential. However, eighty percent of our oceans are too deep to economically mount these devices on the seabed. A solution is to place them on floating support structures. The beauty of making all these devices float is that they can also be located much further out the coast. Additionally, they can also be used to convert renewable energy into an alternative energy carrier – such as H2. Floating offshore renewables will thus be a key enabler to make Europe climate neutral by 2050.
At the Heat & Power lab the research is focused on enhancing efficiency of processes of how energy in the form of chemical bonds can be converted into electricity or power capturing and putting as much of the released heat to good use. This power can be generated in all sorts of ways; for instance through using fuel cells for conversion hydrogen or ammonia. All these reactions have in common that part of the energy is turned into heat instead of 100% electricity. At the lab they design components that can capture this heat and use that energy too instead of letting it go to waste. Another venture for the lab is that they look into how fuel cells can burn cleanly. Combustion processes use air instead of pure oxygen you also emit nitrogen oxides and that needs to be removed afterwards. They experiment by building components or systems that can perform or enhance the reaction under scrutiny.
The next step is for industry to include, embed and integrate the developed system components to increase the efficiency of their processes. Another next step is to develop components that are allow direct air capture – building components that can directly capture CO2 and convert it into power.
The components that are being developed at the Heat & Power lab not only enhance the effectiveness of the process for which they are being developed. They also increase the efficiency of the process with the given context in mind.
prof. dr. Bernard Dam, ir. John Nijenhuis, dr. A. Gangoli Rao, prof. dr. H. Geerlings, dr. W.G. Haije, prof. dr. W de Jong, dr. L. Jourdin, dr. R. Kortlever, prof. dr. A. Ramirez Ramirez, dr. D.A. Vermaas, prof. dr. M. Zeman, dr. M. Alimoradi Jazi
The e-Refinery Institute combines researchers from across the university to work on developing processes and devices to produce fuels and base chemicals from water, air and electricity without the use of fossil resources. For these processes and devices to replace the current operations, they have to be demonstrated at a relevant scale. The e-Refinery Centre is going to be a place where researchers investigate the scaling of various new processes under relevant scale and conditions - for instance for the renewable production of ethylene; one of the main components for the production of plastics.
Through the realization of a e-Refinery Centre the researchers can learn to, experiment and gather new knowledge about how e-refinery installations, set-ups and processes are to be scaled. This centre will facilitate many innovative ideas to bridge the so called valley of death and reaching the demonstration phase instead of doing only simulations that show that it can work on a bigger scale.
The e-Refinery Centre will contribute to the transition as it is expected to accelerate innovation of current industrial processes. Process that currently require fossil resources will be replaced by e-refinery processed and tools making our industry more sustainable.
The TPM Energy transition lab is more an institute which aims to capture and connect all the different types of social science expertise that is being furthered and developed within the TPM faculty focused on the energy transition. At this lab the researchers are trying to quantify and model different kinds of human aspects related to the energy transition. They are building models predicting how people are going to behave in order to create insight in what this means for society. Together they are developing new approaches, methods and tools for fostering an effective fair and legitimate energy transition. Their biggest challenge is to model human behaviour in such a way that it does not violate the richness of this behaviour and to be able to create insight into what is fair for the energy transition.
Besides creating more insights for the energy transition a next step for the Lab is to contribute to building a (systems)theory that will capture more of the psychological side of how a system works or can be changed. With the creation of the valuable insights for the transition the researchers also hope to stay at the forefront of their research field attracting more excellent researchers and stimulating or facilitating interdisciplinary research and education.
Our energy system cannot be transformed by simply changing and replacing current ways of doing things for new technologies. Reality is much more complex as also policies, laws and regulations, people’s behaviour and opinions – depending on which stakeholder they represent - play a role. The TPM energy transition lab brings together various types of social science expertise to create insights into the complexity of the transitions and decisions that need to be made.
Currently, the intermittency of renewable energy generation makes it difficult to meet the society’s energy consumption needs. Batteries and electrolysers only offer partial solutions. Integrating the two gives a special flexibility: it creates an electrolyser that can switch as fast as a battery, and a battery that cannot be full.
The integrated Battolyser System provides a balancing power in the electricity market as well as hydrogen for fuel and feedstock. It stores electricity from the grid at low prices when there is a surplus, turning it into hydrogen when the battery is already full, and sells electricity when there is a shortage, at a higher price. This creates a brilliant and unique business model for Battolyser System’s clients.
The Battolyser System is currently experimenting with demonstrators, and is working on increasing its scale to provide Megawatt capacities. To do so, they currently grow their team for which they see much interest even in the growingly competitive market.
A Battolyser System balances the electricity grid by delivering energy at a high price to the grid when there is a shortage; and by cheaply buying energy from the grid for battery capacity charging and hydrogen production when there is a surplus.
Nice!
How’s the wind up there?
Awesome! I am blown away by all this free energy!
The transition to a 100% renewable energy system will require unprecedented amounts of natural resources and financing while also impacting the environment in a major way. Using lightweight membrane kites to harvest wind energy, Kitepower technology can reduce the material effort to up to 10% of conventional wind turbines while substantially decreasing the environmental footprint and accessing stronger and more persistent winds at higher altitudes. The unique implementation combines a large inflatable membrane kite, a suspended robotic control unit, a tether, and a ground station with an integrated drum-generator unit. Energy is generated in pumping cycles, alternating between energy-generating reel-out phases, during which the kite is operated in crosswind maneuvers to maximize the tether force, and energy-consuming reel-in phases, during which the kite is depowered, and the crosswind maneuvers are stopped to minimize the tether force. A key challenge is the full automation of the operation, increasing reliability and robustness.
Kitepower is developing a 100 kW system based on a 60 m2 kite. Having teamed up with energy giant RWE and a large consortium of European partners, the company has started long-term testing of the 100 kW system in Ireland. Following its market introduction, the envisioned next scaling level is 500 kW.
An immediate contribution is the systematic replacement of Diesel generators by Kitepower systems. The technology is a perfect complement in hybrid power plants to increase the capacity factor and annual energy production.
These joints are much stronger and these structures can be build with 60% less steel.
Yeah, and no more suffering from poor fatigue performance as a welded connection would.
Composite joints replace traditional weld in multi-membered structures such as a Jacket foundation for offshore wind. It is a gamechanger in construction as it transfers loads utilizing composite material placed strategically at location to create the optimum load transfer in a joint. This joint is thereby used the best of two worlds: Steel for its excellent stiffness and composite for its excellent fatigue resistance. When applied in heavy loaded offshore structures it reduces the amount of steel in a structure up to 60%. The biggest challenge for this technology is the acceptance of an innovation by and in the risk-averse market of Offshore wind. That is why we work closely with the supply chain and project developers.
Since 2017 over 150 joints and 1000 material specimens have been tested and in 2023 full scale joints where tested and confirmed the maximum and fatigue capacities are in line with prediction models. Next steps are to finalize the qualification of the technology in 2024 and the focus lies on industrializing the production method to accommodate the volumes of offshore wind and introduction to the market by Pilots and scaled introduction of the technology.
We propose a solution that increases the manufacturing capacities of existing yards by a factor 2 that supply structures such as Jackets and floater for offshore wind. At the same time we realize offshore wind foundations with up to 60% less materials, that generate 40% less CO2 and that have a superior lifetime of 5 to 10 times compared to current state-of-the-art welded offshore wind foundations. This will strengthen the supply chain in their challenge to increase their capacities and accelerate the deployment of offshore wind.
You’re obvious new in the neighbourhood!
We can show you around if you’re interested?
Lobster uses advanced robots to photograph the seafloor in order to support offshore wind developers, consultants and government to understand the undersea environment and make informed decisions. The greatest challenge in this field is to take photos in a consistent manner despite complex, turbid or dynamic conditions underwater. This has to be done at a scale relevant to emerging concerns regarding offshore energy development. Our robots are innovative as we achieve great performance at minimal hardware cost through vertical integration, and design autonomous control systems to ensure consistency across the data. This unique approach enables us to deliver data very cost-effectively. Other providers of seabed data on the market are reliant on equipment suppliers and their subcontractors to innovate, which makes them unable to quickly respond to the emerging market for large scale, high resolution seabed mapping. Currently, we are focused on working with clients in the offshore wind and research sectors, helping them to track changes in marine ecology and to comply with environmental regulations. The benefit for our clients is the acquisition of accurate data that can assist in the planning and monitoring of their projects, without the need for extensive offshore personnel, thus saving them additional time and resources.
Next, we plan to expand our pilot projects, particularly in the offshore wind sector, to fine-tune our robotic technology based on real-world feedback. Our ultimate goal is to establish our position as a reliable partner in various maritime sectors.
Lobster Robotics helps set up green energy projects responsibly by offering clear pictures of the sea floor. This way, we make it easier and safer to build things like wind farms at sea.
Professional fleet owners are experimenting with pilots to electrify their fleets. Ensuring that vehicles are always charged sufficiently to run the business’ operations is the major bottleneck. Connecting the vehicle to the charger is only a small act, but it comes with a huge responsibility. If the vehicles fail to charge, the entire schedule of the next day needs to be reworked. Moreover, when transitioning to autonomous vehicles, the necessity of someone to plug in the charger, makes the entire process once more reliant on humans. Therefore, Rocsys (Robotic Charging Systems) came up with an autonomous charging system that relies on three technology pillars: soft robotics, computer vision and cloud connectivity. The soft robotics ensures robustness and safety when vehicles move e.g. while cargo is loaded, or when employees accidently bump into the robot. Through cloud connectivity, Rocsys integrates with planning software, which ensures all vehicles are charged sufficiently according to their schedule.
Rocsys is mainly focusing on improving the algorithms that the computer vision uses to detect the socket, so it can deal with all kinds of weather conditions. This outdoor testing is necessary to build credibility about the reliability of the automatic charging system. Moreover, Rocsys is exploring solutions to allow multiple vehicles to make use of one charger, by automatically removing the charged vehicle. Lastly, it is preparing for broader use case scenarios, for example for autonomous driving in public spaces – where there is a higher unpredictability, lack of uniformity in vehicles and more safety concerns.
Rocsys enables fleet owners to adopt electric transport on a large scale, with the reliability and robustness that is required for a professional fleet.
Buying solar panels is a big decision for people due to the financial investment, complexity of the jargon and distrust of installers. To address these challenges SuperSola developed a plug & play solar panel. Sockets are capable of power input as well as output so this solar panel has a cable which you just plug into a regular socket feeding power to the grid. Moreover, the solar panel does not need to be installed by a professional. It is simply placed on a flat surface. Because they are not fixed to the roof, there is no risk of leaking and it enables taking the solar panels with you. Supersola decided to focus on flat roofs to ensure safety for its users during installation, and because its standalone panels can easily be used on small surfaces such as garden sheds.
Supersola is available on the market and is expanding to other countries. Because plug&play solar panels are quite unknown, awareness of consumers is still lacking, and legislation is lagging. Supersolar is working on building awareness for their innovation. Next to geographical expansion, they are also looking at ways to diversify their product, for example to make them suitable for use on campside and boats (off-grid).
Make solar energy available to everyone by making it a standalone and plug & play system. This makes it more affordable and easy to use.
Sustainable solutions for heating our homes are often not powerful enough to replace gas boilers. Most residential heat pumps have low power and low efficiency at high temperatures. To warm an entire house extra insulation and low-temperature heating systems are needed. Moreover, a heat pump requires a noisy outdoor unit and uses harmful refrigerants when released. Tarnoc sets out to be a one-on-one replacement for the classic boiler. Their turbine heat pump is an open system without refrigerants. It draws in air through a centrifugal compressor and releases the heat inside the home through the existing radiator system. The energy from the compression is recovered in the turbine and is converted back into mechanical energy, to power the compressor. Through these cycles it is possible to create high temperature heating with a large capacity in a relatively small installation.
Having won multiple awards, Tarnoc is currently piloting its turbine heat pump in different locations. They will do more pilots with big housing corporations and they will place an installation at The Green Village.
When switching to a fully electric system one has more control over the environmental impact, as green energy can be used to operate Tarnoc.
Hydrogen is widely accepted as a promising technology to replace fossil fuels. However, it is challenging to store hydrogen, requiring high pressure, cold temperatures or e.g. ammoniac.
H2Fuel provides a way to produce, store and release hydrogen in powder form under atmospheric conditions. Hydrogen is produced in an exothermal reaction by adding ultra-pure water to sodium borohydride (NaBH4), and does not require additional energy. Once the hydrogen has been issued, the residual substances can be returned to the powder state with hydrogen stored in them: this makes H2Fuel the world’s first circular fuel. By storing hydrogen in a powder, it does not need to be connected to the electricity grid. It can therefore replace generators in rural areas, or provide energy on location.
H2Fuel is looking to license its patented technology, or sell it to the right third party.
Enables the wider use of hydrogen by making it easy to produce, store and release hydrogen under atmospheric conditions and on off-grid locations.
October 2023
Production:
TU Delft | Innovation & Impact Centre
Text & Editing:
Susanne Sleenhoff
Leonie Levrouw
Jurjen Slump
Friso Lippmann
Malou Spruit
Cartoons:
CVIII Ontwerpers – Erwin Suvaal
Infographic (page 6/7):
Iris Jönsthövel
Design, Layout
lizzil creative
About the cover:
The flame is an important symbol for the University. It refers to the Myth of Prometheus – bringing the flame from Mount Olympus to the people enabling progress and civilisation - who is sometimes considered as the first engineer. Here Prometheus meets Benjamin Franklin - who is capturing electricity with a kite – who want to encourage him that we can make the energy transition happen.
Research at the Delft University of Technology is characterised by its inter- and multidisciplinary thinking spread across science, engineering and design disciplines. With ground breaking research we intent to make significant contributions to a sustainable society.
Aiming to enhance collaboration with external partners this booklet presents a small selection of ideas that are being developed in Delft.
www.tudelft.nl/innovation-impact