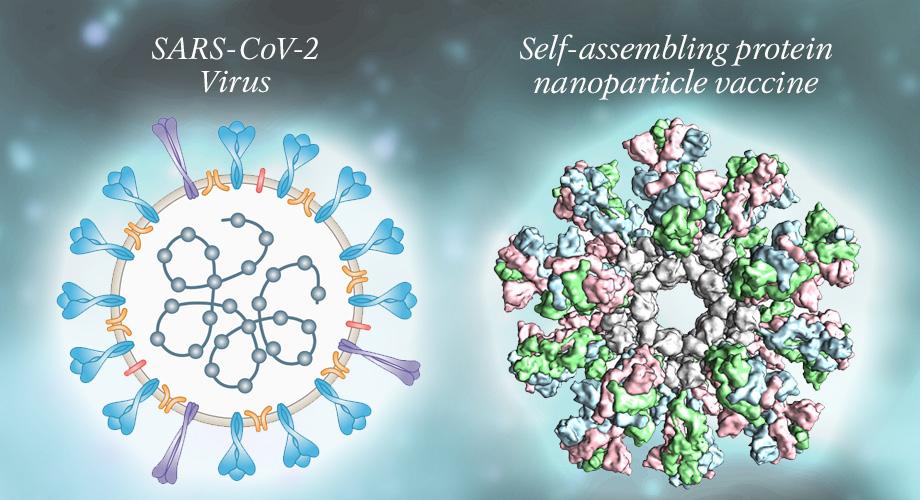
4 minute read
NANOPARTICLE-BASED VACCINE DESIGN
By Eva McCord and Teresa Nam
In the realm of biosynthetic pathways, as well as within the underlying mechanisms that govern molecular interactions, much of the complexity that determines successful signaling can be reduced to one trait: shape. Ranging from the weaving alpha helix to the microtubule lattice to the seemingly simple formation of a dimer from two compatible monomers, a molecule’s shape and structure doesn’t just provide researchers insight into the molecule’s behavior. Experimenters and scientists can capitalize on these molecular attributes and make crucial inferences regarding how the molecule plays a role in its greater cellular environment. By extension, this same principle of shape itself determining the nature of a molecule can be applied to engineering-centric solutions for some of the greatest battles in healthcare, namely the use of nanoparticles as anticancer drug delivery systems. Based on recent findings from Xu et al., shape—with specific emphasis on chirality—can be capitalized upon in order to create “nanoparticle enantiomers,” or otherwise identically-structured nanoparticles that yield vastly different immune responses. But amidst other experimental, design-driven approaches to the ever-present demands of modern healthcare, it is important to recognize and disseminate knowledge regarding the importance of developments in nanoparticle technology. In doing so, more and more individuals may contribute to conversations pertaining to the socioeconomic tapestry of healthcare and medicine itself, and how these miniscule structures may enable advances in patient care previously thought impossible. In order to properly understand the impact of the Xu team’s discovery, a clear definition of what nanoparticles even are must be established. With the rapidly-evolving and ever-expanding realm of biotechnology already a somewhat nebulous field in the minds of the general public, simultaneously introducing the nanoscale may prove daunting, as well as cause understandable confusion. How are nanoparticles created? How do nanoparticles work? What makes nanoparticles distinct and separate from other therapeutic methods already employed in both clinical and research settings? Nanoparticles can be succinctly summarized by two key components: size and shell (and now shape, of course!). These devices operate on the very same scale as the actin and tubulin monomers that assist your cells through mitosis, the DNA helicase and ligase that oversee DNA repair, and the hemoglobin carrying oxygen throughout your body. However, the most interesting aspect to nanoparticles is not necessarily their size—which is implied in their name—but their shell-like structure. Often designed in the shape of a sphere, the outer surface of the hollow “nanoball” is coated in a specially-chosen biological coating; this coating allows for the nanoparticle to interact with both its general environment and specific molecular targets, and ultimately facilitates the particle’s overall biocompatibility. This shell houses the “core particle,” which in medical applications is often the drug the nanoparticle had been created to deliver. A helpful analogy to better visualizing nanoparticle structure would be to liken the nanoparticle to a biological Trojan Horse; the outer shell of the nanoparticle allows the particle to freely interact and associate with its target tissues, only for the inner core to pack a surprise, nano-scale punch. And while the answer may be intriguing to some, yet dissatisfactory to others, nanoparticles can be applied to an incredibly vast array of medical challenges and pursuits, ranging from the aforementioned drug and gene delivery to full-scale tissue engineering and tumor destruction (Salata). Within these interdisciplinary applications that the genuine beauty of nanoparticles is revealed, along with the overarching motivations to fuel and support innovation within bionanotech.
Advertisement
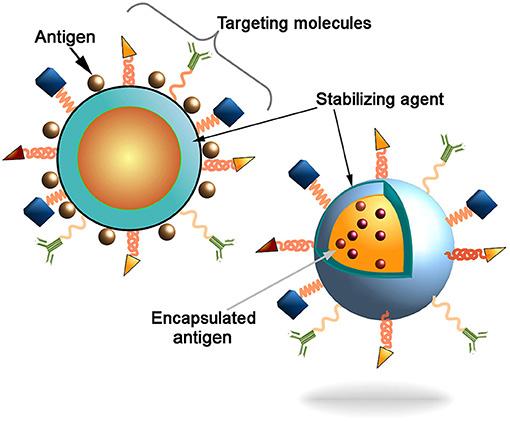
Pivoting back towards the work of Xu et al., while bioengineers have long understood the aforementioned importance of size and structure, the shape of nanoparticles—and the degree to which this shape influences a nanoparticle’s efficiency—had been only vaguely understood. Through the creation of a tunable system using circularly polarized light, Xu et al. was able to directly control and adjust the asymmetry of left- and right-handed enantiomers of gold nanoparticles (Xu). In doing so, the chirality of the nanoparticle could be directly linked to the strength of immune cells in mice, with left-handed enantiomers found to produce an immune response twice as strong as their right-handed counterparts (Hooftman). This significant deviation in performance was found to be due to the left-handed nanoparticles exhibiting a greater binding affinity for specific receptors within the immune cells themselves, suggesting that the enantiomers of gold nanoparticles at large could be used as immune system stimuli (Hooftman). This additional stimuli, thus, may improve the efficacy of a vaccine. This was later proven by Xu’s team after they discovered that the left-handed enantiomer was more effective at triggering antibodies against the influenza virus than not just the right-handed enantiomer, but alum—the conventional substance utilized to enhance the body’s immune response to the influenza virus.
While these results seem alltoo good to be true for biomedical researchers and bioengineers alike, seizing on this moment of promise also provides a crucial window for researchers to discuss not just how they can improve the quality of treatment, but the quality of care delivered to patients. In other words, questions such as: How do nanoparticles play a role in the socioeconomic design of healthcare and medicine? How do advancements in learning how to make them more efficient and effective impact patients and care accessibility? There is no time more relevant or pressing than today, following a period of rapid vaccine development and subsequent discourse regarding the ethical and moral faces of vaccinology, to discuss the future of clinically-applied nanoparticles. There emerges a distinct “fork” in the conversation of nanoparticle-driven global healthcare, split between the creation of life-saving, non-invasive treatments for the world’s most in-need and vulnerable populations and the threat of a “nano-divide” (Salamanca-Buentello and Daar). This nano-divide, in which wealthier nations could isolate and restrict access to nanoparticle treatment from nations lacking the same financial and medical means, would simply serve to further aggravate and amplify the already-present fragility and power imbalances that define these global relationships. In saying all of this, it is important to keep an eye to the future of nanoparticle-driven care, particularly as it runs parallel to the future of medicine at large. By identifying what qualities and characteristics are most indicative of nanoparticle effectiveness—and by deducing how these characteristics can be made tunable—engineers and healthcare workers alike will be able to collectively reduce the toxicity burden faced by patients undergoing intensive immunotherapy. Likewise, with nanoparticles being comparatively non-invasive alternatives to surgery, supporting advances in nanomedicine are directly conducive to making necessary, life-saving opportunities accessible to more patients than ever. Ultimately, while Xu et al.’s findings stand as just one of many critical junctures on the path to sparking dialogues around nanomedicine, these efforts assist in making nanoparticles “seen” in the eyes of both healthcare workers and the general public—no electron microscope necessary.
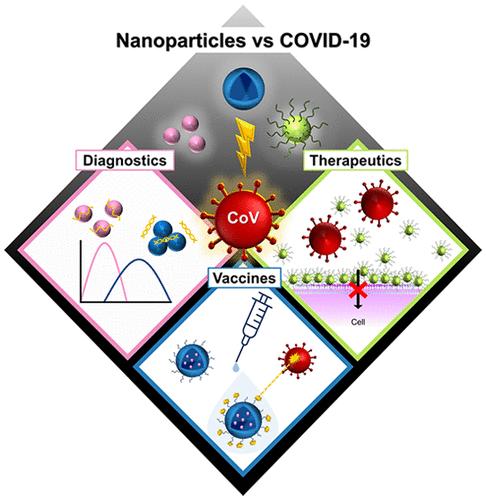
Credits: https://www.nature.com/articles/ d41586-021-03806-7 https://www.nature.com/articles/ d41586-021-03806-7 https://jnanobiotechnology. biomedcentral.com/track/ pdf/10.1186/1477-3155-2-3.pdf https://www.nature.com/articles/ s41565-021-00899-z