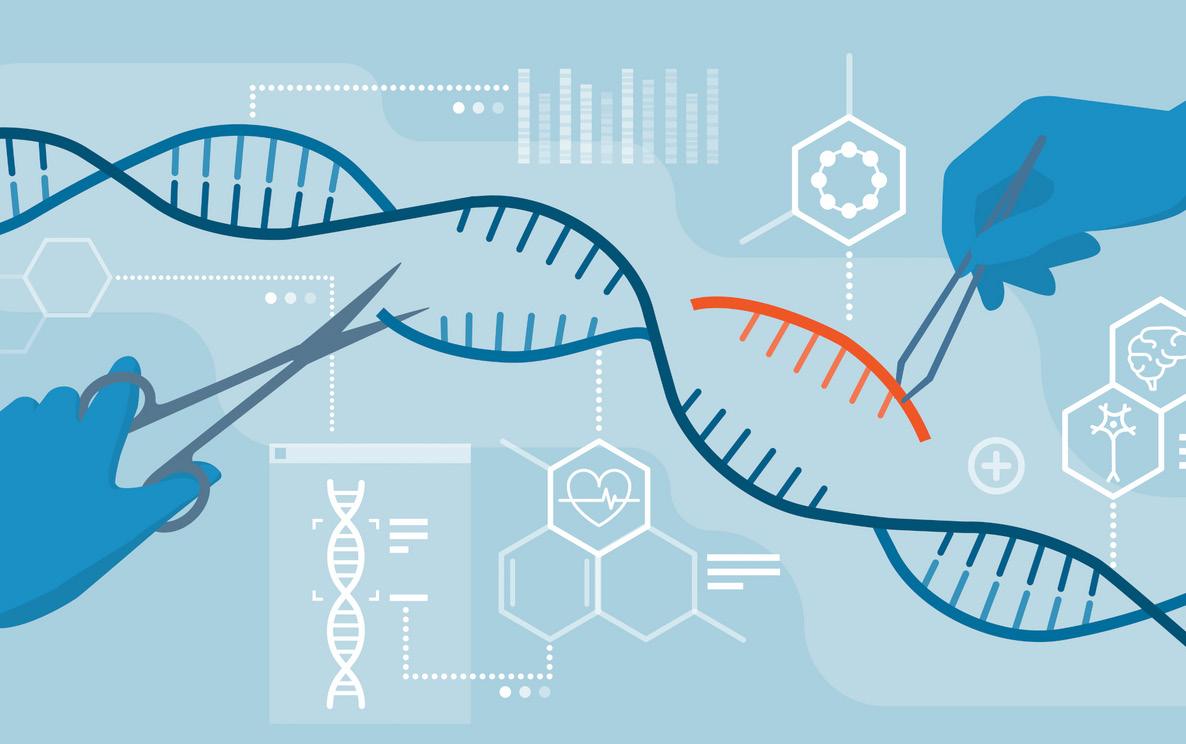
8 minute read
HUMAN GENE EDITING: WHEN TO SAY STOP?
by LIZZIE HUFFAKER
Lizzie Huffaker is a second-year Philosophy and Astrophysics major at the University of Chicago. She loves investigating the intersection of science and the humanities, both in her studies and outside of school. Outside of SISR, Lizzie is a Co-Music Director of the Ransom Notes, one of UChicago’s competitive a cappella groups, and plays bass in the Symphony Orchestra. She also loves baking, watching shows with friends, and her weekly Trader Joes run.
Advertisement
The field of gene editing is one of great controversy, both promising miracle-esque solutions to human diseases and presenting great ethical dangers. What if scientists could edit human genes to eliminate cancer? To cure blindness? To increase intelligence or athleticism? To build the “perfect human?” The potential is incredible— and incredibly frightening.
Our debate began in the years following the Holocaust, when the field of “bioethics” began to emerge, born from an urgent need to prevent the atrocities, specifically those emerging from genetics being used to support supremacist ideologies in WWII, from happening again. Though the prospect of directly editing a human gene seemed fantastical at the time, a new era of eugenics was on the horizon, and scientists, politicians, ethicists— and even the public— were ready to get involved.
Around the 1950s and 60s, there was a shift in ethics ideology, led by both scientists and politicians who wanted to defend the field of eugenics while still distancing themselves from the German eugenics that led to the Holocaust. A leader in this movement, biologist Robert Shinseimer, argued that desirable human traits depended not on race or ethnicity, but on intelligence, strength, and virtuosity, which incentivized scientists of the age to discover a technique to directly manipulate the genetic composition of humans.1
Although scientists made little progress towards this goal in the late 1900s, the debate continued regarding the moral implications of human gene editing. Around this time, geneticists made an important distinction between somatic and germline editing. Somatic editing, also called “somatic gene therapy,” refers to the manipulation of an individual’s non-reproductive cells during their lifetime in a way that doesn’t affect their reproductive cells. On the other hand, germline editing affects the reproductive cells of a person, with therefore longer-lasting effects, impacting not only the manipulated individual, but also their future children. Generally, the eugenicists of the 20th century were concerned with germline editing as a means to affect the entire human race, aiming to “edit out” mental and physical disabilities, as well as susceptibility to diseases such as HIV.
Opponents of eugenics argued for restricting human gene editing to purely somatic purposes, given the longevity of the unknown consequences in the case of germline editing. They believed that if we allow for germline editing, it becomes harder to set a moral boundary. This belief won out for most of the 20th century and even into the early 2000s, primarily because the public itself was so invested in creating clear restrictions on this kind of research, especially as the prospect of actual genetic manipulation became increasingly realistic. For example, one prominent figure opposing eugenics was the Jewish philosopher Hans Jonas, whose experience escaping Nazi Germany shaped his later bioethical beliefs in regard to eugenics. He had such a big influence on the field of bioethics that he convinced George W. Bush’s Council on Bioethics to retract federal funding from stem cell research in 2001.2
However, most could not deny the long list of potential benefits to such research, and in 2005, zinc finger nucleases
(ZFNs) entered the biotechnology research scene as a new method of selecting and copying strands of DNA to an unprecedented level of accuracy. ZFNs, along with transcription activator-like effector nucleases (TALENs), discovered in 2010, work together to cut two strands of DNA at a precise point, triggering an alarm system which forces the DNA to repair itself. Scientists can then manipulate the DNA to repair itself in a new way, without the target gene. However exciting and effective this discovery was, the process of designing and synthesizing the ZFNs and TALENs proved to be lengthy and expensive, making it an unrealistic option for future use.3
As the field of biotechnology flourished in the early 2010s, the issue of bioethics fell away from the public sphere as other hot-button issues like abortion became more prominent.4 This allowed much of biotechnology research to go uncontested, paving the way for the introduction of CRISPR-Cas9 in 2012.
The story of CRISPR, or “Clustered Regularly Interspaced Short Palindromic Repeats,” actually begins much earlier in 1987, when Yoshizumi Ishino discovered an intriguing phenomenon in his laboratory.5 The Japanese student was sequencing a gene in E. Coli bacteria when he noticed an unusual pattern of 29 repeated base pairs of genes separated by unique DNA. This seemingly small observation proved revolutionary in the world of genetics, as scientists continued to research CRISPR in the late 1900s and early 2000s. This phenomenon works in tandem with Cas9, a dual RNA-guided protein, meaning the Cas9 enzyme is guided to a select strand of viral RNA and bound to that strand using another piece of RNA, resulting in the problematic strand being cut out. Once combined with the Cas9 protein, CRISPR would change the field of genetics forever. The revolutionary part of CRISPR-Cas9 is the way the DNA repairs itself: once the Cas9 has cut out the target strand of DNA, it can receive a “suggested” replacement strand to repair the damages, which can be manipulated by scientists to contain any number of variations on the original strand. This provides an efficient and effective way of editing certain strands of DNA.
Regarding safety, the introduction of CRISPR-Cas9 reduces much of the previously held worries surrounding gene editing and specifically germline editing. While the CRISPR-Cas9 technique does still pose the safety risk of long-term consequences from unintentional gene edits, the level of precision at which it can operate is incredibly promising for the future of gene editing and especially for the increased safety of gene therapy operations.
The development of CRISPR-Cas9 revitalized the otherwise dormant debate on the use of biotechnology for somatic and germline editing practices, this time with a new urgency as many saw the previously dystopian (or perhaps utopian, depending on who was asked) landscape becoming an ever-nearer reality. The primary justifications for setting the boundary at somatic vs. germline editing in the mid-1900s were for both safety and maintaining autonomy. Safety was a concern at the time because the field of human gene editing was still mostly foreign, and many were worried about the unknown long-term consequences of germline editing. Autonomy was relevant because while somatic editing allowed for knowing consent from the altered individual, germline editing would affect those who did not have the option to choose. However, as technology improved and the socio-political landscape shifted, these justifications became harder to defend as reasons to hold the somatic/germline border.
Though the argument of autonomy was originally claimed by the anti-germline side of the debate, the ruling of Roe v. Wade in 1973 and the growing relevance of the abortion debate changed the moral landscape and forced many in the bioethics community to reconsider how they used autonomy to justify the constraint on human gene editing. While proponents of “consent of the altered,” argue that germline editing is wrong because those potentially affected cannot consent, a new argument arose that a couple should have the autonomy to choose whether their own reproductive cells are edited. As expected, this debate continues to prevail in the bioethics community as the question of abortion does in the socio-political sphere.
CRISPR-Cas9 is one large step in the field of genetics, presenting both inspiring results for curing diseases and frighteningly long-lasting consequences. Now, the task remains to continue refining this research while maintaining an open discussion about the ethics of the practice. This incredible potential to better human medicine cannot be ignored, and it has motivated bioethicists to work on redefining the boundary of what is “ethical” in gene editing.
References
1. Evans, John H. “Setting Ethical Limits on Human Gene Editing after the Fall of ... - PNAS.” Institute for Practical Ethics, 22 Sept. 2020, https://www.pnas.org/doi/10.1073/pnas.2004837117.
2. Bush, George W. “President Discusses Stem Cell Research.” National Archives and Records Administration, National Archives and Records Administration, 9 Aug. 2001, https://georgewbush-whitehouse.archives.gov/news/releases/2001/08/20010809-2.html.
3. Luthra, Ritika, et al. “Applications of CRISPR as a Potential Therapeutic.” Life Sciences, Pergamon, 25 Aug. 2021, https://www. sciencedirect.com/science/article/pii/S002432052100895X?via%3Dihub.
4. Gabel, Isabel, and Jonathan Moreno. “Genome Editing, Ethics, and Politics.” AMA Journal of Ethics, U.S. National Library of Medicine, 1 Dec. 2019, https://www.ncbi.nlm.nih.gov/pmc/articles/PMC7001785/.
5. Ishino, Yoshizumi. History of CRISPR-Cas from Encounter with a Mysterious Repeated Sequence to Genome Editing Technology. Journal of Bacteriology, 1 April 2018, https://journals.asm.org/doi/10.1128/JB.00580-17.