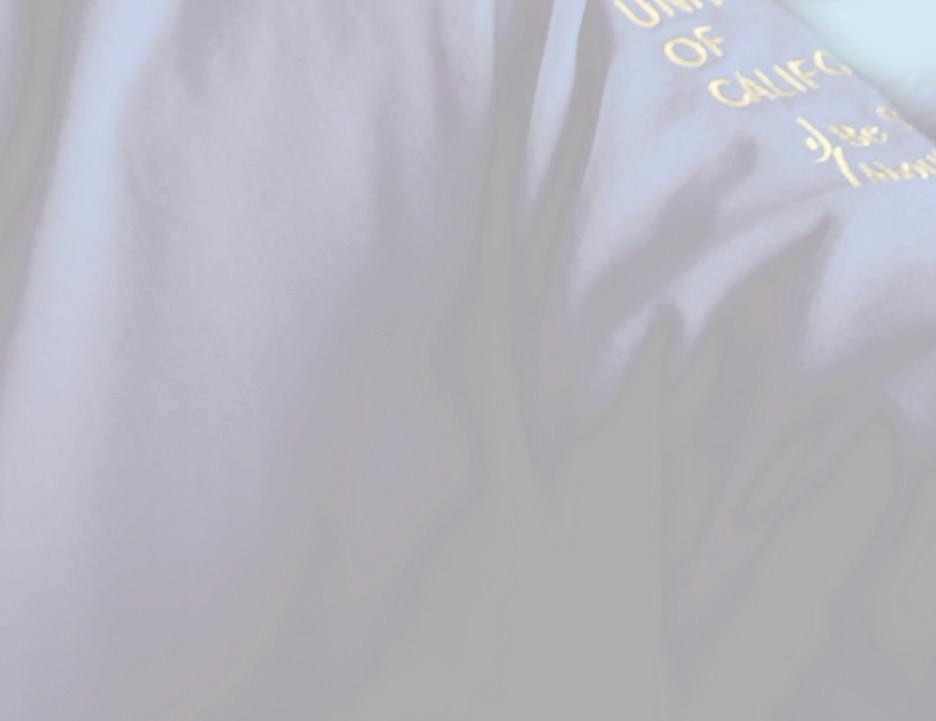
19 minute read
Research & Funding
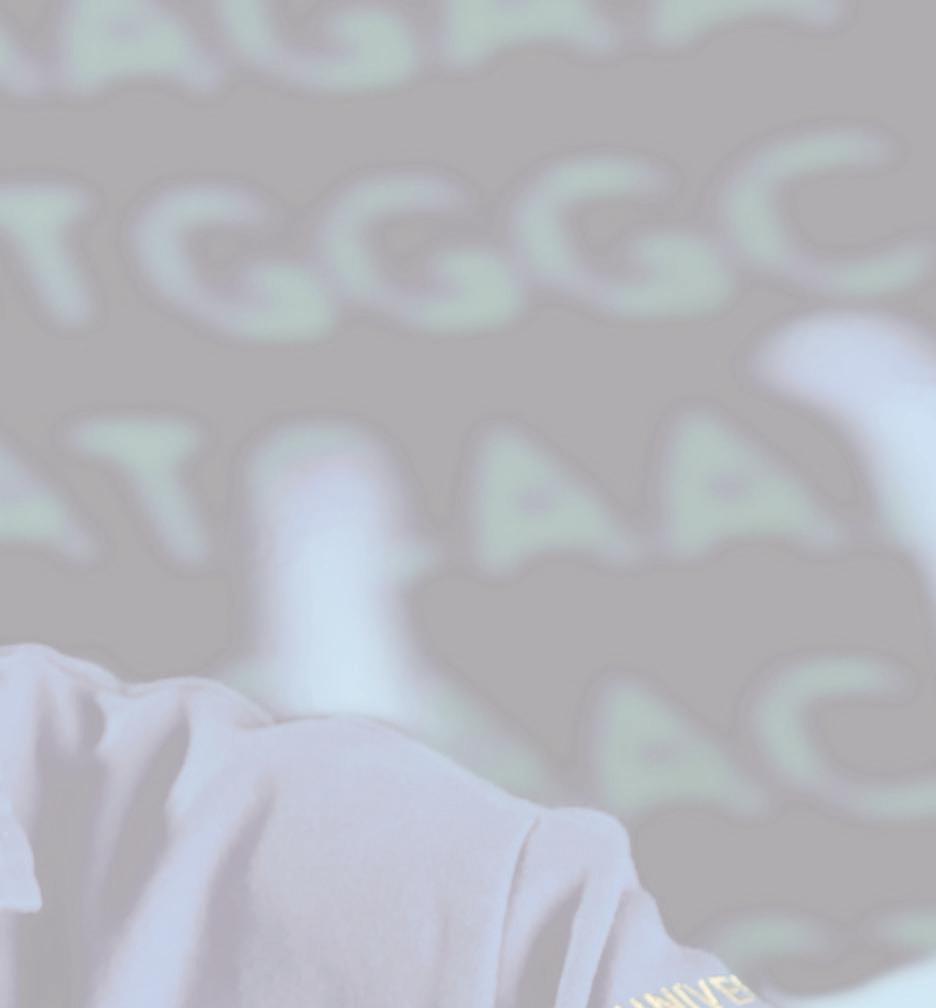
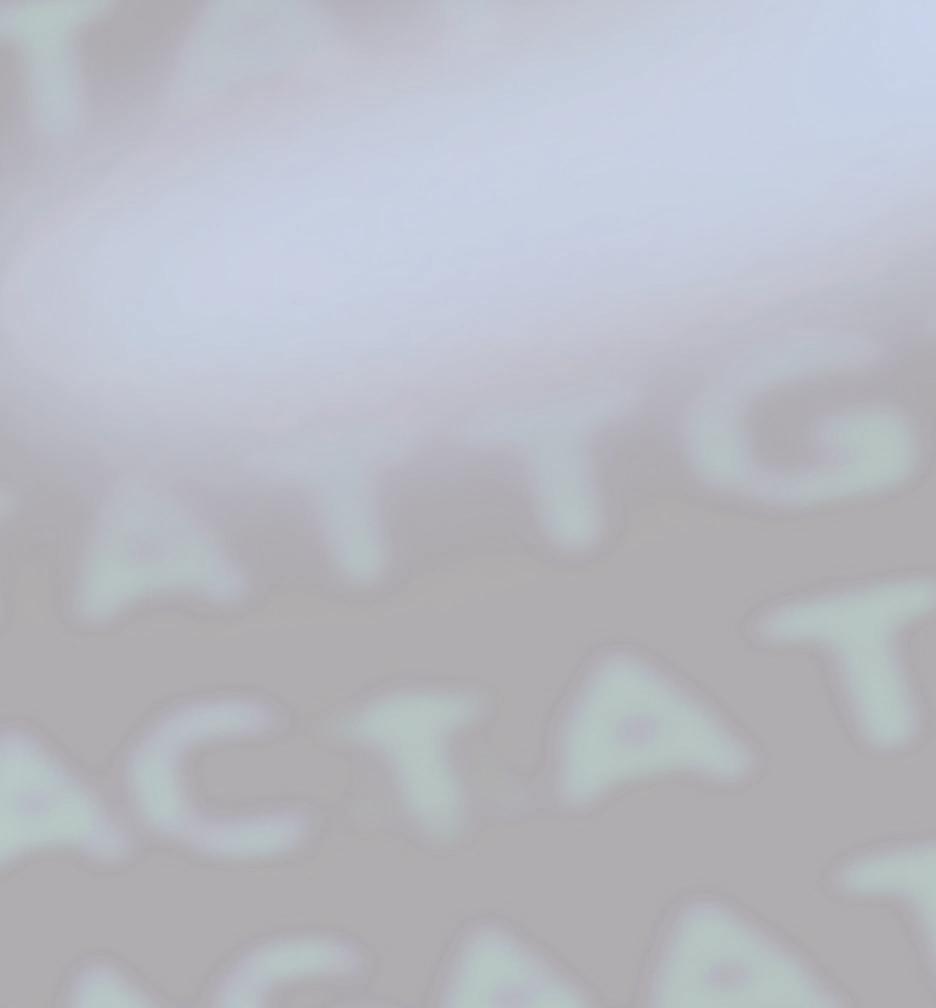
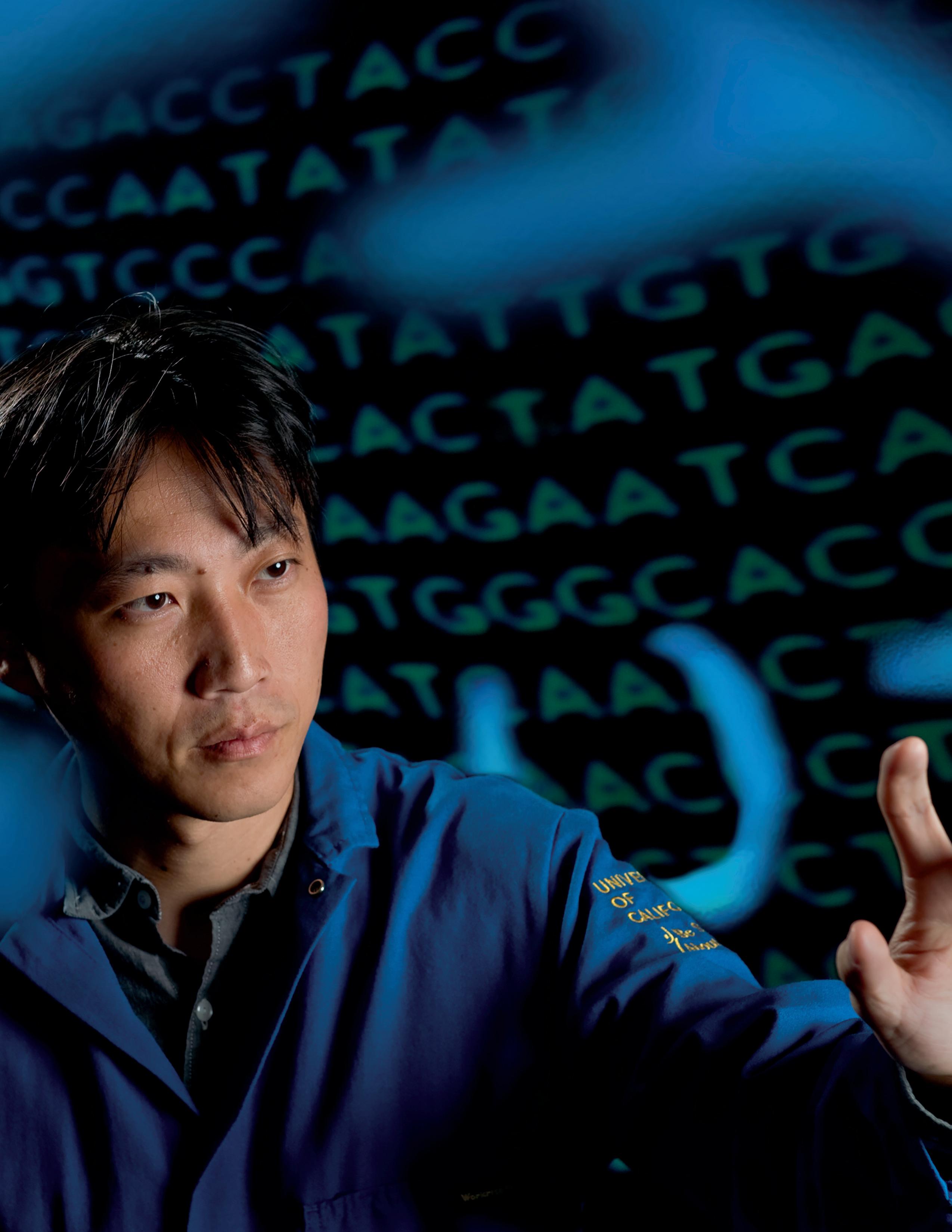
LIU RECEIVES NIH TRANSFORMATIONAL RESEARCH AWARD
Biomedical engineer Chang Liu is developing a system for making antibody generation a routine and widely accessible process. He won a Director’s Transformative Research Award from the National Institutes of Health under its High-Risk, High-Reward Research Program for the work and will receive $8.4 million over five years. Liu is one of only nine to receive this recognition.
“It is hard to overstate the importance of monoclonal antibodies in the life sciences,” said Liu, associate professor of biomedical engineering. “Antibodies are critical tools in biomedical research and diagnostics, and they are a growing class of therapeutics to combat cancer and pathogens up to and including the virus responsible for COVID-19.”
Liu said current methods for making custom antibodies are slow, costly, inaccessible to most researchers and often unsuccessful. His NIH proposal centers on simplifying the process through continuous and rapid evolution of high-quality antibodies requiring only the simple culturing of yeast cells.
He said his autonomously evolving yeast-displayed antibodies technology could have a transformative impact across the biomedical field by turning monoclonal antibody generation into a rapid, scalable and accessible process where any lab with standard molecular biology capabilities can generate custom antibodies on demand.
“We believe this can be achieved by combining our new technology for continuous protein evolution, a yeast antigenpresenting cell that we will engineer, and cutting-edge generative machine-learning algorithms for antibody library design,” he said.
Liu said that in addition to the continuous directed-evolution techniques that he has invented in his UCI laboratory, the project will rely on antibody design and artificial intelligence expertise provided by his collaborators on the project, Andrew Kruse and Debora Marks at Harvard Medical School.
The project could potentially result in “an explosion of crowdsourced antibody sequence data that will train our machinelearning algorithms to design better antibody libraries for our autonomous evolution system, starting a virtuous cycle,” he said.
In addition to contributing to cancer and anti-viral therapies, Liu said he and his fellow researchers will attempt to generate nanobodies against biogenic receptors that respond to acetylcholine, adrenaline, dopamine and other neurotransmitters. The goal of this work will be to develop a better understanding of psychopharmaceuticals in neurobiology and addiction.
“In the past, the Transformative Research Award has allowed some of the most ambitious and impactful ideas in biomedicine to blossom, and several previous winners are scientific heroes of mine,” said Liu. “We have big shoes to fill, but we are up to the challenge.”
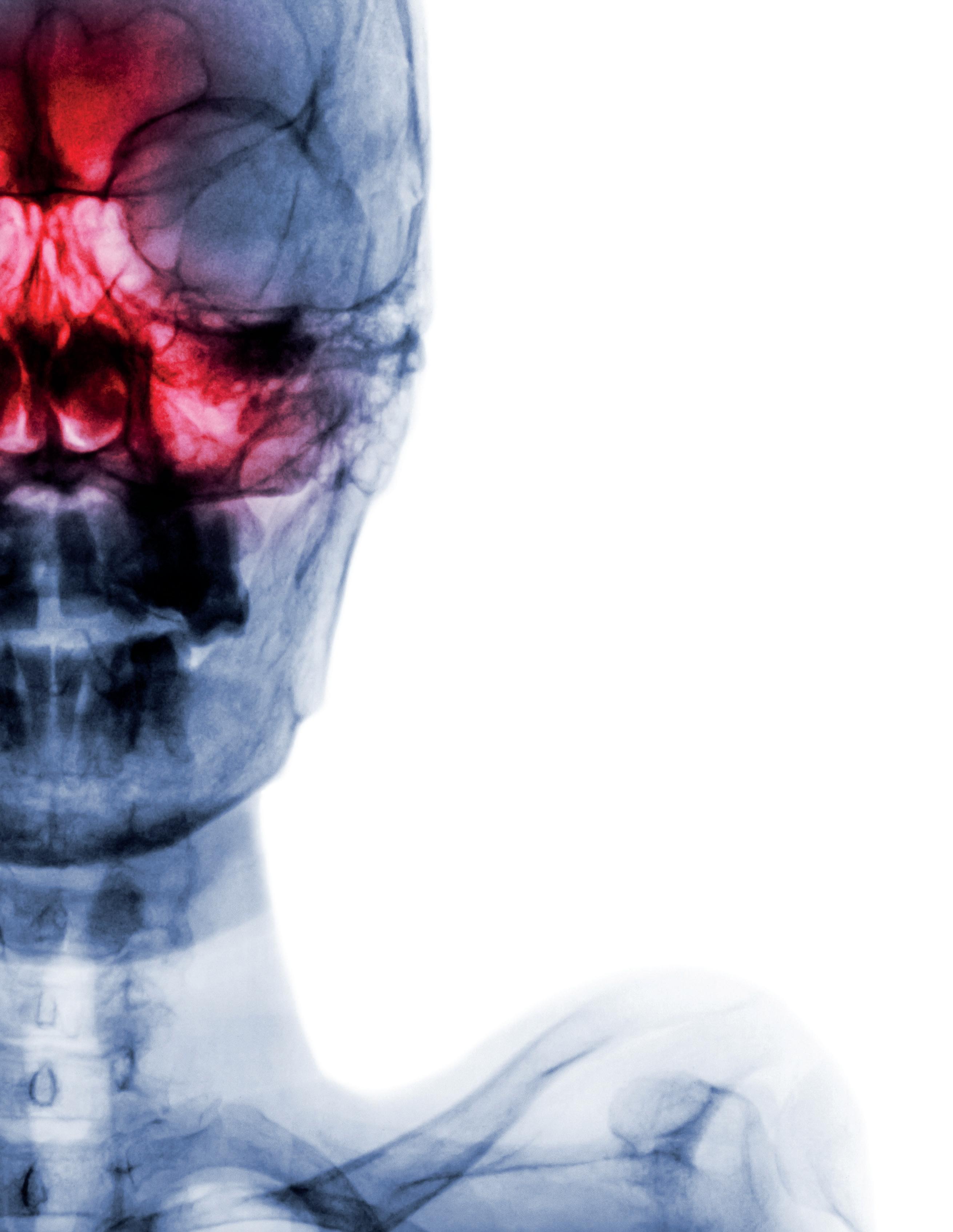
RESEARCHERS ADVANCE EFFORTS TO CHARACTERIZE MUCOSAL HEALTH
Approximately 50 million Americans suffer from painful sinus and allergic nasal upper respiratory ailments. Known medically as chronic rhinosinusitis and allergic rhinitis, the resulting headaches, stuffy/runny noses, itchy eyes and sneezing are responsible for more than $35 billion a year in healthcare costs and 3.5 million missed workdays. UCI biomedical researchers are building a technology that could help doctors treat these ailments. With a $2.3 million, four-year R01 award from the NIH’s National Institute of Biomedical Imaging and Bioengineering, Zhongping Chen and Dr. Brian Wong are creating an innovative in vivo imaging system called phase-resolved spectrally encoded endoscope (PR-SEE).
“Right now, the response to therapy is entirely based on patientreported outcomes,” said Wong, an otolaryngologist/facial surgeon who has a joint appointment in biomedical engineering.
The PR-SEE will employ two distinct imaging techniques to overcome current limitations on in vivo cilia imaging. (Cilia are tiny, hairlike structures on airway cell surfaces that sweep in a rhythmic pattern to transport mucus.) Optical coherence tomography (OCT) uses two scanning mirrors to provide information from deep within tissue. Spectrally encoded interferometry, a method that uses one mirror, provides faster imaging speed, but cannot achieve deep measurements like OCT. Together, the two techniques can give medical professionals quantitative information they have not had access to. “This has never been done in vivo before,” said Chen, professor of biomedical engineering.
The device will measure ciliary beat frequency (CBF) – the speed cilia sweep. Along with other factors, the CBF determines the efficiency of mucus transport, providing a strong indicator of upper airway health.
The device also will assess amplitude and propagation of the mucosal metachronal waves. “CBF is only one of the many factors that dictate the ability of cilia to transport mucus,” explained Chen. “It is also important to study the sweeping pattern (amplitude) and how well each cilium coordinates with each other (metachronal waves). These quantitative factors will provide a more comprehensive understanding of how airway cilia work, and will go a long way toward determining upper airway health.”
Rhinosinusitis currently accounts for more antibiotic prescriptions than any other diagnosis in ambulatory settings, and severe cases result in 600,000-plus sinonasal operations annually.
Chen added: “This imaging modality establishes an objective means to gauge sinus health and the response to treatment, which will aid us in better development of drugs, devices and other therapies.”
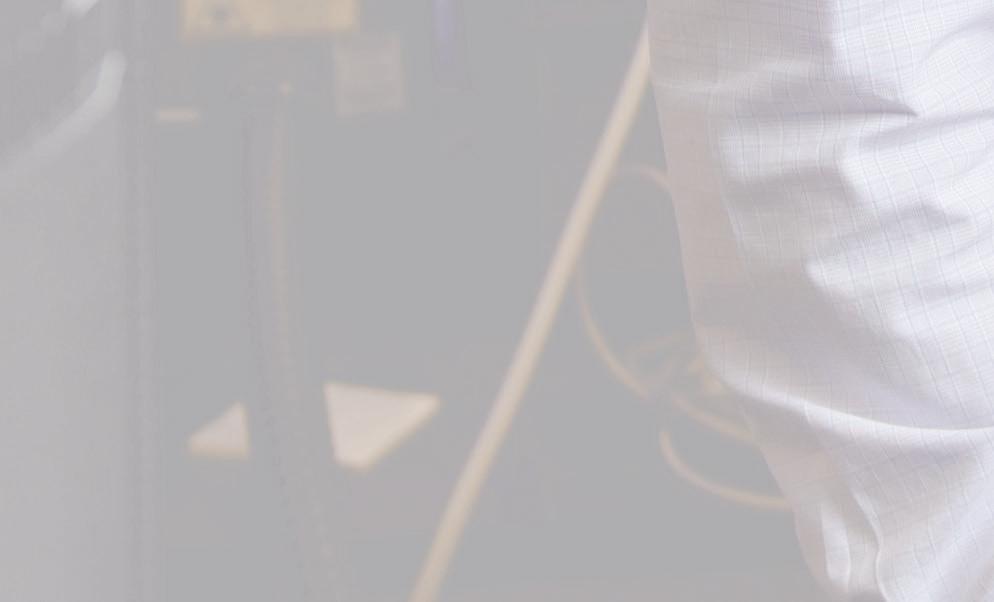
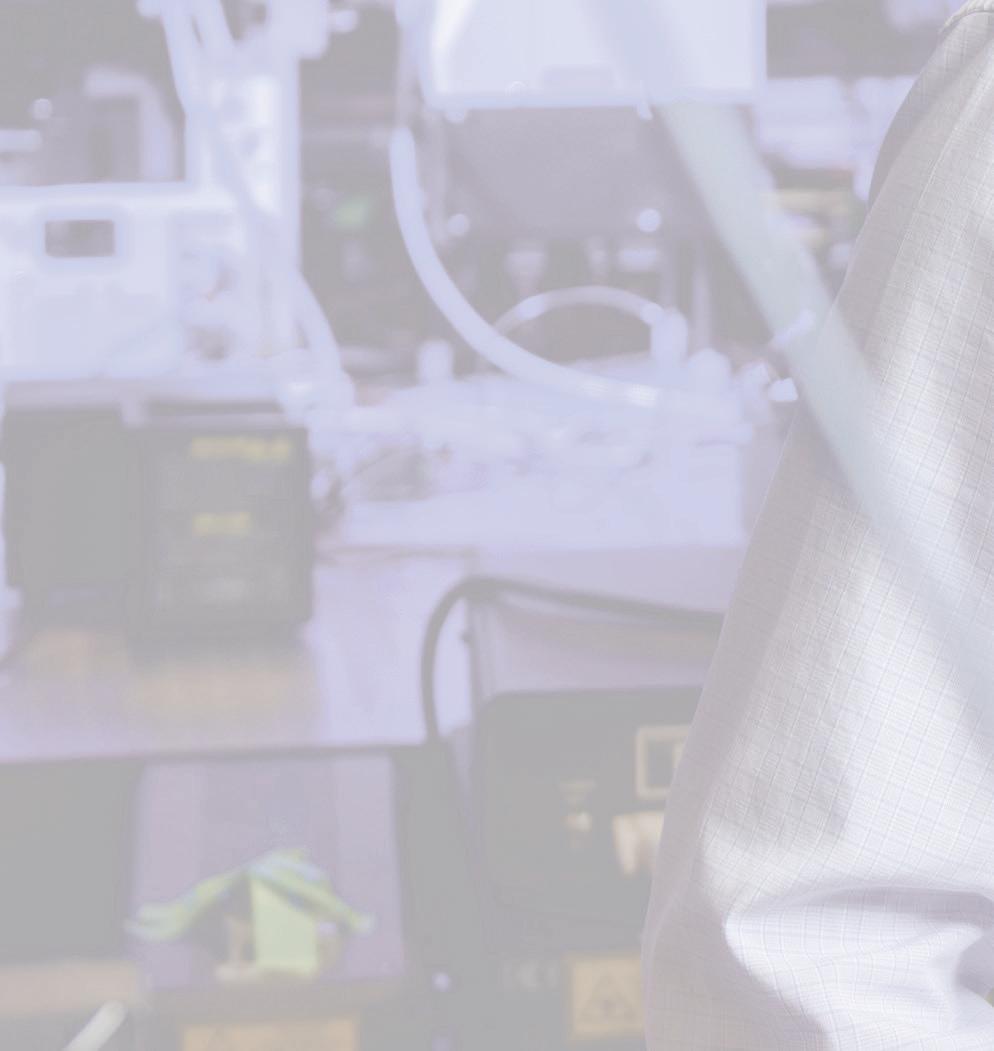
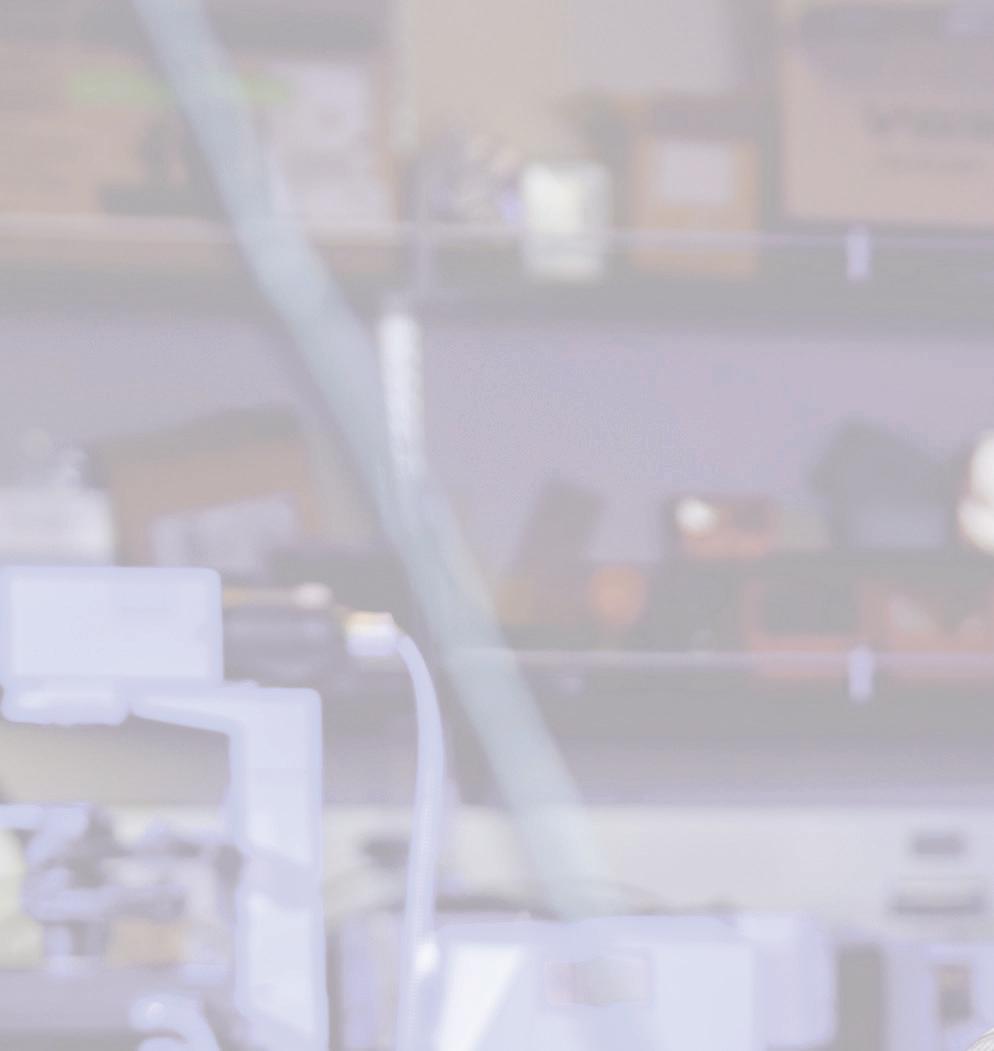

BOTVINICK TO DEVELOP FIRST-OF-ITS-KIND DIABETES MONITOR
Elliot Botvinick, professor of biomedical engineering, has been awarded a three-year, $3.5 million grant from The Leona M. and Harry B. Helmsley Charitable Trust to further the development of an innovative continuous-use monitor for those with Type 1 diabetes. The first-of-itskind device will simultaneously measure insulin, glucose, lactate, oxygen and the ketone body beta-hydroxybutyrate with a single probe inserted just beneath the skin.
Called iGLOBE (Insulin + Glucose + Lactate + Oxygen + BetaHydroxybutyratE) LifeStrip, the monitor utilizes light and chemistry to provide sensing capabilities for multiple analytes, which can be critical for controlling blood glucose and detecting possible dangerous events. The device will include continuous insulin monitoring and improve dosing efficacy by providing real-time feedback on the dynamics of insulin-pump therapy as well as real-time estimates of a patient’s sensitivity to the insulin.
It is also important to monitor blood glucose in those with Type 1 diabetes, known as insulin dependent diabetes. When blood glucose is elevated above normal values, called hyperglycemia, the body produces a chemical called beta-hydroxybutyrate. Elevated beta-hydroxybutyrate is associated with diabetic ketoacidosis, a dangerous condition, which can result in hospitalization or death. iGLOBE monitors this chemical to indicate dangerous levels and ensure automated insulin delivery functions properly.
Monitoring lactate, produced during exercise, is also important, as it can indicate changing metabolic states, which can lead to changes in blood glucose hours after exercise. This will improve glucose prediction and improve insulin dosing.
“Clinical evidence suggests that both beta-hydroxybutyrate and insulin sensing would improve outcomes and decrease the rates of hospitalization, severe morbidity and death associated with hypo- and hyperglycemia,” said Botvinick, who is also associate director of UCI’s Edwards Lifesciences Center for Advanced Cardiovascular Technology and professor of surgery at UCI Beckman Laser Institute (BLI).
The addition of beta-hydroxybutyrate and insulin monitoring capabilities has the potential to be life-altering. “When taken together, glucose, lactate, beta-hydroxybutyrate and insulin monitoring can transform the care of people with Type 1 diabetes,” Botvinick said. “iGLOBE can improve glucose control, compensate for glucose variations associated with exercise, inform of possible or current diabetic ketoacidosis and inform of failing or failed insulin delivery.”
Botvinick is collaborating with Gregory Weiss, UCI professor of chemistry, molecular biology and biochemistry; and David O’Neal, M.D., professor of endocrinology at Australia’s University of Melbourne. The team includes John Weidling, BLI associate project scientist, and biomedical engineering graduate students Toni Wilkinson and Dat Nguyen.
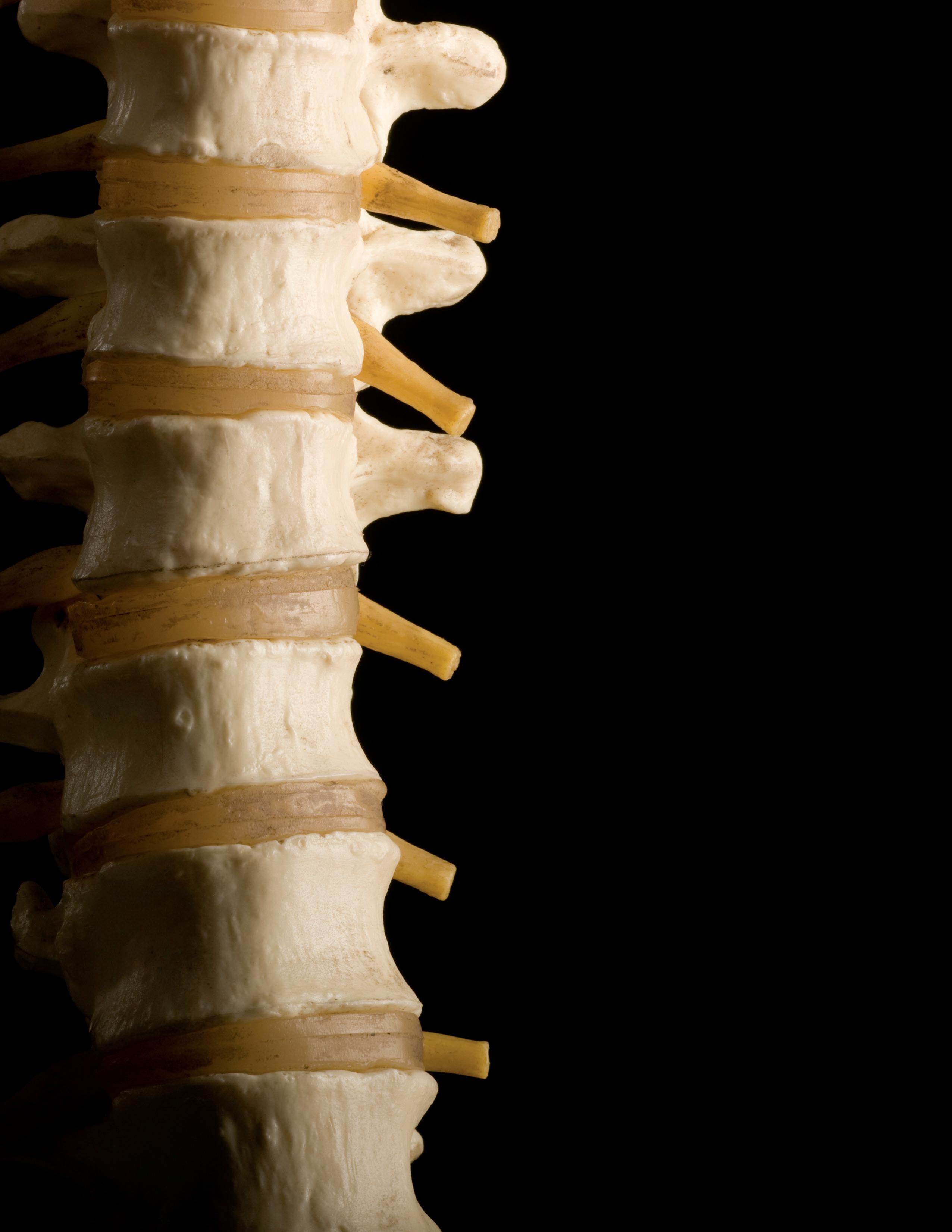
TEAM FOCUSED ON BIOLOGIC IMPLANT FOR SPINAL FACET JOINTS
Kyriacos Athanasiou, Henry Samueli Chair in Engineering and Distinguished Professor of biomedical engineering, is creating a biologic implant for the spinal facet joint. With a $2 million NIH grant, the Samueli School research team, led by Athanasiou, will be the first to focus on the treatment of facet joint degeneration, a highly prevalent contributor to back pain.
“The goal of the project is to use selfassembled cartilage, which is a technique for cartilage tissue engineering that my group has been developing for over a decade, to generate an implant that can restore structure and function to degenerated facet joints,” Athanasiou said.
Current treatment options for the condition are limited. “It has been found that degeneration in the facet joints is present in 70 percent of the population by the age of 30,” said team member Rachel Nordberg, a postdoctoral scholar in biomedical engineering. “As we age, this degeneration continues to progress, afflicting essentially the entire population by the age of 60.”
Co-investigator Jerry Hu, a program manager in the Department of Biomedical Engineering, added: “Unfortunately, management of facet pain is short-lived. On average, treatments such as neurotomy last for only nine months, which is why we’re seeking to develop novel living tissue implants for the facet.”
Other collaborators on the NIH-funded project include Drs. Michael Yaszemski and Benjamin Elder, spine surgeons at the Mayo Clinic.
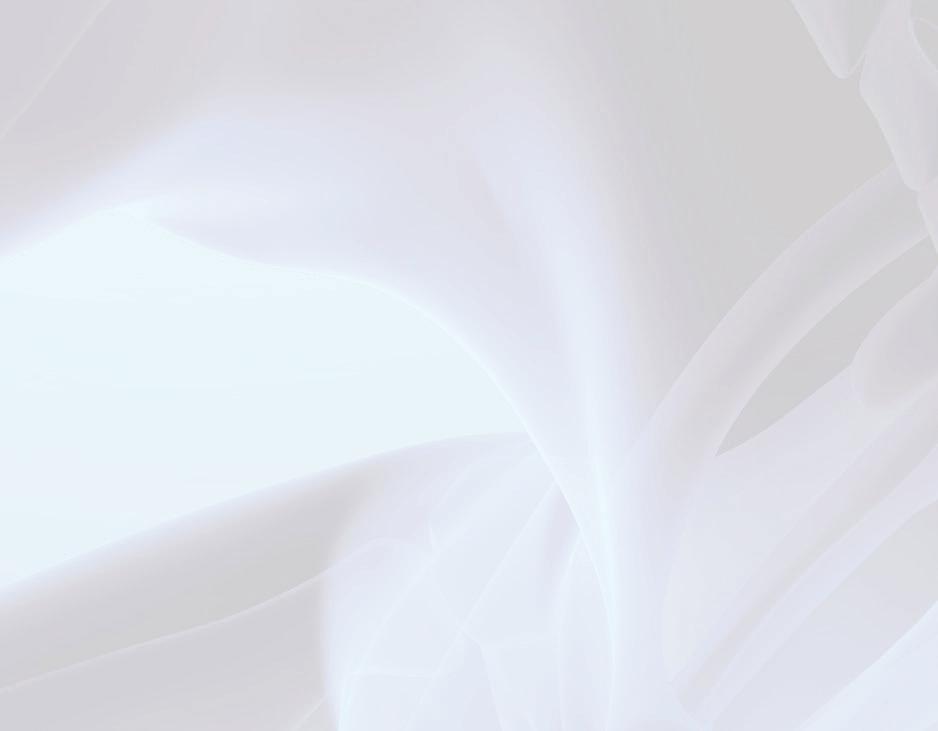
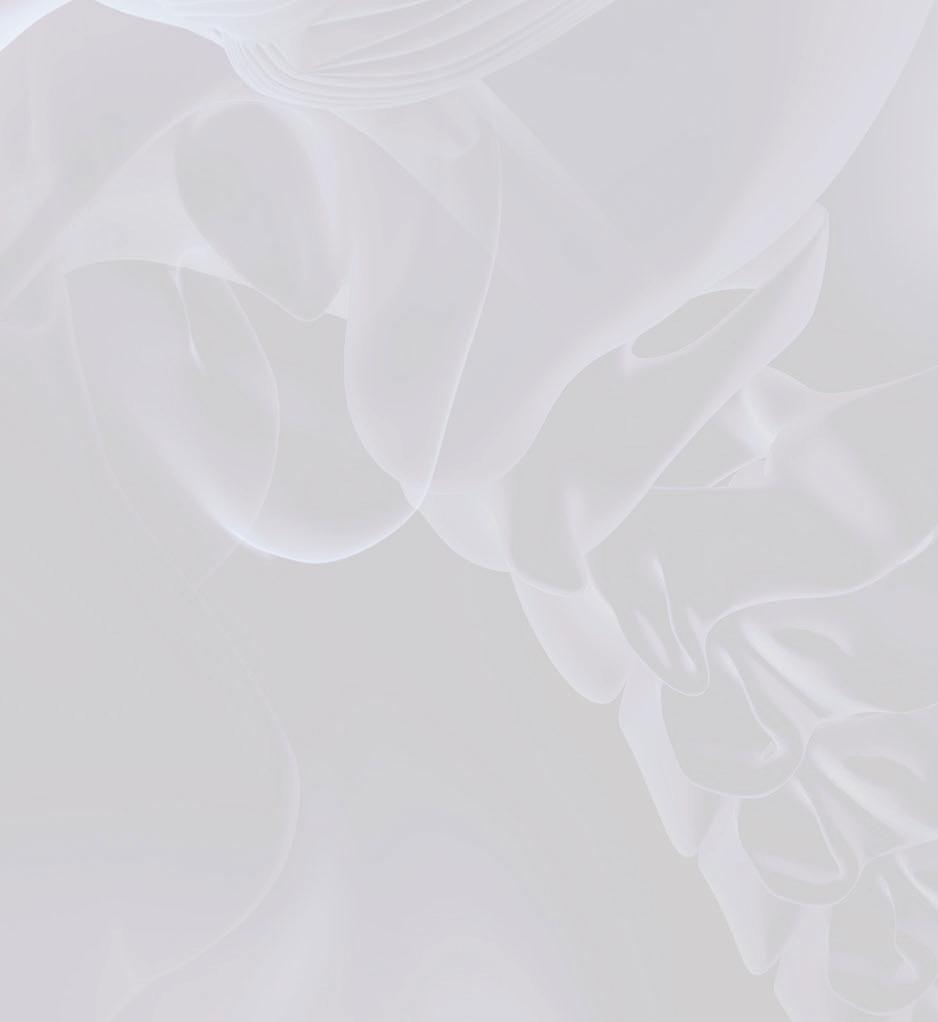
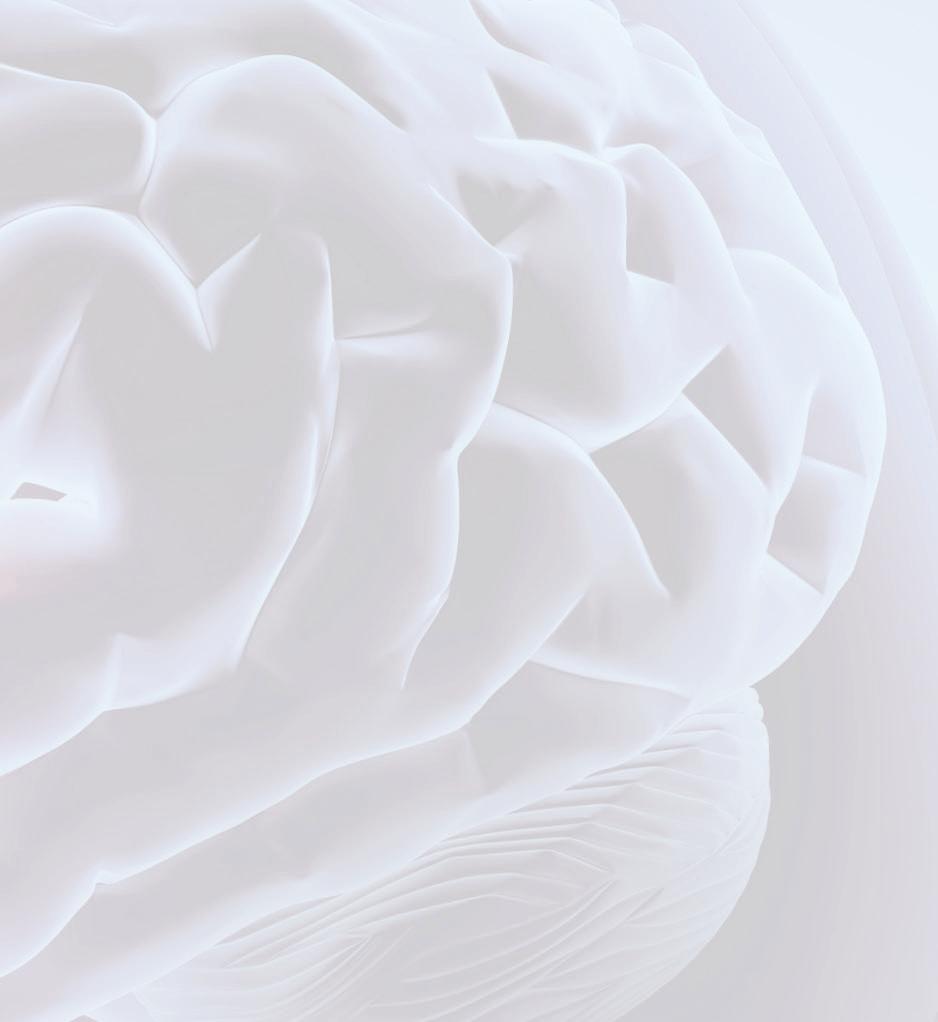
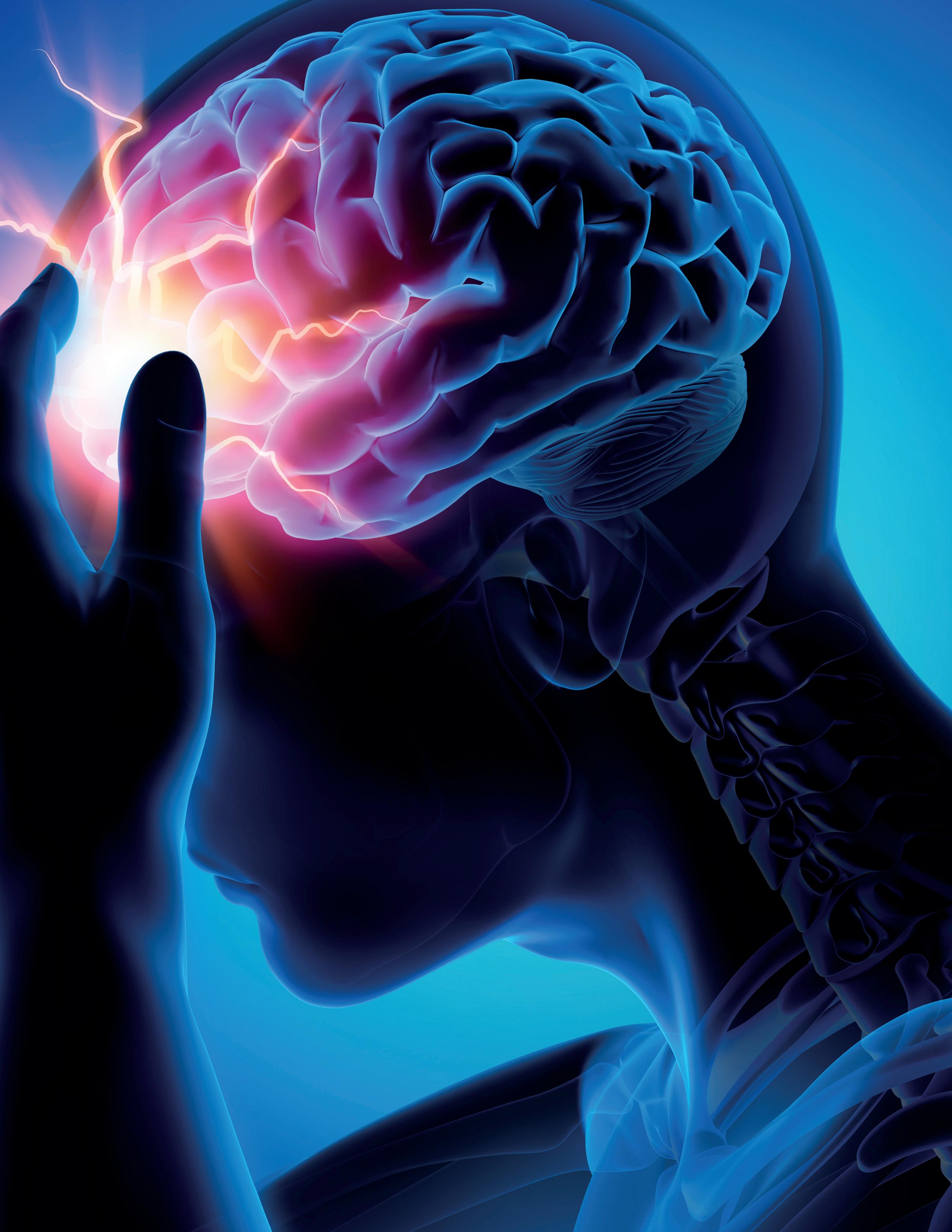
COLLABORATIVE EFFORT TO IMPROVE EPILEPSY SURGERY
In the United States, 3.4 million people live with active epilepsy, a disorder in which nerve cell activity in the brain is disturbed, causing seizures. The neurological disorder is usually treated with medication. However, for people with severe epilepsy, surgery may be the best option.
Beth Lopour, associate professor of biomedical engineering, is collaborating with pediatric epilepsy specialists at Children’s Hospital of Orange County (CHOC) on research that she hopes will improve the quality of life for children with the most severe cases of epilepsy.
She won a $1.8 million five-year R01 grant from the NIH National Institute of Neurological Disorders and Stroke to develop and validate computerized tools to more accurately and objectively identify regions in the brain where epileptic seizures originate.
Currently, to determine where the seizures are originating, clinicians implant electrodes directly onto a patient’s brain to continuously record electrical activity. This invasive recording may last days to weeks, until enough data is captured to proceed with surgery. Surgeons and epilepsy specialists then use this information, combined with brain imaging and other test results, to guide removal of the seizure-generating brain tissue.
The problem is current epilepsy surgery does not always work to curtail seizures. The majority of patients remain on anti-seizure medications after surgery, and roughly half of patients continue to have seizures.
“The long-term goal of this grant is to improve the outcomes of patients undergoing epilepsy surgery by developing more accurate methods to localize seizure-generating tissue,” said Lopour.
Specifically, Lopour is developing recording techniques, computational algorithms and data analysis methods to better identify and measure high-frequency oscillations, the short bursts of high-frequency electrical activity that occur in epileptic patients’ brains. Recent research has suggested that HFOs are a good biomarker of where seizures originate, and surgically removing HFO-generating brain tissue increases the likelihood of seizure freedom.
Lopour explains that seizures are unpredictable and scary, which not only affects children but also their caregivers and family. She is grateful for the support and participation from the patients and their families at CHOC. “Participating in clinical research is a brave and selfless act, and we are continuously humbled by the trust and support these families afford us,” said Lopour. “It is only because we have such great partnerships between researchers, clinicians and families that we can pursue such impactful work, and we sincerely hope our endeavors lead to improvements in the care and outcomes of patients with epilepsy.”
Lopour is working with CHOC Drs. Daniel Shrey, pediatric neurologist and epilepsy specialist, and Joffre Olaya, pediatric neurosurgeon.
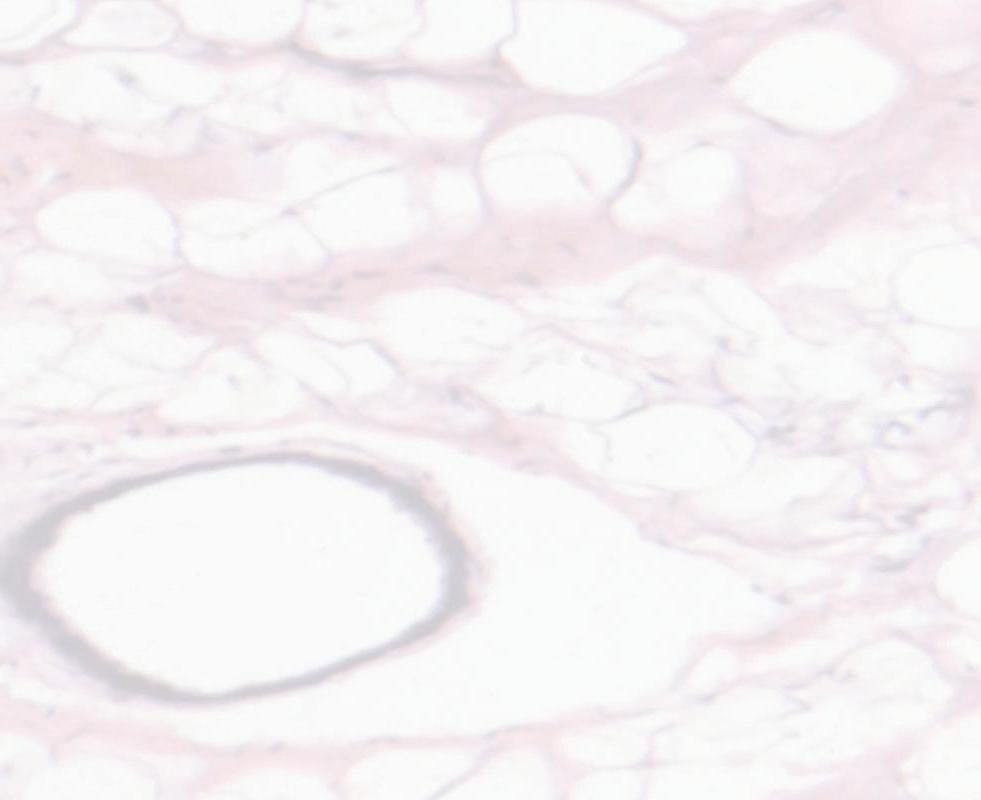
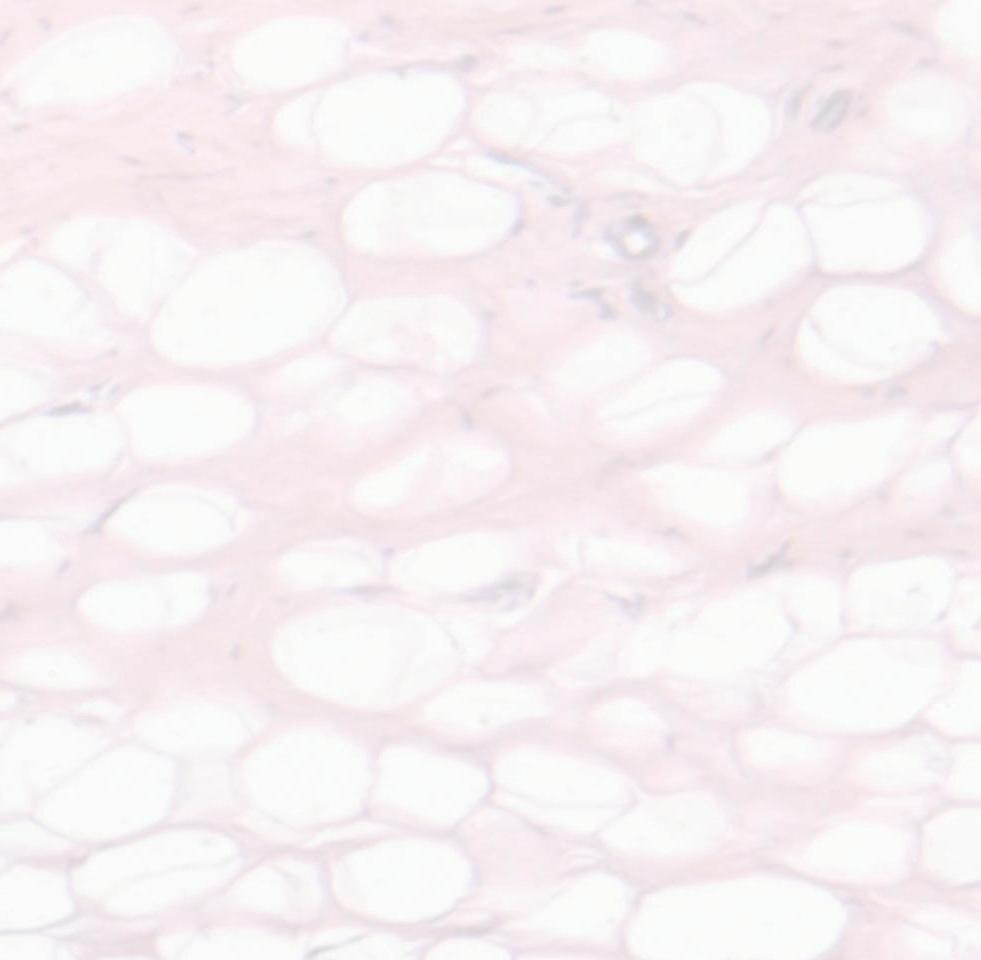
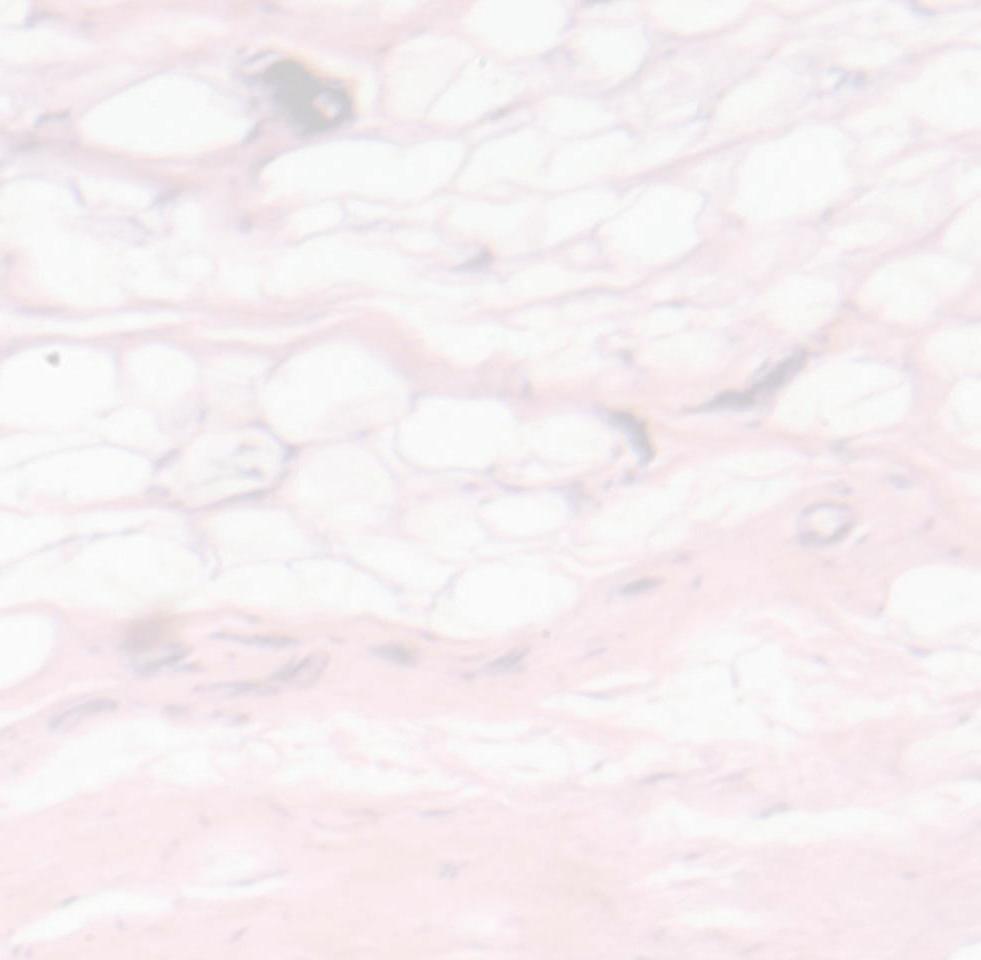
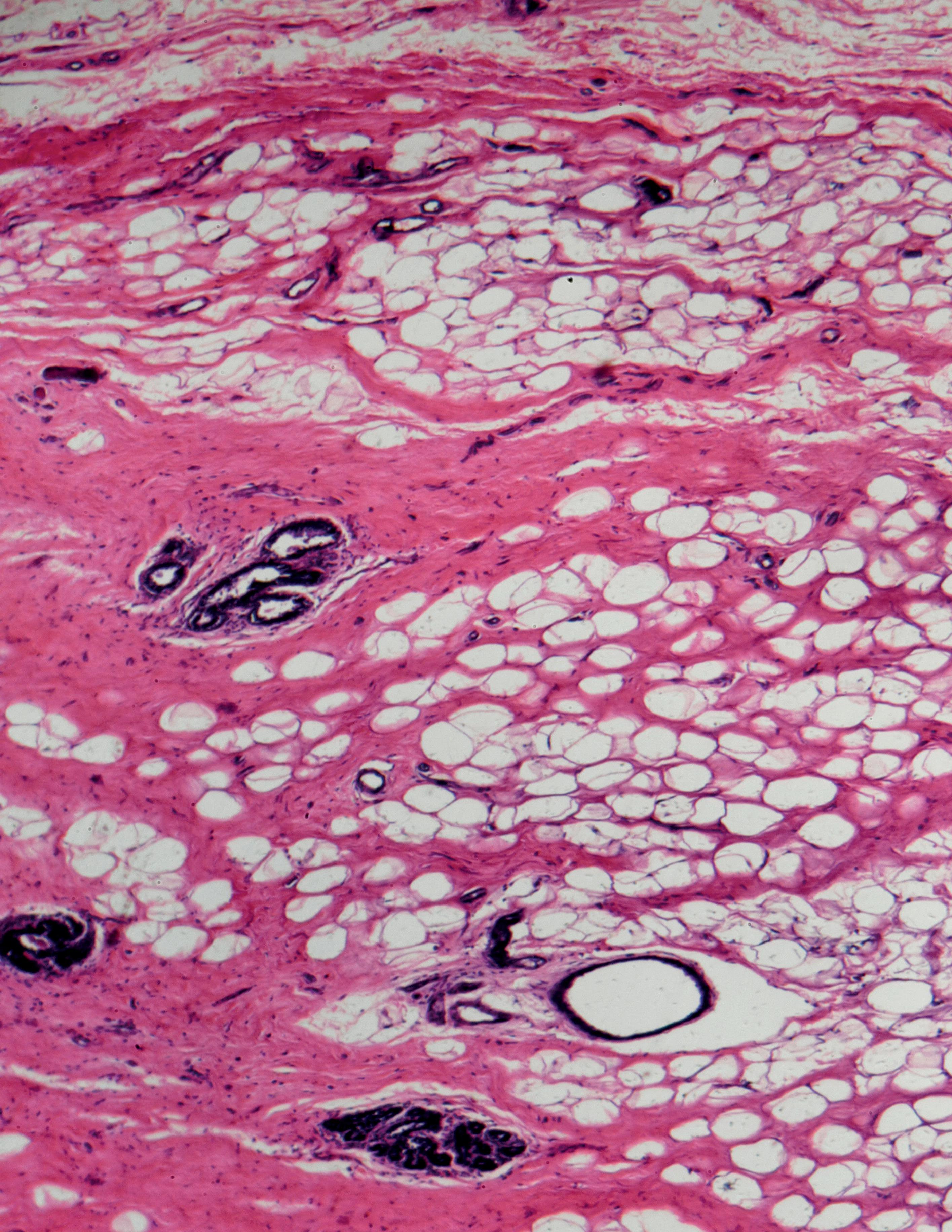
NEW SINGLE CELL ANALYSIS TECHNOLOGY HOLDS PROMISE TO ADVANCE DISEASE DIAGNOSIS
UCI biomedical engineering researchers have been awarded $1.1 million from the NIH National Cancer Institute’s Innovative Molecular Analysis Technologies program to further their work on an integrated microfluidic platform that could help dramatically change the way tumor tissue is clinically evaluated. With three years of funding support, the team, led by Jered Haun, associate professor, will be able to test the technology on human tissue samples. The results could help scientists make progress in disease diagnosis and drug development.
Solid tumors are complex mixtures of different cell types, and these differences are key factors driving disease progression, metastasis and drug resistance. “Assessing cellular heterogeneity and identifying key driver cells are critical for understanding tumor biology, and for creating the most powerful clinical diagnostics,” said Haun. “Targeted therapies must be directed toward the most important cell types if effective cures are to be achieved. With this technology applied in clinical settings, we hope to help usher in an era of precision molecular medicine.”
Currently, single cell analysis studies are hindered, as tissues must first be dissociated into single cell suspensions using methods that are often inefficient, labor-intensive and highly variable. Importantly, certain cell types can be released more easily than others, which will bias the single cell analysis assay and lead to incorrect conclusions.
The new platform will combine four separate microfluidic device technologies that Haun has pioneered. The devices were designed to work sequentially, starting from tissue specimen digestion, through dissociation and filtration to finally extracting single cells. Any remaining cell clusters would be recirculated back into the front end of the device to maximize cell recovery. Single cells will be continuously extracted from the system as soon as they are ready, within minutes after dissociation, to prevent overtreatment and maintain viability.
“This multifaceted approach will enable us to tailor flow properties and shear forces to the appropriate magnitude and size scale, resulting in gradual and ultimately complete breakdown of tissue in a fast, efficient and gentle manner,” said Haun.
The researchers will test each device separately using human breast, pancreatic and prostate tumor tissue specimens. They will then integrate all the devices into a versatile system that will operate one, multiple or all devices, as well as establish continuous processing. Finally, they will evaluate suspensions using single-cell RNA sequencing, which assesses each cell’s gene expression profile, to determine whether cell subtypes are biased by any device component and/or released at different time points during the process.
IMMUNE SYSTEM RESEARCH BENEFITS WOUND HEALING
The key to unlocking the mystery of how the immune system helps heal wounds appears to be in understanding the role of a protein called Piezo1, according to research by Wendy Liu. The professor of biomedical engineering published research on Piezo1 in Nature Communications.
Macrophages, a type of white blood cell in the immune system, perform different functions during immune responses to pathogens and injuries. Liu’s research found that macrophages lacking Piezo1, a mechanically activated cation channel protein, showed reduced inflammation and enhanced wound healing.
“In our study, we examined how cells of the immune system – specifically macrophages – respond to the stiffness of a material,” Liu explained. “Stiffness is important in many biological contexts. For example, when medical devices are implanted into the body, the materials they are made out of are usually much stiffer than the tissue around it, and these implants can cause inflammation and scarring that is mediated by macrophages. In addition, tumors or diseased cardiovascular tissues are often stiffer than healthy tissue, and macrophage activity is also involved in these diseases.”
The study revealed that Piezo1, which allows ions like calcium to pass through cell membranes, is a mechanosensor (able to sense mechanical stimuli) of stiffness in macrophages, and its activity modulates the behavior of these cells. In stiff environments, the ion channel becomes activated, causing more calcium in the cells and higher levels of inflammation. Macrophages cultured on soft substrates have reduced inflammatory activation when compared to cells adhered to glass or other stiff substrates.
“We found that macrophages that lack the Piezo1 channel could not sense different stiffness environments,” Liu said. “Furthermore, stiffer implants usually cause more scarring compared to soft implants. However, when Piezo1 was not present, scarring around the stiff implant was much less, and more like the response to a soft implant.
“By identifying a molecule that is required for sensing different stiffness environments, we can start thinking about therapies that target Piezo1 and control inflammation.”
Liu’s research was a collaboration with Medha Pathak and Michael Cahalan, both from the UCI School of Medicine.
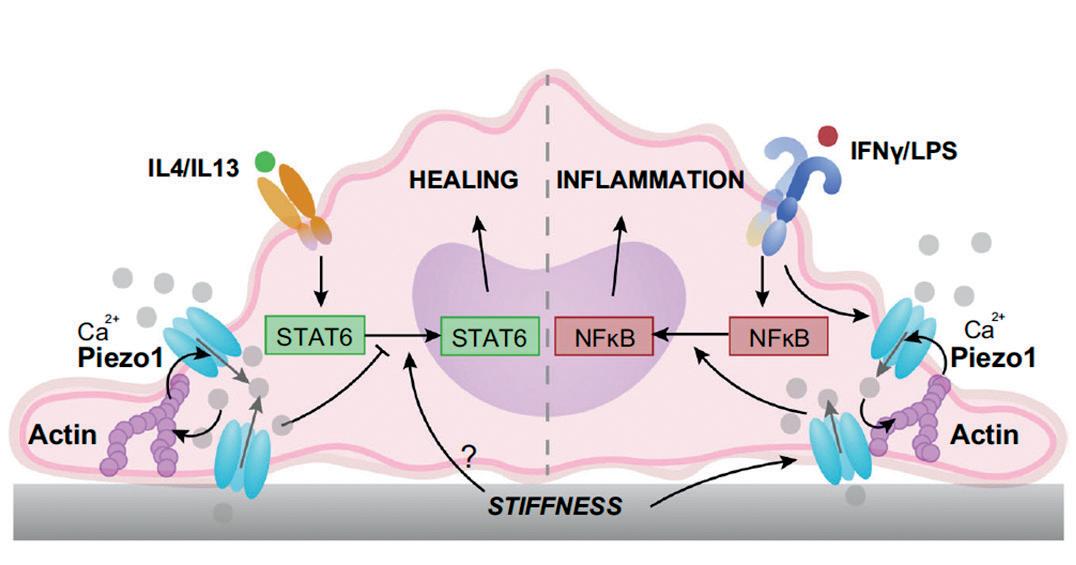
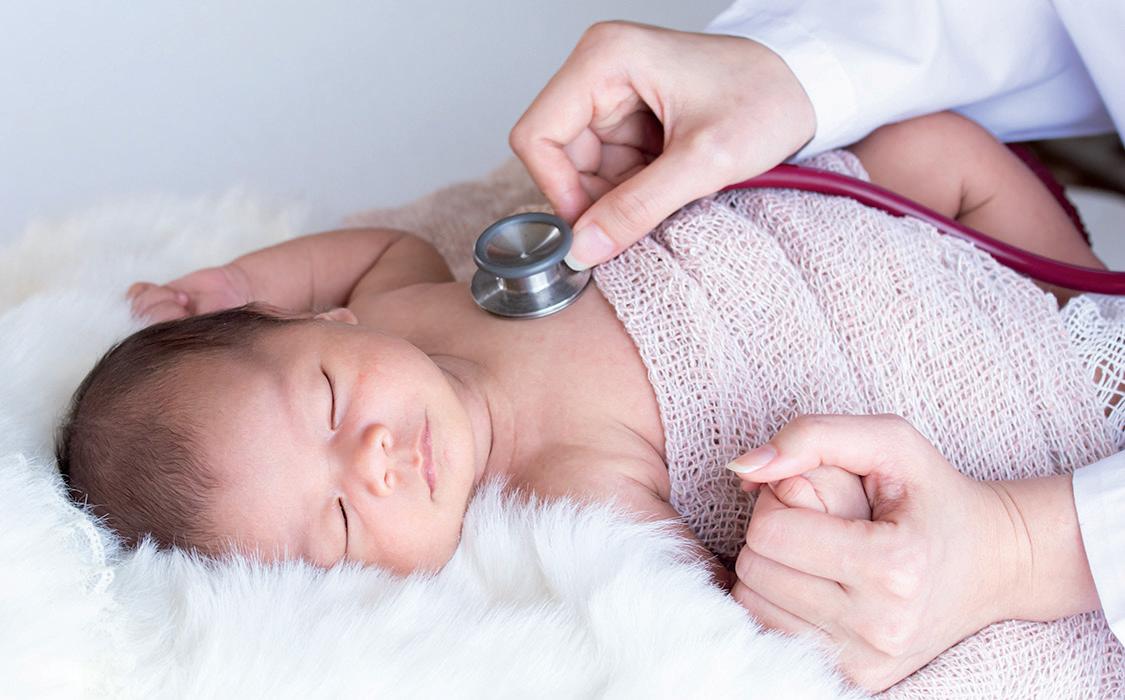
COLLABORATIVE GRANT FURTHERS EARLY HEART DEVELOPMENT PROJECT
Biomedical engineers from UCI and Oregon Health and Science University are collaborating on research to better understand how blood flow and genetic processes influence early heart development. Their project has been awarded two, three-year collaborative grants from the National Science Foundation Division of Chemical, Bioengineering, Environmental, and Transport Systems totaling $650,000.
Congenital heart defects are present at birth and can affect the structure of a baby’s heart and the way it works. About 1 in every 4 babies born with a heart defect has a critical congenital heart defect and will need surgery or other procedures in the first year of life, according to the Centers for Disease Control and Prevention. Why most defects come about and how they eventually lead to heart failure remains unknown.
UCI’s Dr. Arash Kheradvar, professor of biomedical engineering, will join OHSU’s Sandra Rugonyi, professor of biomedical engineering, to conduct the research. Kheradvar explains that although scientists speculate both blood flow and genes contribute to heart development, it is not yet known how they interact with each other and the synergistic roles they play in heart malformations.
“Using advanced imaging methods and computational simulations, our teams plan to unravel how altered blood flow affects programmed genetic processes, and conversely how altering genetic processes changes blood flow, leading to hearts with defects,” said Kheradvar.
Using two avian models of heart development, the research has three main objectives: to determine blood flow and flow-induced stresses during normal and aberrant cardiac formation through advanced engineering methods; identify and quantify cellular responses to normal and abnormal heart development by generating spatiotemporal maps of cardiac adaptation; and ascertain the genetic and epigenetic adaptations in cardiac tissues using sequencing technologies.
More broadly, research results will provide fundamental knowledge on embryonic heart development, help with strategies to improve diagnosis of heart defects and preventing heart malformations, and eventually guide early fetal interventions to repair cardiac defects and promote healthy heart function.
The award also supports outreach activities involving rising high school students in California and Oregon. UCI’s ASPIRE (Access Summer Program to Inspire, Recruit and Enrich) will be implemented at OHSU.
RESEARCHERS USE MICROFLUIDICS TO ASSIST IN AGRICULTURAL MEASUREMENTS
Plant researchers search for ways to adapt crops to be more nutritious, resource-efficient and resilient in a variety of climates. Genomics, and more specifically genotyping, which measures genetic identity, play a growing role in this ongoing endeavor to breed new crop varieties. Agricultural companies often perform hundreds of thousands of these genetic measurements each day.
The Samueli School’s Center for Advanced Design and Manufacturing of Integrated Microfluidics (CADMIM), a National Science Foundation-backed collaboration between university researchers and industry partners, has received a new NSF grant to continue their work on a microfluidic device that can sustainably monitor and improve agricultural crop breeding.
The $250,000 Partnerships for Innovation grant from the NSF Division of Industrial Innovation and Partnerships leverages technology created over the last few years by CADMIM researchers. Biomedical engineering Associate Professor Elliot Hui leads the team creating a device that automates the measurement of genetic markers across many plants simultaneously, using microfluidics. The process uses fewer supplies, runs reactions faster and enables the completion of four times the number of tests in a given time frame compared to current technology, all at a fraction of the cost.
The project, a partnership with agricultural plant seed producer KWS SAAT SE & Co., has already achieved proof-of-concept, and the new funds will advance device development and production. The team will use the new grant to transition to a platform that will be faster to manufacture and more conducive to performing the biochemical reactions. They will also work to scale up the device and create a fully functional prototype.
The Partnerships for Innovation grants are specifically targeted to bridge the gap between academic research and commercialization. Through the NSF I-Corps program, the research team will interview 100 potential customers to identify customer needs. A large component of the grant also focuses on leadership and innovation. Hinesh Patel, a graduate student in Hui’s lab, will receive entrepreneurship training to help the team successfully produce and market the technology.
While the original research was geared to the plant agriculture industry, the technology can extend to other applications, including medical research, healthcare and consumer markets like genealogy.
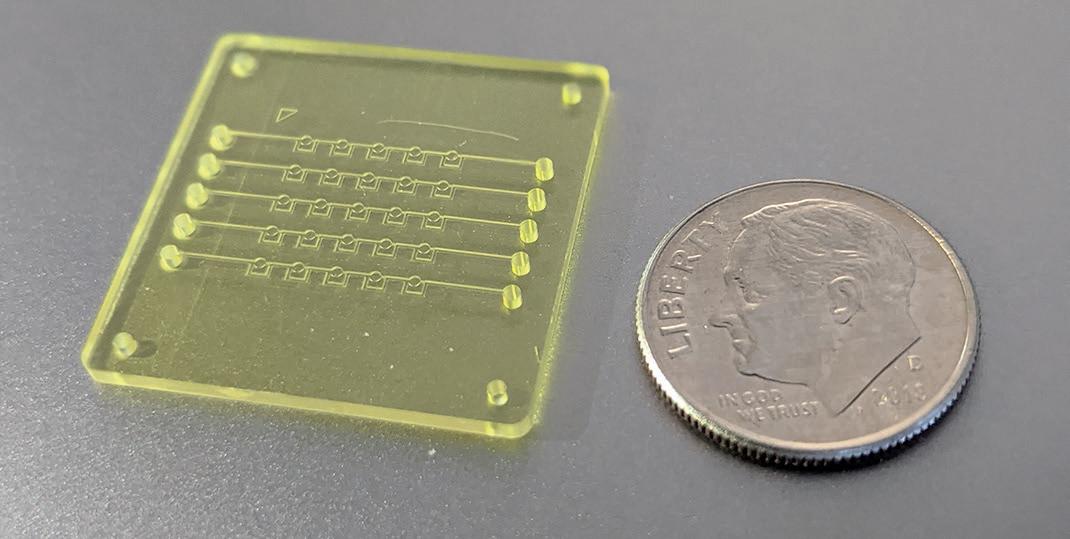
BIOCHIP INNOVATION COMBINES AI AND NANOPARTICLE PRINTING FOR CANCER CELL ANALYSIS
A new lab-on-a-chip can help study tumor heterogeneity to reduce resistance to cancer therapies. In Advanced Biosystems, UCI researchers describe combining artificial intelligence, microfluidics and nanoparticle inkjet printing in a device that enables examining and differentiating cancers and healthy tissues at the single-cell level. “Cancer cell and tumor heterogeneity can lead to increased therapeutic resistance and inconsistent outcomes,” said lead author Kushal Joshi, former biomedical engineering graduate student. The team’s biochip addresses this problem through precise characterization of a variety of cancer cells from a sample.
“Single-cell analysis is essential to identify and classify cancer types and study cellular heterogeneity. It’s necessary to understand tumor initiation, progression and metastasis in order to design better cancer treatment drugs,” said co-author Rahim Esfandyarpour, assistant professor of electrical engineering and computer science with a joint appointment in biomedical engineering.
His group combines machine learning techniques with accessible inkjet printing and microfluidics technology to develop low-cost, miniaturized biochips that are simple to prototype and capable of classifying various cell types.
In the apparatus, samples travel through microfluidic channels with carefully placed electrodes that monitor differences in the electrical properties of diseased versus healthy cells in a single pass. The researchers’ innovation was to devise a way to prototype key parts of the biochip in about 20 minutes with an inkjet printer, allowing for easy manufacturing in diverse settings. Most of the materials involved are reusable or inexpensive.
The invention also incorporates machine learning to manage large amounts of data the system produces. This improves the accuracy of analysis and reduces dependency on skilled analysts.
Esfandyarpour said, “Our work has potential applications in single-cell studies, in tumor heterogeneity studies and, perhaps, in point-of-care cancer diagnostics – especially in developing nations where cost, constrained infrastructure and limited access to medical technologies are of the utmost importance.”