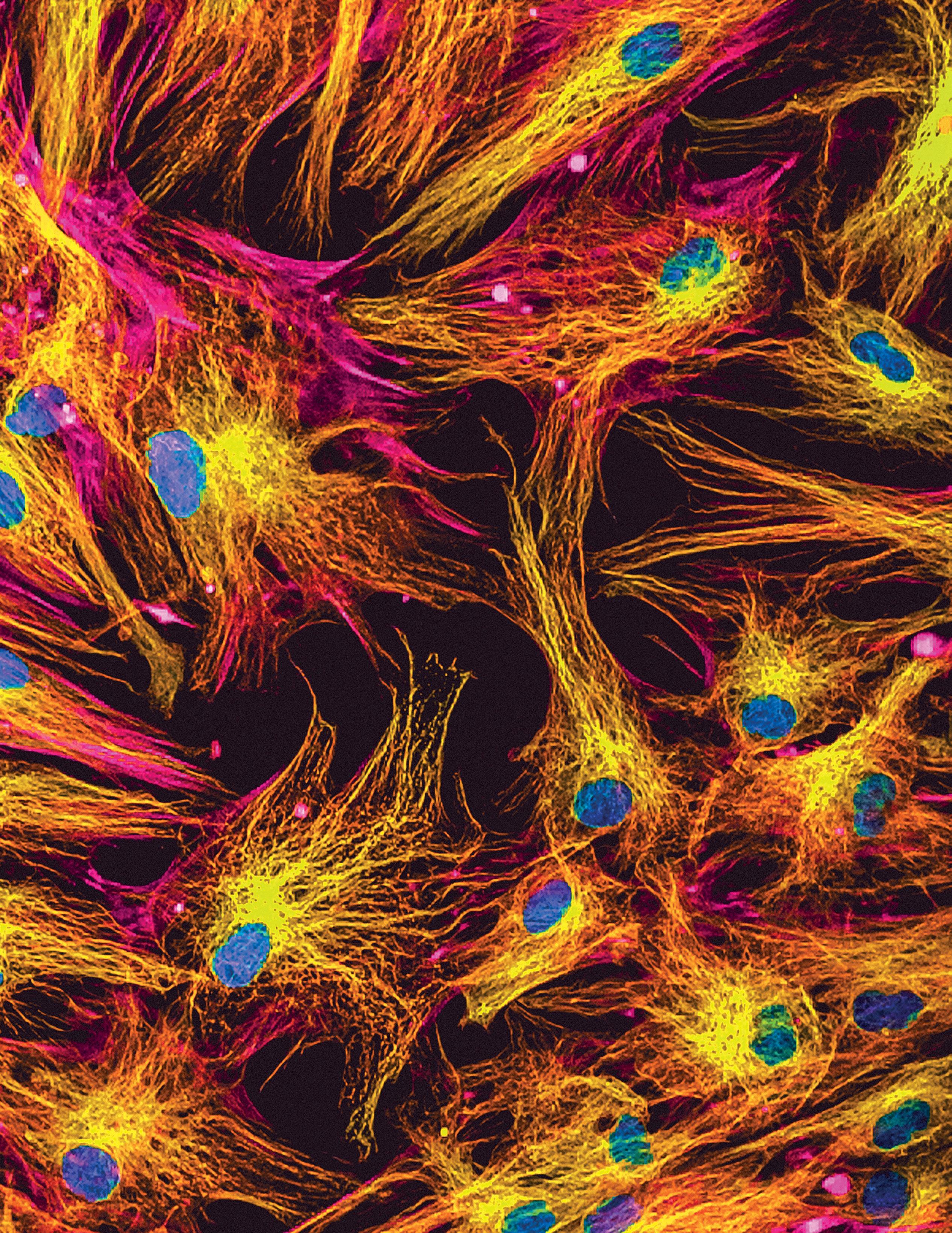
16 minute read
Research & Funding
RESEARCHERS DEVELOP NEW BIOPSY TECHNOLOGY FOR ANALYZING MULTIPLE TUMOR TISSUE BIOMARKERS
A team led by UCI biomedical researchers Weian Zhao and Enrico Gratton has developed a new biopsy technology that can profile multiple tumor microenvironment biomarkers simultaneously, revealing cellular spatial organization and interactions that will help advance personalized disease diagnosis and treatment. Current single-biomarker biopsies lack the ability to analyze many different markers and often fail to predict patient outcomes.
Called the Multi Omic Single-scan Assay with Integrated Combinatorial Analysis, the fluorescence imaging-based technology can spatially profile a large number of mRNA and protein markers in cells and tissues, including clinical tumor tissues. A study published in Nature Communications shows that MOSAICA enables direct, highly multiplexed biomarker profiling in a 3D spatial context using a single round of staining and imaging instead of the repeated processing steps typically needed in conventional methods.
Clinicians and scientists will now have a holistic view of the different immune and cancer cell types in tumor tissues, providing greater insight for determining patient prognosis and treatment.
“Spatial biology is a new science frontier and mapping out each cell and its function in the body at both the molecular and tissue level is fundamental to understanding disease and developing precision diagnostics and therapeutics,” said Zhao. “Many cancer immunotherapeutics, including immune checkpoint inhibitors, don’t work and scientists realized that was because of the spatial organization of all the tumor tissue cell types, which dictates drug efficacy. The MOSAICA can characterize the spatial cellular compositions and interactions in the tumor immune microenvironment in biopsies to inform personalized diagnosis and treatment.”
A startup company called Arvetas Biosciences, Inc. was cofounded by Zhao and Alan K. Hauser, Ph.D., to further develop and commercialize the technology for widespread use, ranging from oncology to neurological disorders.
NEW MICROFLUIDIC PLATFORM DEVELOPED FOR INTRACELLULAR DELIVERY
Cell therapy has become a powerful tool to treat patients suffering from cancer and other debilitating diseases stemming from gene mutations. One of the critical steps to manufacturing cell therapy products is the intracellular delivery of genetic coding molecules to make cells more powerful in fighting diseases.
To address the challenges of intracellular delivery, UCI researchers led by Abraham Lee have developed a microfluidic platform to safely and precisely deliver genetic coding molecules into cells. Called Acoustic-Electric Shear Orbiting Poration (AESOP), their highthroughput nonviral intracellular delivery platform optimizes transfer of cargo sizes with poration, or pore formation, of the cell membranes.
The standard approach for intracellular delivery is to use the molecular machinery of viruses to deliver genetic molecules into cells. However, viral delivery is limited by the size of the molecules it can carry. It also suffers from unwanted side effects from the virus that can be harmful to the patient.
“The ability to achieve intracellular delivery without the use of viruses is a holy grail for many applications, including but not limited to cell therapy – drug discovery processes, stem cell research, gene editing, etc.,” said Lee. “Although there are other nonviral methods, ours is unique in the sense that it can deliver molecules precisely and uniformly to a large population of cells, enabling safe and more effective cell engineering for therapeutic purposes.
“Our technology relies on a series of vortices, which are analogous to micro ‘washing machines,’ that stretch and twist the clothes (i.e., the cells) to make sure every part of it is uniformly absorbing the detergent (i.e., the genetic coding molecules).”
The vortices are formed based on tiny air bubbles trapped in lateral side cavities, something Lee’s lab has developed called lateral cavity acoustic transducers or LCATs. By applying an acoustic energy to the device, the microvortices are formed. Researchers also fabricate electrodes beneath the vortices to apply electrical fields. Lee’s group has demonstrated uniform and precise cell transfection – a procedure introducing foreign nucleic acids into cells to produce genetically modified cells, shown by the intracellular delivery of DNA plasmid, small double-stranded molecules, as well as the gene editing of cells with large genetic-coding plasmid complexes.
Lee and his team were recently awarded a $1.18 million dollar NIH grant to further their AESOP research.
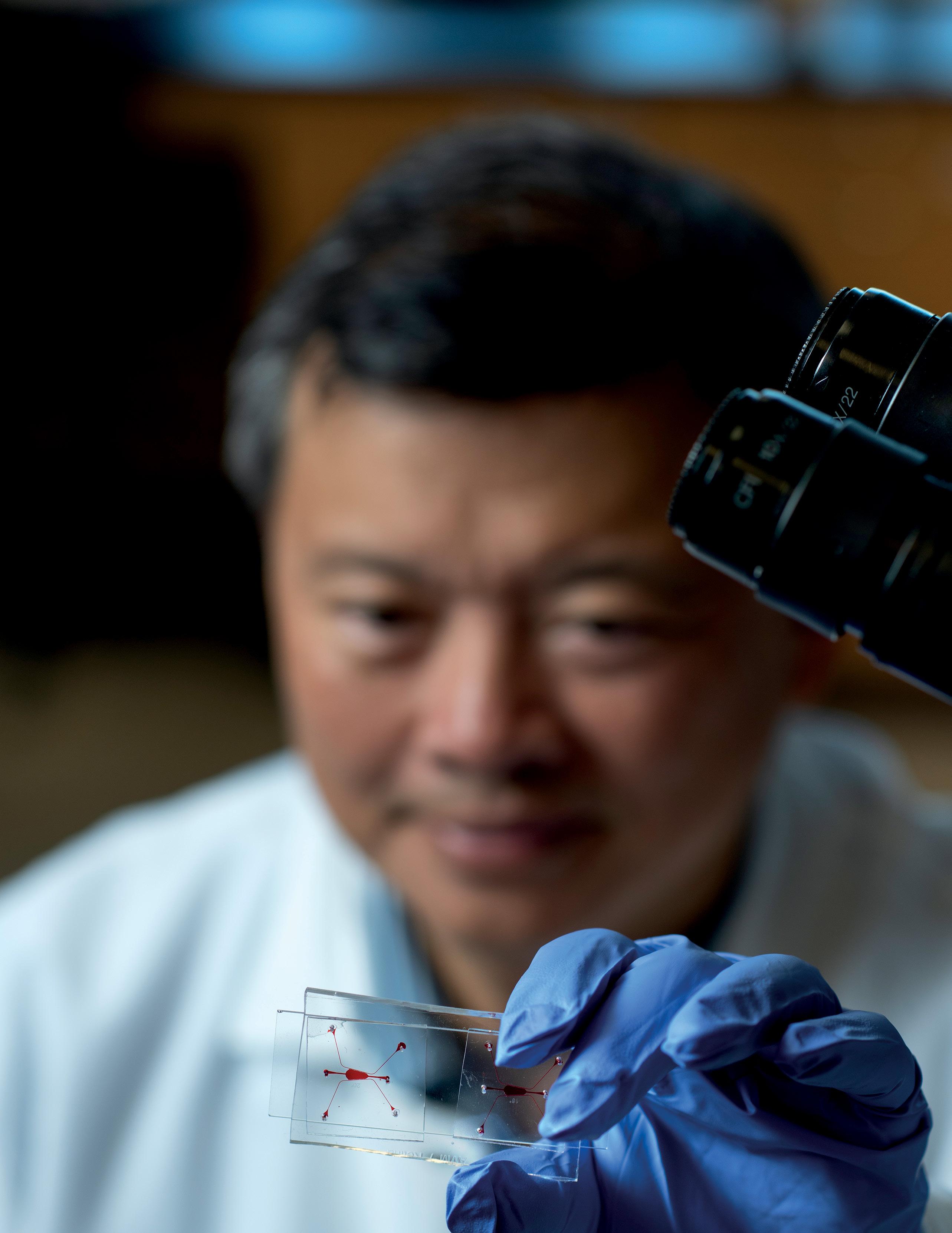
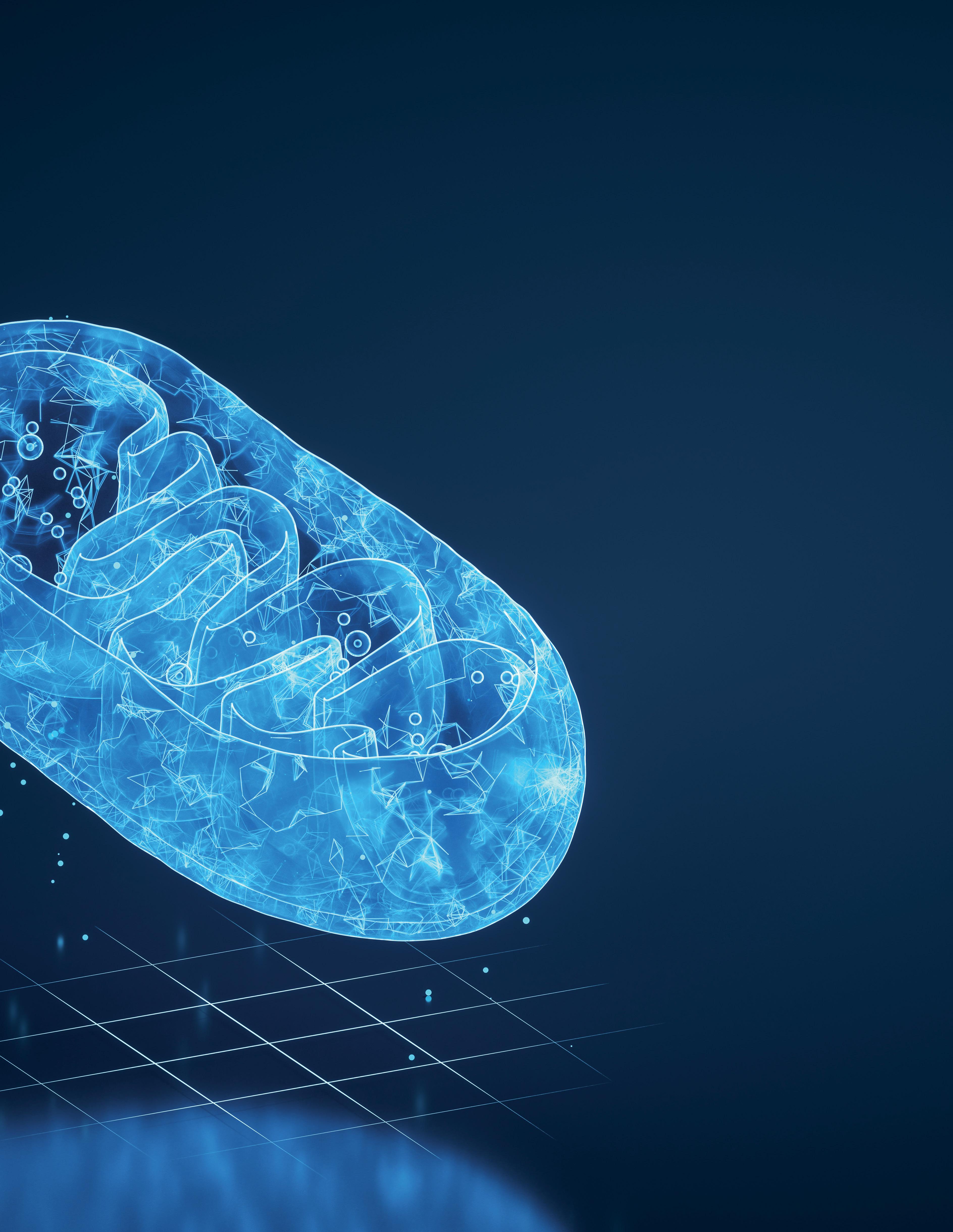
GRADUATE STUDENT CREATES ALGORITHM TO UNLOCK MITOCHONDRIA’S INFLUENCE ON DISEASES
Mitochondria, the organelles inside cells responsible for making energy, hold keys to understanding diseases like cancer, diabetes and Alzheimer’s. Pursuing an interest in studying metastasis – the spread of cancer, Austin Lefebvre, Samueli School graduate student researcher in biomedical engineering, created an algorithm called Mitometer to track mitochondria in live-cell two-dimensional and threedimensional time-lapse images.
Research over the past decade has shown that mitochondria move to the edges of cancer cells to migrate to other parts of the body. Lefebvre began investigating mitochondrial motility in the biomedical department’s Laboratory for Fluorescence Dynamics. What he thought would be a simple task – create an algorithm to study the mitochondria’s movement to the edges of breast cancer cells during migration – ended up taking more than a year and a half of iterative work. Mitochondria are very complex in shape and size, and they vary between cell types and cancers. So making an algorithm to analyze shape, track movement, and work in the general cell biology field proved challenging. “A lot of people have made algorithms before that are very specific for their microscope or cell type, and mitochondria that are specific sizes, specific shapes,” explained Lefebvre. “But none of them are shape or size independent. That was messing up my progress, so I wanted to make it easier for myself and share it with everyone to let them use it too.” What differentiates Mitometer from other programs is its speed, automation and lack of bias. Mitometer only requires the pixel size and time between frames in the time-lapse images to identify mitochondrial motility and morphology. The segmentation algorithm isolates individual mitochondria by removing the background but preserving the shape and size. The tracking algorithm links mitochondria via differences in morphological features and displacement.
“The algorithm and software will make studying these mitochondria a lot easier for researchers,” said Lefebvre. “By using the software, it can help identify the mitochondrial differences, so that down the line, we can have better ideas about how to target the more aggressive types of cancer and other diseases like diabetes, Alzheimer’s, Huntington’s and Parkinson’s.”
FUNDING ENABLES TEAM TO STUDY CELL SIGNALING
The W.M. Keck Foundation has awarded $1 million to biomedical engineering researchers at UCI and Boston University to study cell signaling, which could unlock significant discoveries in human physiology and lead to therapeutic innovations.
Co-principal investigators Chang Liu, professor of biomedical engineering at UCI, and Ahmad (Mo) Khalil, associate professor of biomedical engineering at Boston University, will develop a platform to create biomolecules that enable the systematic study of biased signaling by G-Protein Coupled Receptors (GPCRs). GPCRs are a large class of membrane proteins used by cells to convert extracellular signals into intracellular responses regulating many physiological functions in the human body, including neurotransmission, vision, smell, the endocrine system, and the immune system.
“This award will give us the freedom to pursue new ideas in molecular and cell biology by evolving proteins that precisely modulate GPCRs,” said Liu.
In the classical model, GPCRs act as on/ off switches where a ligand acts to turn on or off all signaling from a GPCR. In recent years, however, this classical model has been expanded through the discovery of biased GPCR ligands, biomolecules that do not simply activate or inhibit all signaling but selectively modulate downstream pathways. In effect, this turns a black-and-white signaling paradigm into “color” by adding another dimension to its possibilities, explained the researchers.
“GPCRs control almost every aspect of human physiology and the ability to selectively activate certain GPCR signaling pathways over others is hypothesized to have major therapeutic advantages, like treating pain or heart conditions with fewer side effects,” said Liu. “Yet whether this selective activation is possible in a general manner, that is still an open question in the field. We are excited to answer that question by leveraging our continuous protein evolution approaches.”
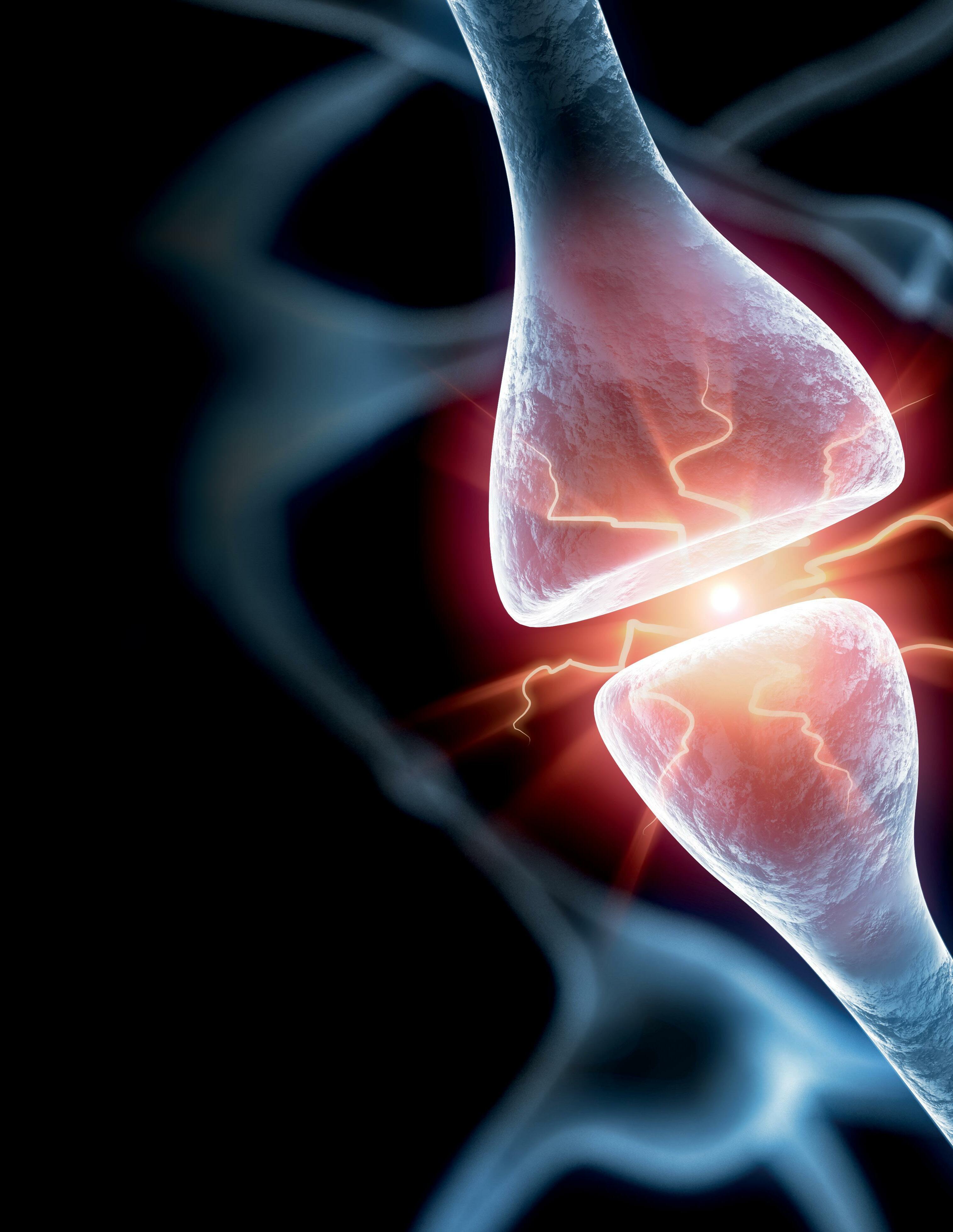
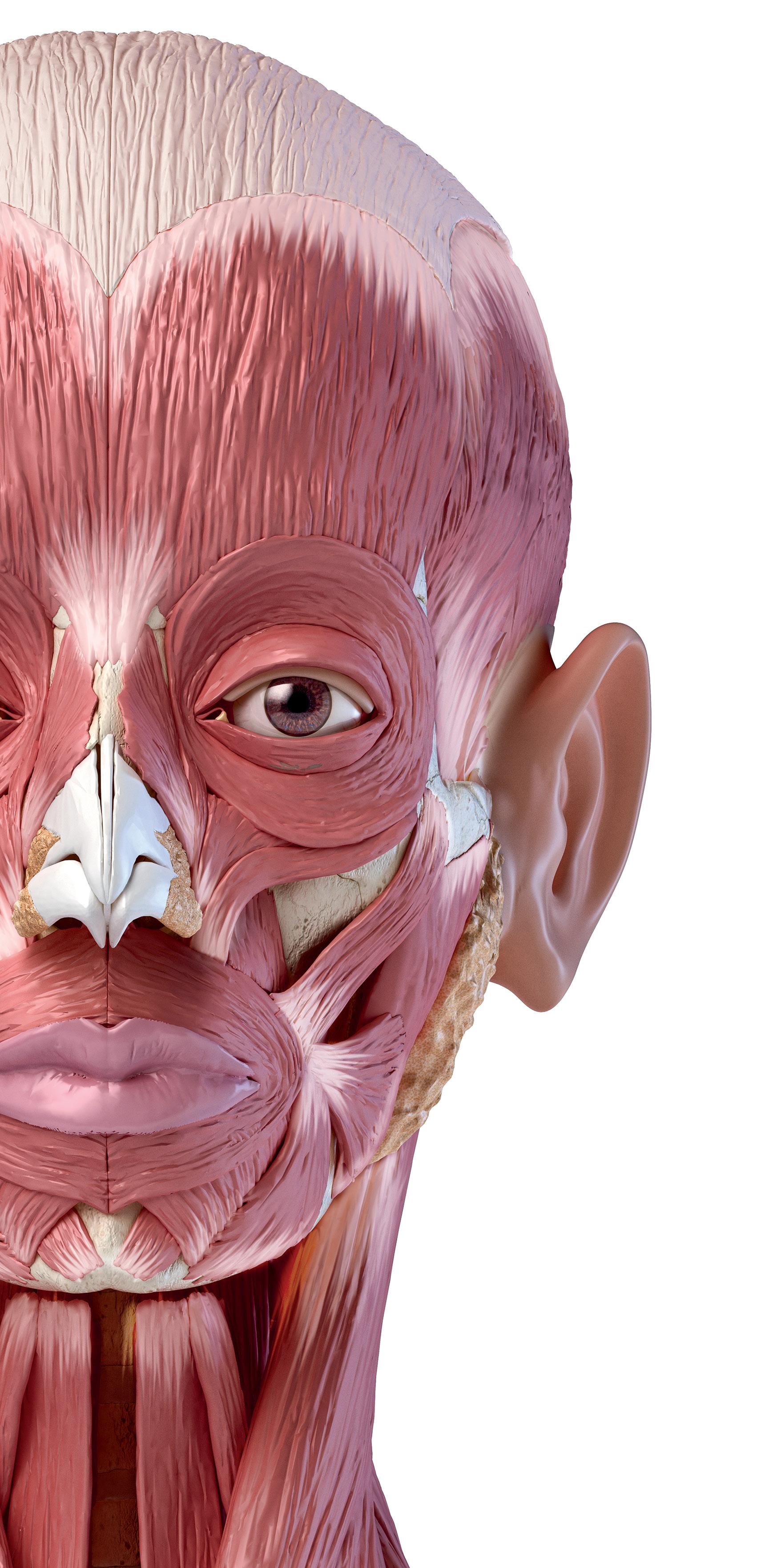
CROSS-DISCIPLINARY RESEARCHERS WILL EXPLORE APPLICATIONS IN REGENERATIVE MEDICINE
The W.M. Keck Foundation has awarded $1 million to an interdisciplinary team of UCI researchers to study the formation and health of cartilages, tissues that are vital to skeletal structures.
A particular focus for the UCI team, which includes scientists from the School of Biological Sciences and Samueli School of Engineering, will be an examination of “peculiar cells” that are giant in size and packed with lipid molecules, according to co-principal investigator Maksim Plikus, professor of developmental & cell biology.
These cells come together to form and provide mechanical structure to cartilages in the nose, ears and other areas of the head, neck and chest. Having detected the presence of fat-rich cartilages in mice, the researchers now intend to conduct a thorough investigation of their developmental biology, biochemistry and biomechanics in hopes of better understanding birth defects of the face and learning how to harness the cells’ unique metabolism in new regenerative therapies for cartilage diseases.
“We have a pretty good basis of understanding about how [cells called] chondrocytes secrete an extracellular matrix to make up the voluminous packing foam-like tissue of ordinary cartilages,” Plikus said. “We are now keenly interested in studying newly found giant chondrocytes that secrete small amounts of extracellular matrix yet contain lipid-filled sacs that get packed together like Lego blocks.”
Fat-rich cartilages are, paradoxically, found to have exceptional mechanical properties, despite the fact that these tissues contain mostly cells and not much matrix, according to co-principal investigator Kyriacos Athanasiou, Distinguished Professor of biomedical engineering.
“A tissue that is so highly cellular should not be as strong and stiff because cells are orders of magnitude softer and weaker than the macroscopic tissue,” he said. “Thus, we believe this work will allow us to unveil new theories in tissue biomechanics.”
Athanasiou’s research group focuses on tissue regeneration therapies to help patients with a host of afflictions, so members are hoping that some of the fundamental knowledge gained in this new project will help in their efforts.
“The work supported by the Keck Foundation is truly exciting because the discovery of these fatty cartilage cells may allow us to understand how certain diseases of craniofacial cartilages may come about,” Athanasiou said. “Equally exciting, this may allow us to consider an entirely different way of making, or ‘tissue-engineering,’ these cartilages – for example, in the nose – by potentially considering fat-filled cells as the ‘engineers’ to fabricate cartilages similar to native tissues.”
INNOVATIVE DNA RECORDER STUDIES DRUG RESISTANCE IN BREAST CANCER CELLS
How do breast cancer cells develop resistance to chemotherapy? How does DNA damage change cell fate? These are key questions Theresa Loveless, UCI postdoctoral researcher in biomedical engineering, seeks to answer by creating innovative DNA recorders to study cells’ histories and behaviors.
The NIH has granted Loveless a Maximizing Opportunities for Scientific and Academic Independent Careers Postdoctoral Career Transition Award to Promote Diversity. The program supports early career, independent investigators from diverse backgrounds conducting research in NIH mission areas. The long-term goal of the program is to enhance diversity in the biomedical research workforce. The award includes $100,000 per year for the one or two years of the mentored phase and up to $249,000 per year for three years for the independent phase.
“I’m grateful that the study section and program official thought my proposal had promise,” said Loveless, who became disabled in graduate school. She developed persistent pain and weakness in her hands and wrists due to severe tendinitis. By strictly limiting movements that cause injury, she is now able to do experiments for about eight hours per week. She has learned to find resources that have let her stay in science, and she has been engaged in increasing representation for the disability community.
If Loveless secures an independent faculty position, she will take the funding with her to her own lab. She will continue developing her new DNA recorder, called Prime Editor CHYRON or peCHYRON, which will offer deeper insight into the lives of cells. peCHYRON is based upon the first DNA recorder Loveless created with adviser Chang Liu, called Cell History Recording by Ordered Insertion (CHYRON).
Unlike CHYRON, peCHRYON causes very little DNA damage. It can also record more information in parallel and at higher fidelity, providing deeper insight into the whole history of the cell. “It’s a better recorder,” Loveless explained. “I’m hopeful that, in the long term, we can start to really understand how something like endogenous DNA damage, which is damage that happens in the natural process of cell growth and division, affects the cells’ later development.”
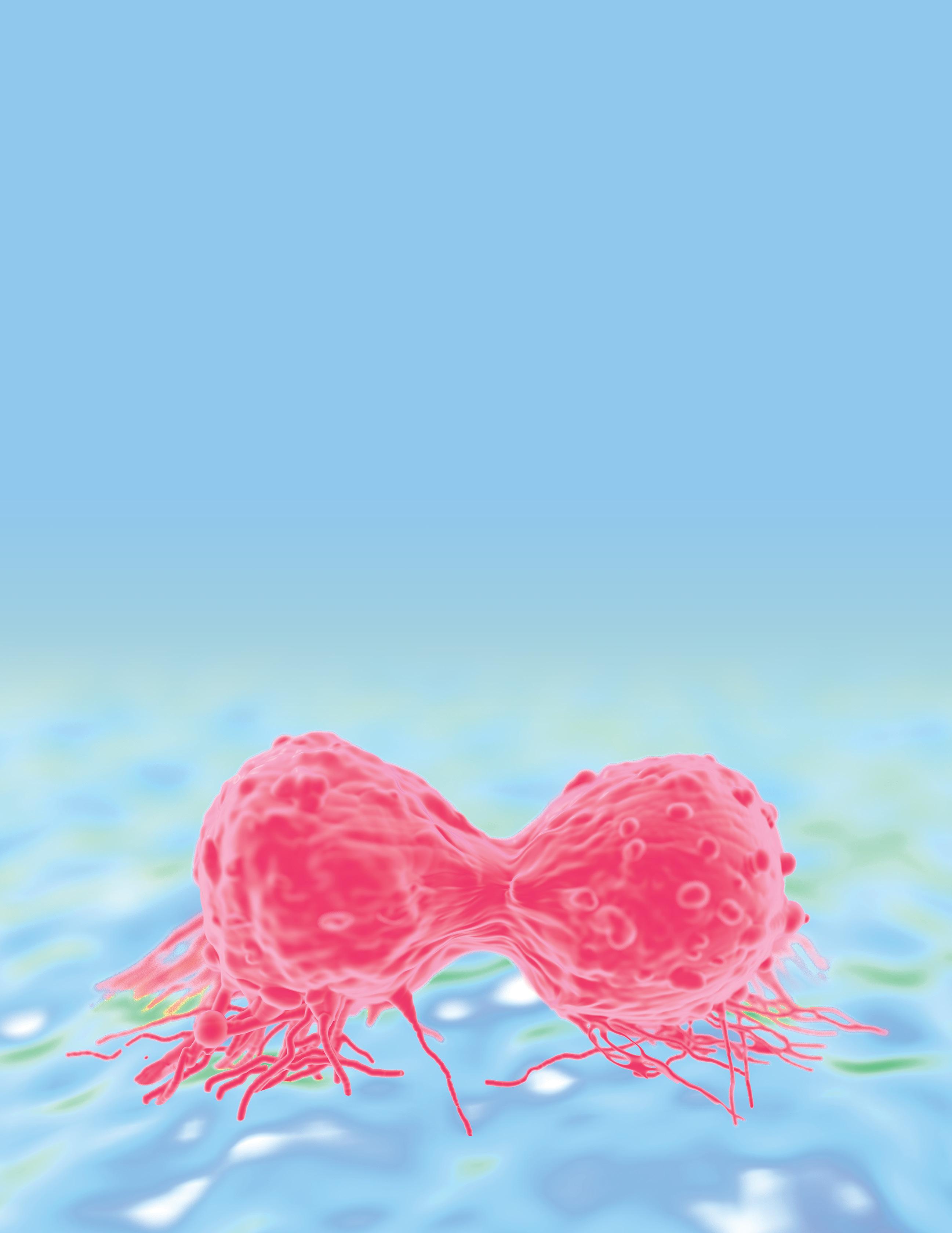
RESEARCHERS DEVELOP NONINVASIVE OPTICAL BIOPSY TECHNOLOGY FOR WOMEN
Up to 50% of women going through menopause experience symptoms that negatively affect their general health and sexual function. Increasingly, energy-based devices, such as lasers including C02 micro-ablation, are emerging to treat symptoms that include vaginal atrophy and distressing urinary symptoms. To better understand the full effect of laser treatment on vaginal tissue, UCI biomedical engineering researchers are developing a new noninvasive intravaginal imaging system that could serve as an optical biopsy tool, ultimately enabling individually tailored screening, treatment and monitoring for patients.
“We are working on a point-of-care, multifunctional endoscope that can obtain real-time simultaneous information on structural, vascular and biomechanical changes before, during and after vaginal laser procedures,” said Zhongping Chen, professor of biomedical engineering.
A pioneer in biophotonics, Chen is working with an interdisciplinary team of scientists, engineers and physicians. The group recently received a $2 million R01 grant from the NIH.
The new imaging system combines optical coherence tomography and OCT angiogram into one technology and will function as an optical biopsy, providing objective, noninvasive scientific parameters to assist clinical practitioners as well as governing bodies (including the FDA) to determine best practices. Safe and effective therapies to relieve progressive menopausal symptoms over a long period are critical.

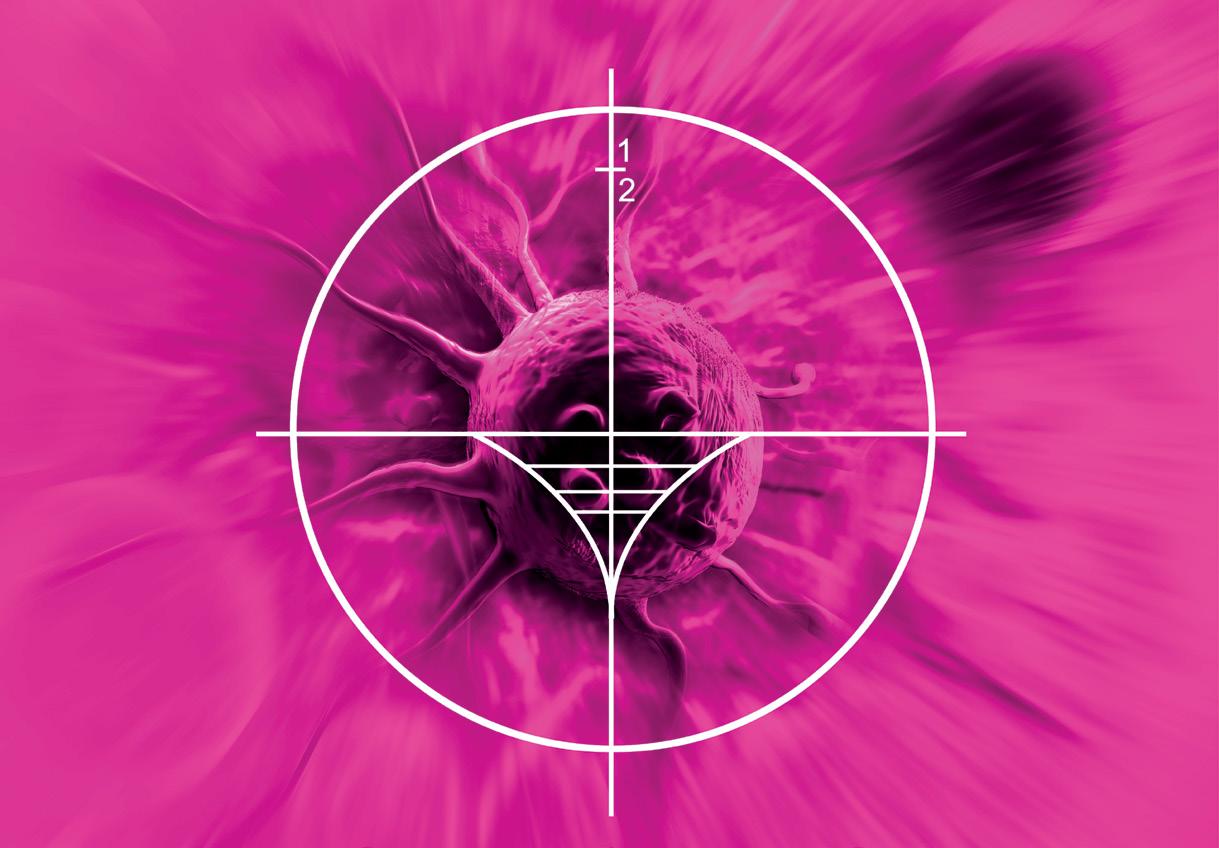
TUMOR TISSUE PROCESSING TECHNOLOGY EARNS FUNDING
UCI researchers have received a $1.1 million NIH award to further their work on an integrated microfluidic platform that could help dramatically change the way tumor tissue is clinically evaluated. With three years of funding support, the team led by Jered Haun, associate professor, will be able to test the technology on human tissue samples. The results could help scientists make progress in disease diagnosis and drug development.
Solid tumors are complex mixtures of different cell types, and these differences are key factors driving disease progression, metastasis and drug resistance. “Assessing cellular heterogeneity and identifying key driver cells are critical for understanding tumor biology, and for creating the most powerful clinical diagnostics,” said Haun. “Targeted therapies must be directed toward the most important cell types if effective cures are to be achieved. With this technology applied in clinical settings, we hope to help usher in an era of precision molecular medicine.”
Currently, single cell analysis studies are hindered, as tissues must first be dissociated into single cell suspensions using methods that are often inefficient, labor-intensive and highly variable. Importantly, certain cell types can be released more easily than others, which will bias the single-cell analysis assay and lead to incorrect conclusions.
The new platform will combine four separate microfluidic device technologies that Haun has pioneered. The devices were designed to work sequentially, starting from tissue specimen digestion, through dissociation and filtration to finally extracting single cells. Any remaining cell clusters would be recirculated back into the front end of the device to maximize cell recovery. Single cells will be continuously extracted from the system as soon as they are ready, within minutes after dissociation, to prevent overtreatment and maintain viability.
“This multifaceted approach will enable us to tailor flow properties and shear forces to the appropriate magnitude and size scale, resulting in gradual and ultimately complete breakdown of tissue in a fast, efficient and gentle manner,” said Haun.
The researchers will test the devices using human breast, pancreatic and prostate tumor tissue specimens.
NEW CARDIAC IMAGING TECHNOLOGY TO STUDY PULMONARY ARTERIAL HYPERTENSION
Pulmonary arterial hypertension (PAH) is a rare but serious condition in which high blood pressure occurs in the arteries that carry blood from the heart to the lungs. A progressive disease, it can lead to heart failure. To diagnose and monitor PAH, physicians conduct right heart catheterization, an invasive test to measure the pressure inside the heart and lungs. UCI’s Dr. Arash Kheradvar and his team have developed an alternative noninvasive imaging technology that could be used more widely to help physicians diagnose and follow up this condition in patients.
Kheradvar, professor of biomedical engineering, has been working over 10 years on the technology. Called echocardiographic particle image velocimetry (echo-PIV), it uses high frequency sound waves to scan the blood velocity and other characteristics of the heart. He has received a five-year $3.3 million award from the NIH’s National Heart, Lung and Blood Institute to study the energy state of the right ventricle of patients with pulmonary arterial hypertension, using echo-PIV, and to test the feasibility of this approach for monitoring disease progression and regression. Noninvasive measurement of the right heart’s energy state could provide objective insights into the functional status of the heart, instead of the current use of right heart catheterization.
“We believe much more information regarding the hemodynamics of the right heart can be inferred from noninvasive echo-PIV, helping us understand the unidentified mechanisms of the disease,” said Kheradvar.
Kheradvar is working with an interdisciplinary team on the project and will test the technology at Cedars Sinai Medical Center.
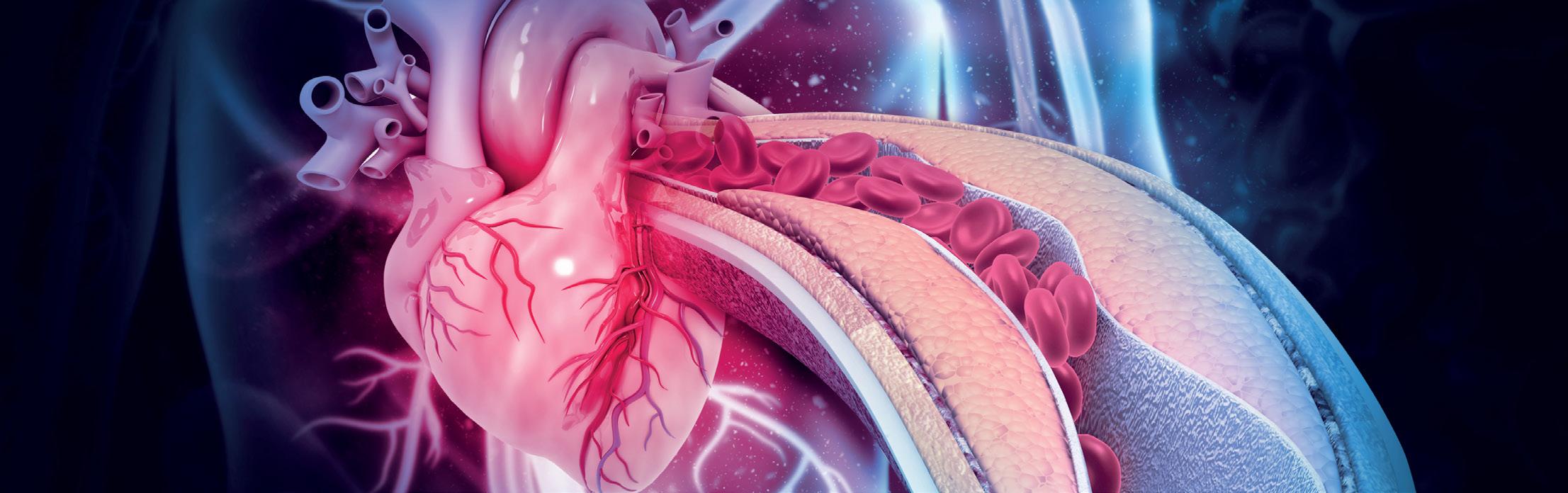
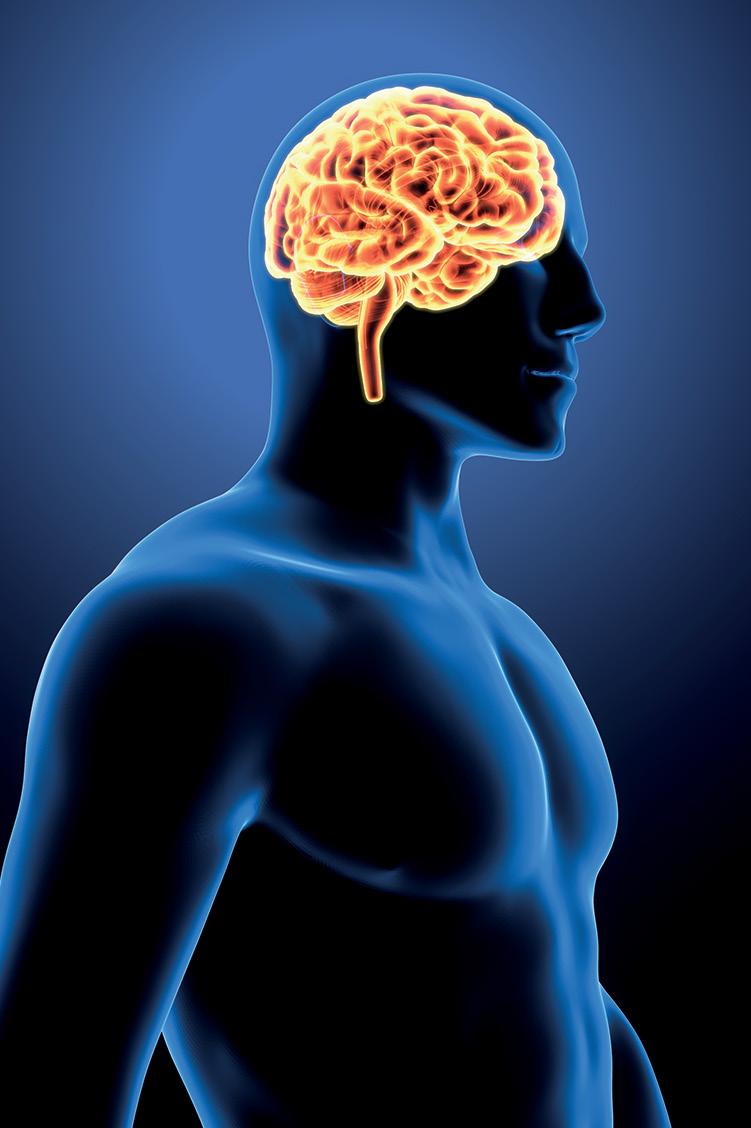
FUNDING SUPPORTS CHRONIC STROKE RESEARCH
Over 8 million people in the U.S. are living with chronic stroke. The resulting disabilities lead to significant public health costs and decreased quality of life. With no means to satisfactorily restore functions after stroke, new effective regenerative approaches are much needed. Therefore, a new concept of “engineered neural networks” (ENN) is being developed by BME Professor Zoran Nenadic and Dr. An Do in UCI’s neurology department.
The ENN is envisioned to contain neurons derived from human adult stem cells and be structured to have precise inputs and feedback loops. These connections will enable the ENN’s integration with other brain and body areas and thereby learn behaviors. Ultimately, this integration process can lead to ENNs replacing stroke-damaged brain and any associated functions.
Before this goal can be achieved, the first step is to develop a benchtop testbed cultured neural network (CNN) and verify that it can be trained to perform an arbitrary sensory task and motor behavior. The researchers recently received funding from the National Science Foundation to conduct the study. Successful completion would demonstrate that CNNs can be trained to encode arbitrary behaviors and interact with other brain and body areas. This would justify further research to pursue the development of ENNs and methods to implant such systems into the stroke-damaged brain. Ultimately, this may restore brain resources in a functionally meaningful manner, which may in turn lead to ground-breaking regenerative treatments for stroke rehabilitation.