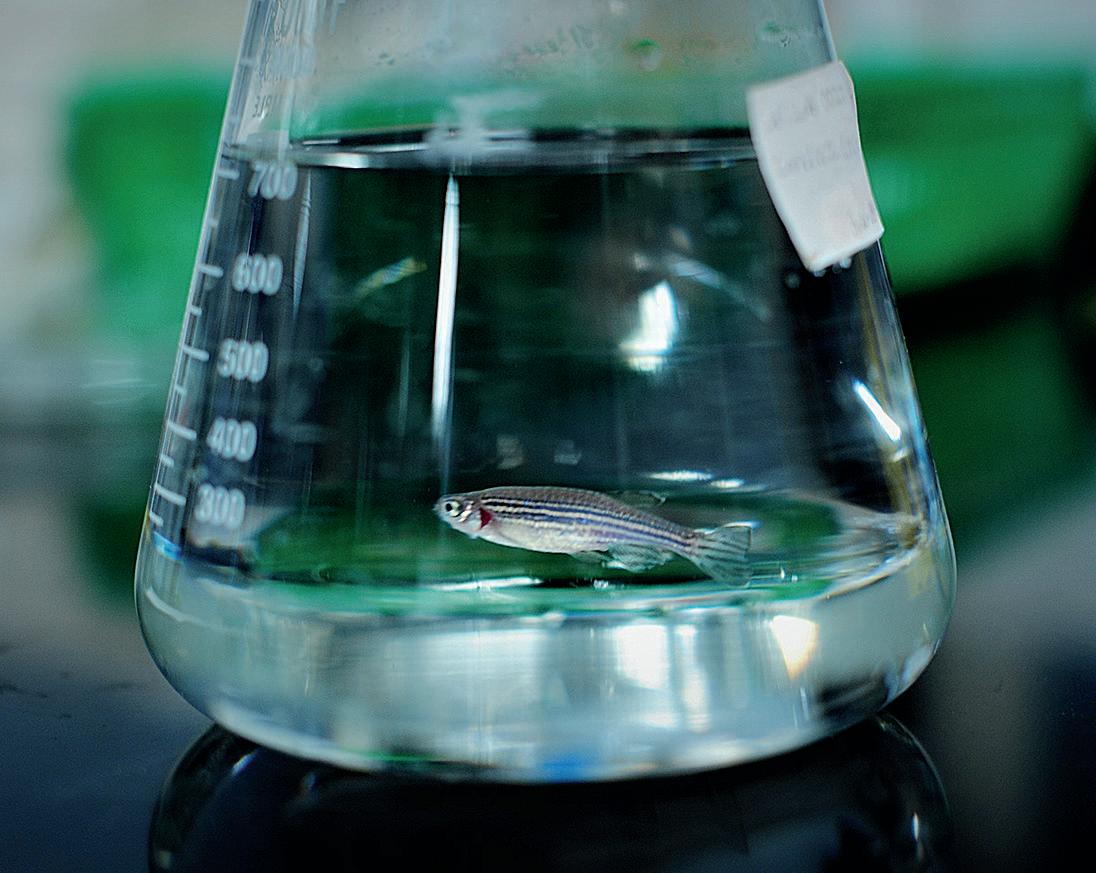
11 minute read
Highlights
Cao Secures Funding for Brain Biosensors and for Zebrafish Research
A team led by Hung Cao received $1 million from the National Science Foundation to design integrative approaches for measuring brain activity during social interactions in sleep-disrupted animal models.
Early-life sleep disruption has been shown to affect the development of complex social behaviors in prairie voles, the studied model, impairing social bonding in a manner similar to autism in humans.
Cao, assistant professor of electrical engineering and computer science, will create neural biosensor microprobes that can simultaneously – and in real time – detect two key neurotransmitters governing excitation and inhibition. The ratio of these neurochemicals, L-glutamate and gamma-aminobutyric acid, holds important information about brain control of social interactions in healthy and autistic individuals. The wireless system will be combined with EEG to track electrical activity in the brain.
“We know that sleep disruption in early life affects the ability to form bonds with partners in voles, but we don’t know why or what’s going on in the brain’s chemistry,” said Cao. “We need dedicated devices to measure the excitatory/ inhibitory balance in vivo during social interactions, with optimal temporal and spatial resolution. Such tools do not currently exist.”
Cao’s co-principal investigator on the grant is Dr. Miranda Lim, a neurologist at Oregon Health and Science University who examines sleep disruption in the developing brain.
“We hope to solve a longstanding mystery in neurobiology about the critical contribution of sleep to brain development,” said Cao. “The outcomes could help reveal how neural processes may go awry in neurodevelopmental disorders, and enable the next generation of neural prostheses, therapeutics and brain-machine interfaces.”
Cao also won a National Institutes of Health grant to further his research on electrophysiology assessment in zebrafish. The Small Business Innovation Research Phase II award from the NIH Office of Research Infrastructure Programs advances Cao’s work with startup Sensoriis, Inc., which develops sensing solutions to address health care problems. The two-year $1.5 million grant includes a $477,394 sub-award that will directly fund Cao’s research.
Zebrafish, which have physiological similarities to humans, have long been used for understanding human cardiac and neurological systems and for drug screening. Their small size, low maintenance costs, quick regeneration, conserved genome and optical transparency make these vertebrates ideal for experimental models.
Cao’s project focuses on creating novel devices and systems that can provide reliable electrocardiogram and electroencephalogram data from both adult fish and larvae; building cloud-based systems that can process, interpret and study large-scale data; and designing cardiac and neurological studies and drug screening methods that use zebrafish models and the study’s novel tools.
Tseng Develops New Interlayer RF Resonators for Wireless Biosensing
Noninvasive, wireless medical devices and biosensors have emerged in recent years as powerful tools to track human performance, individual
biological markers and wellness. These small, flexible devices have enabled easy monitoring of physiological parameters, such as heartbeat and glucose levels, but the biosensors in these devices often are large, require a lot of power, and have short life spans and low sensitivity.
Peter Tseng, assistant professor of electrical engineering and computer science, is working to design and develop wireless passive radio frequency identification device (RFID)-enabled biosensors with programmable sensitivity and selectivity (the ability to differentiate molecules from one another). These sensors will require no electronics at the sensing node, allowing their implantation into a host of new environments and systems. In December 2019, he won a $500,000 CAREER award from the National Science Foundation to develop a multifunctional interlayer-RF resonator as a platform for passive and wireless biosensing.
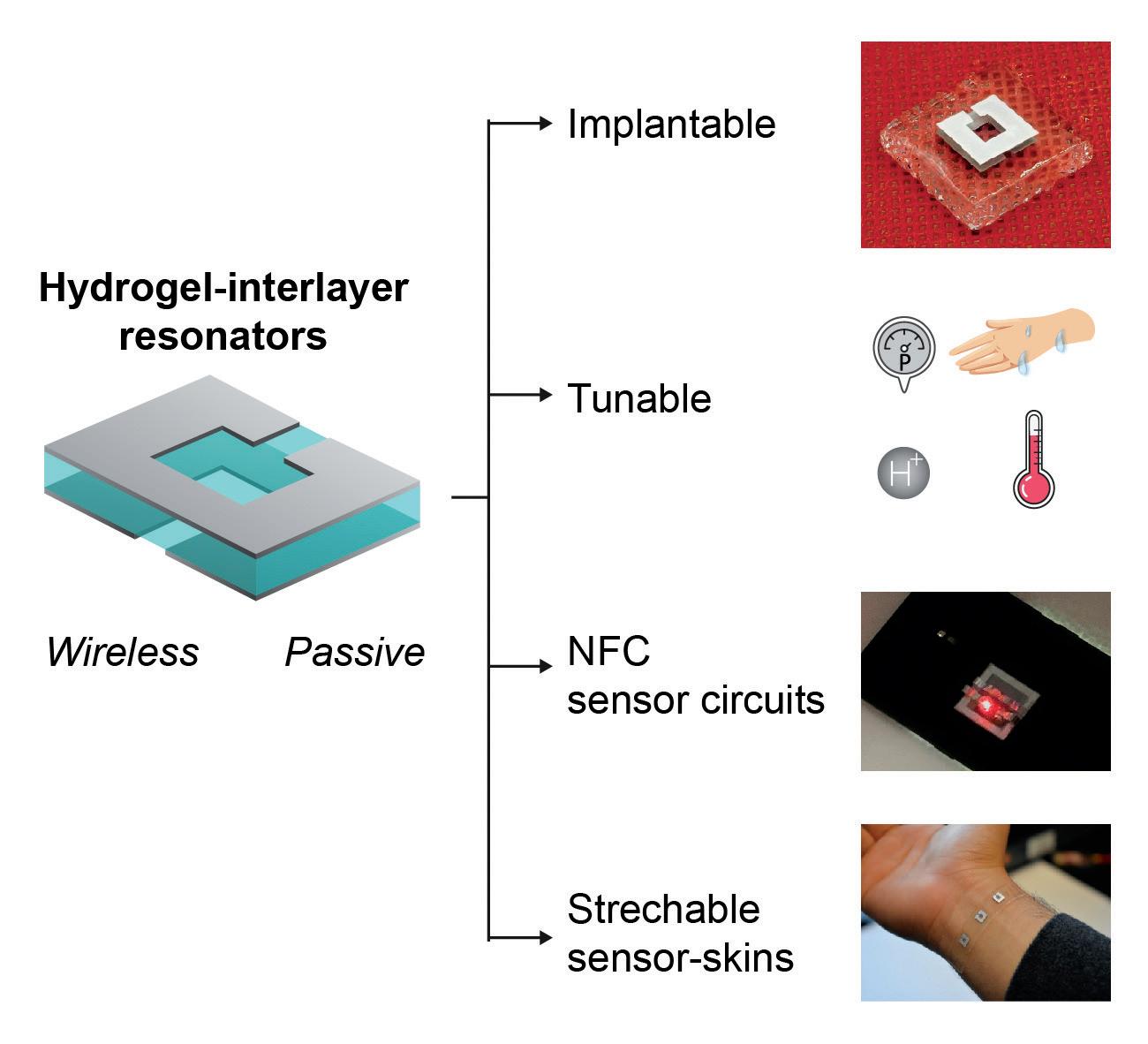
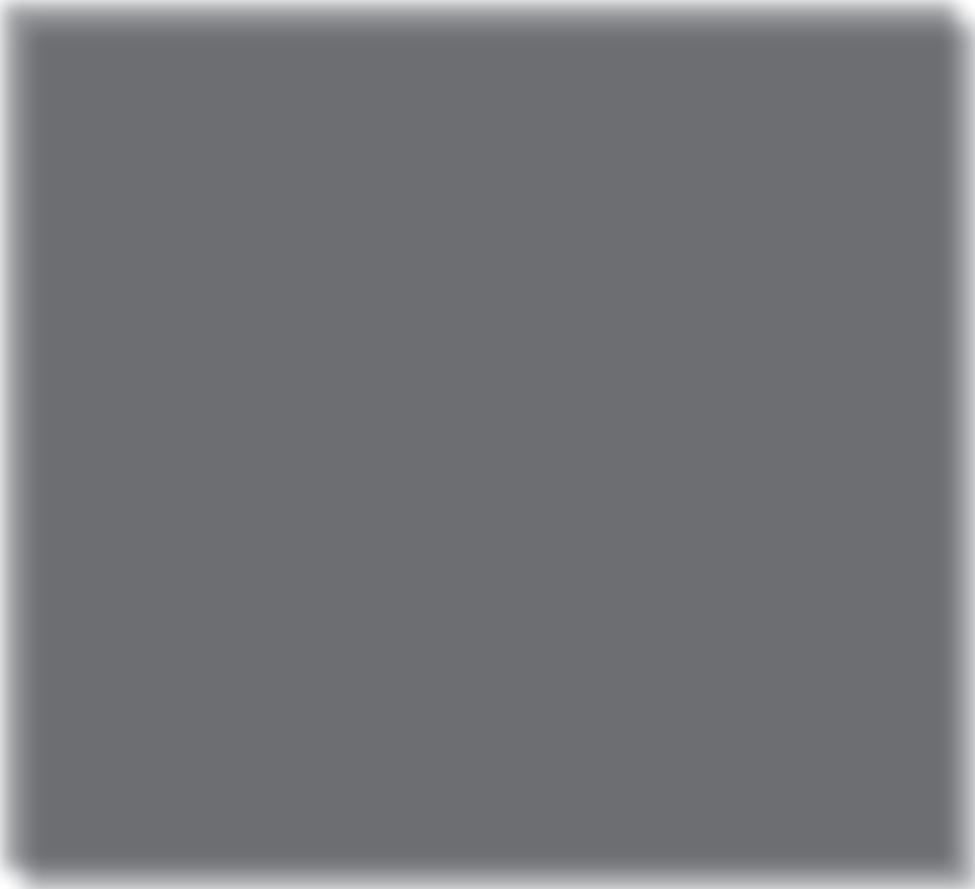
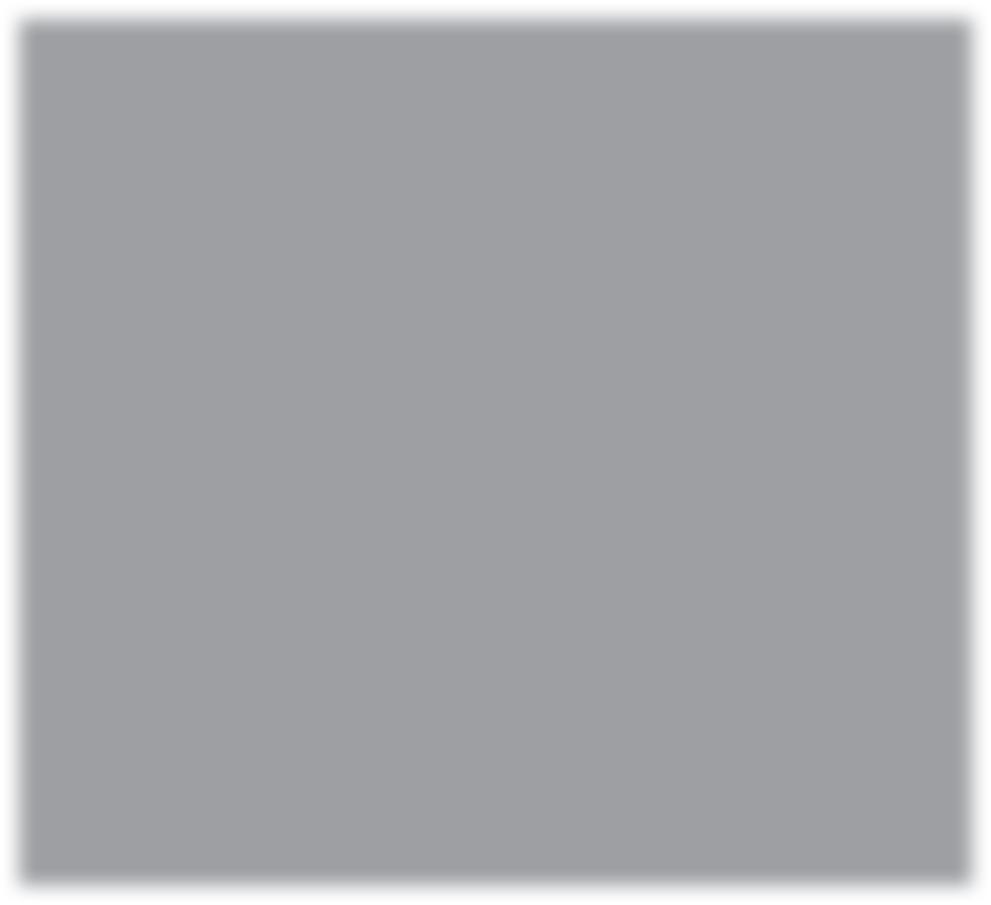
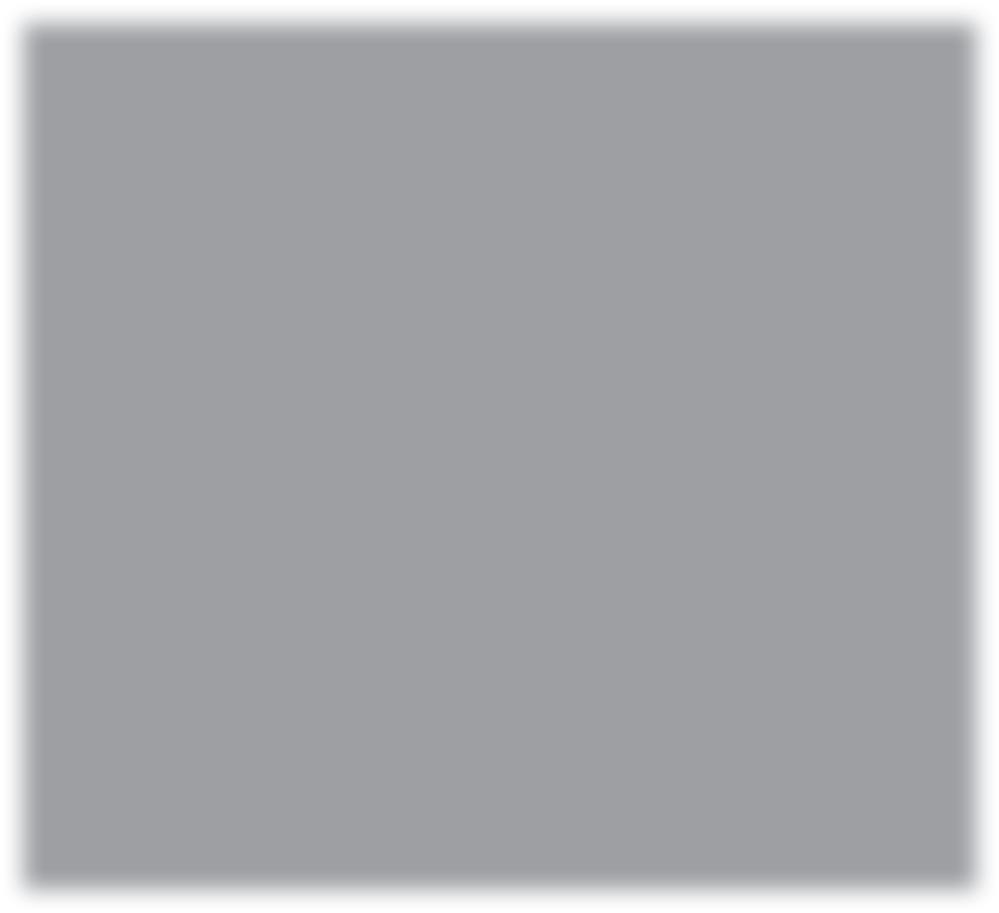
These new sensors will contain an intermediate layer of nanoporous materials that will absorb, swell or deform in the presence of specific chemical stimuli. This will cause their resonant spectra – or properties, including frequencies – to change, providing enhanced detection mechanisms. Proposed interlayer materials include membranes, separators, deformable materials and temperature/pH/metalresponsive polymers, many of which have long lifetimes and a lack of degradative mechanisms.
The next-generation biosensors will utilize sensing modalities that can support inherent wireless readout and robust, long-term operation. They will be attached, embedded or potentially even implanted, integrating with living systems in new ways and enabling new applications in wireless health, Tseng said.
Tseng will also conduct and analyze theoretical and computational studies on how the unique electromagnetic and bio-interactive physics of specific materials combine to influence sensor performance.
The five-year grant includes an outreach component that focuses on training a new generation of younger, and often underrepresented, students. Skills including fabrication, scanning electron microscopy, experimental methodology, data analysis, modeling and computer-aided design are being incorporated into UC Irvine summer programs like the Samueli School’s FABcamp for middle-school students.
“We are developing next-generation, wireless biosensors with tunable sensitivity and selectivity that can interface with living systems,” said Tseng. “This award from the NSF is a huge milestone toward accomplishing our goals.”
[HIGHLIGHTS]
Researchers Develop ‘Lab on a Chip’ for Personalized Drug Efficacy Monitoring
UCI researchers and collaborators have developed a lab-on-a-chip platform to facilitate continuous, inexpensive, rapid and personalized drug screening.
The technology is capable of evaluating the effectiveness of treatments on cancer cells without bulky readout equipment or requiring the shipment of samples to labs. The scientists’ work was published in the American Chemical Society journal Analytical Chemistry.
“There is an ever-present need for simplified and low-cost identification of a patient’s personal cancer resistance and medication efficacy before and throughout treatment,” said senior author Rahim Esfandyarpour, assistant professor of electrical engineering and computer science, as well as biomedical engineering. “We envision our work as another step toward potentially enabling the personalized screening of drug efficacy on individual patients’ samples, possibly
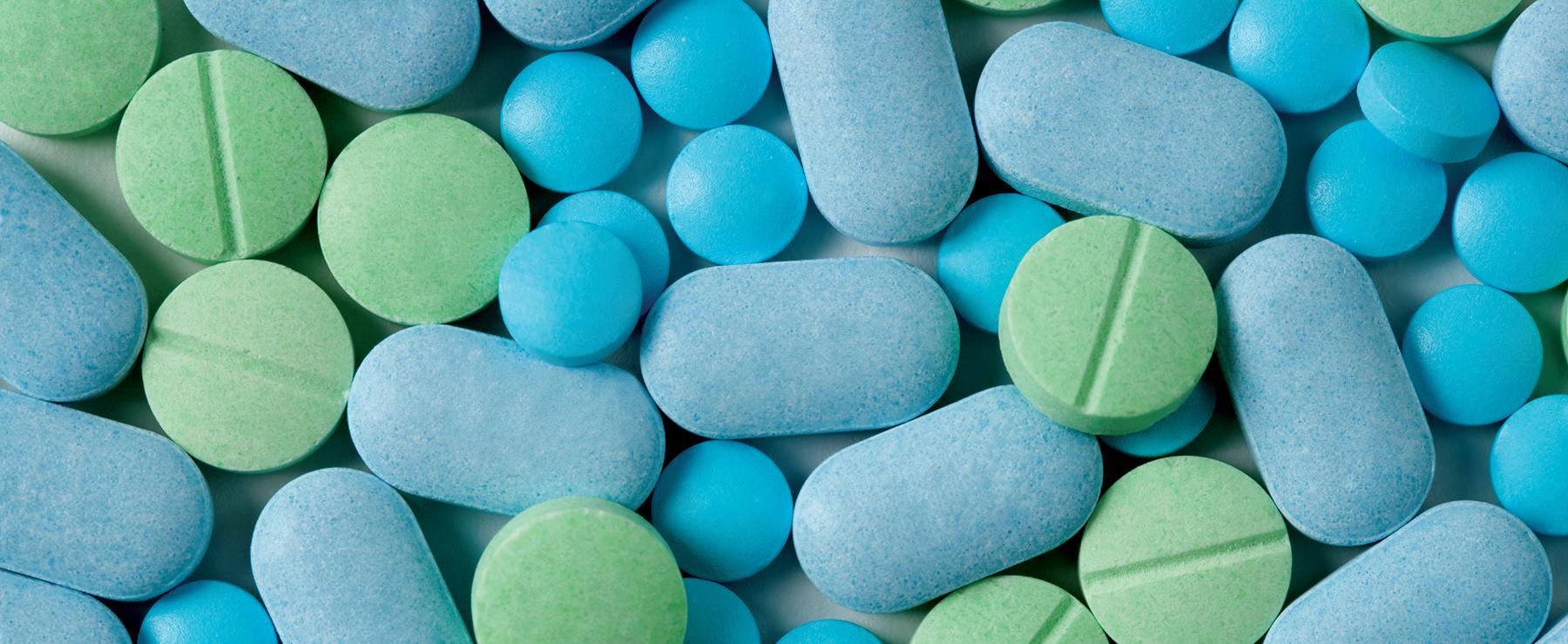
leading us to a better understanding of drug resistance and the optimization of patients’ treatments.”
He said that most current approaches to drug efficacy testing require expensive imaging, lab work and large-scale cell culture experiments. “Our platform is an initial prototype that we hope to further develop in the future into a personalized medicine tool for cancer patients facing drug resistance,” said co-author Vanessa Velasco, a Stanford University postdoctoral researcher.
The lab-on-a-chip technology employs advanced electrical and electrochemical techniques to precisely manipulate cancer cells of interest in parallel with the continuous characterization of the potential effectiveness of therapeutic agents custom-made for patients. The end result should greatly reduce the time and cost associated with treating cancer.
Burke’s New Instrumentation, Novel Approach Allow a Noninvasive Look inside Living Cells
Electrical engineering professor Peter Burke’s efforts to noninvasively peer inside living cells and measure their metabolic activity was boosted this year by a grant from the U.S. Department of Defense Army Research Office.
The one-year, $282,611 Defense University Research Instrumentation Program (DURIP) grant is funding a deep-subwavelengthresolution optical microscope to upgrade Burke’s inverted light microscope/ AFM/scanning microwave microscopy instrumentation.
Several years ago, Burke helped develop a broadband calibration technique that could successfully measure, with nanoscale resolution, the properties of nanowires, nanotube quantum dots and other nanoelectronic components on top of and inside of semiconductor wafers. Now, he and several colleagues are using a similar approach to noninvasively peer inside living cells and measure their metabolic activity. Burke and colleagues from UCI, France’s Institute of Electronics, Microelectronics and Nanotechnology, and the University of Pennsylvania, demonstrated a direct, ultra-highbandwidth nanoscale electronic interface to the interior of living cells, one that can calibrate and measure electrical properties of a cell during all phases of the cell life cycle. This could have important implications for unlocking the mysteries of aging, cancer, diabetes and neurodegenerative disorders, as well as developing medical treatments and new drugs. Specifically, the nanoelectric interface provides simultaneous fluorescence and electronic imaging of vital cellular properties such as the mitochondrial membrane potential. The probe, which is in contact with living cells, could be used in a variety of analyses, including electronic assays of membrane dynamics, nanoelectronic actuation of cellular activity and tomographic (X-ray-like) nanoradar imaging of the form and structure of vital organelles in the cytoplasm, the substance between the cell membrane and the nucleus. This is a “powerful new way to probe living systems using nanoelectronics that enables a direct nanoscale electronic interface to living cells,” Burke said.
The DURIP is funding a sophisticated optical microscope with resolution down to 50 nanometers – about 10 times finer than its predecessor. Called an Airyscan, it images live cells with high sensitivity and low light intensity.
“We are very pleased to be able to upgrade our existing state-of-theart scanning microwave microscope platform with the latest in Airyscan deep subwavelength optical imaging,” Burke said. “It is gentle enough to allow the imaging of live cells with nanoscale resolution without damaging them, and is allowing us to build the first integrated platform for this type of groundbreaking research.”
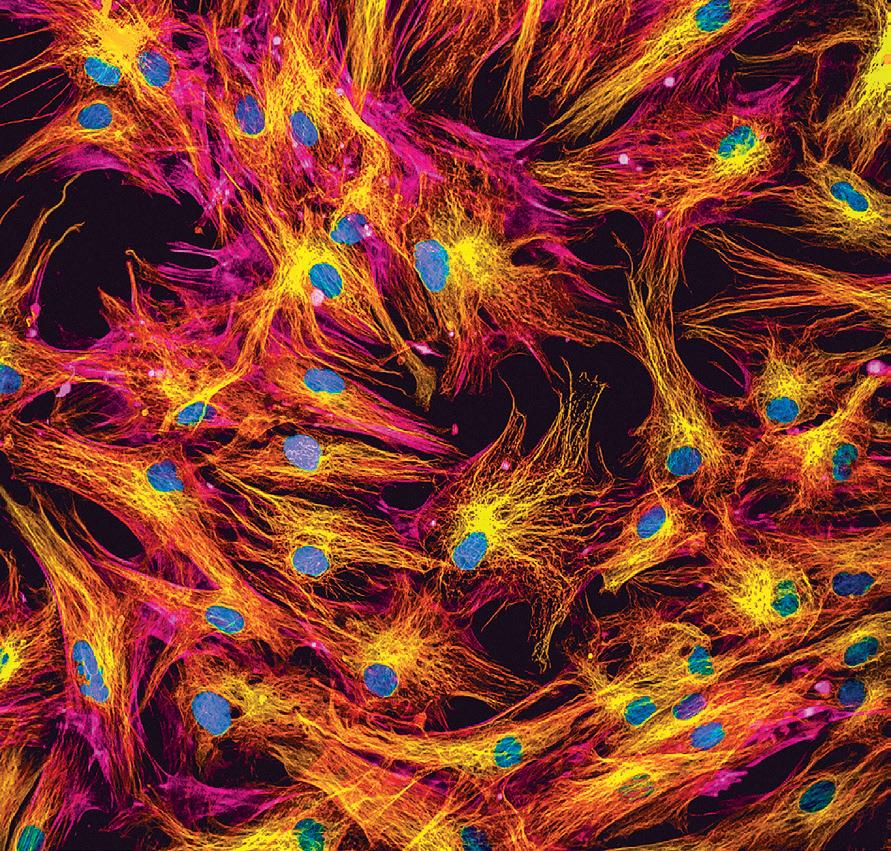
[HIGHLIGHTS]
Aghasi Explores Terahertz Electronics
The capabilities of electronic circuits vary based on the circuits’ frequency – how many light waves can move past
a certain point in a given time. Twenty years ago, the maximum frequency of electronic circuits was about 100 gigahertz. Today, thanks to advances in semiconductor technologies and their scaling, integrated electronic circuits have the potential to achieve much higher frequencies – in the millimeter wave and terahertz frequency ranges. These extremely high frequencies enable a slew of new applications in high-speed computation, communications and more.
Over the last decade, there has been increased interest in designing systems that operate in the terahertz frequency range (300-3000 GHz), but complexity of design and measurement, as well as technology limitations, have slowed successful implementation. “The terahertz region is somewhat new to the electronic circuit, since technology advances – essentially faster transistors and development of design methodologies – have allowed operation in this region only
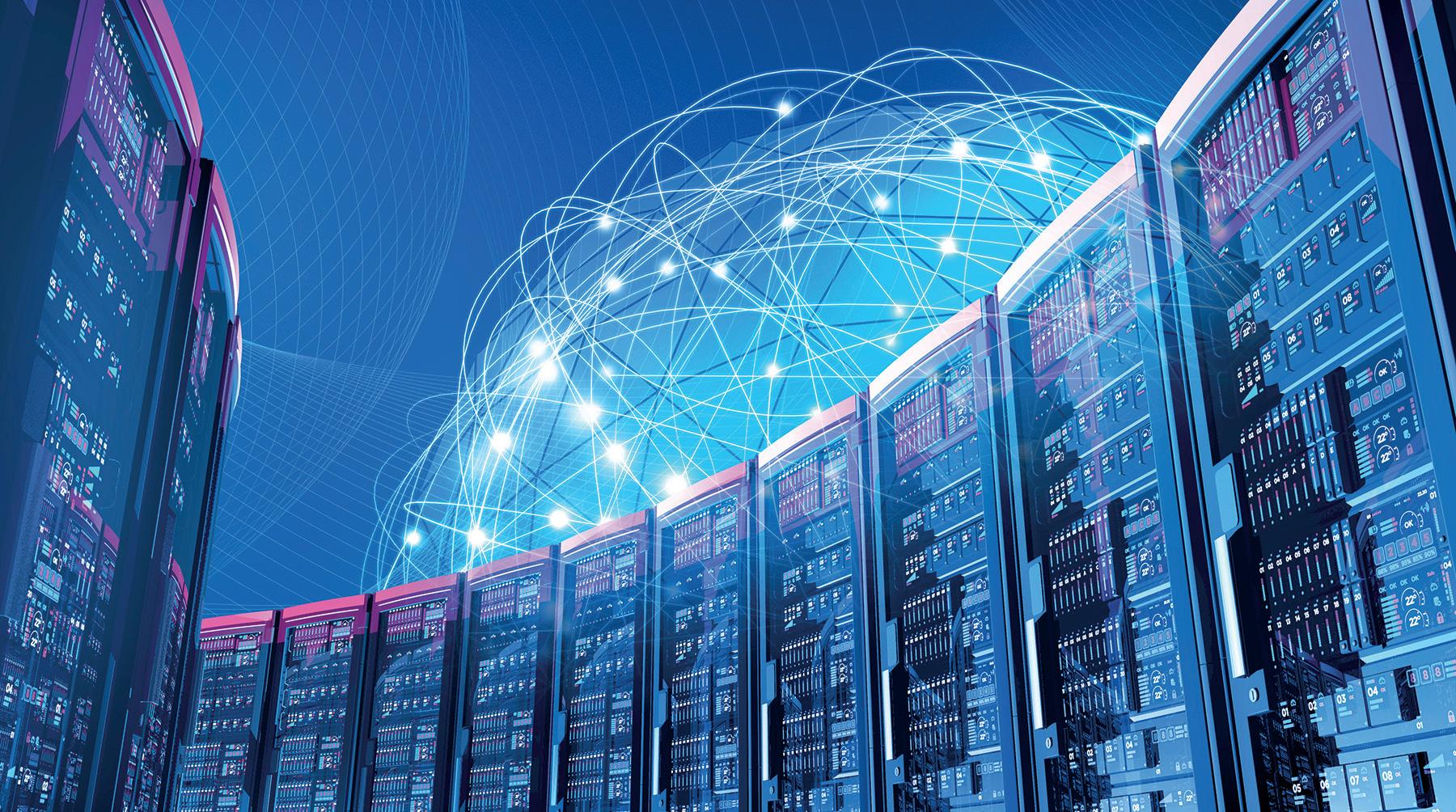
recently,” said Hamidreza Aghasi, assistant professor of electrical engineering and computer science.
Aghasi researches processes and techniques that can help overcome these challenges and generate higher frequency signals. In a paper published in Applied Physics Reviews, he details specific phenomena in electronics – including nonlinear processes and wave propagation techniques – that can help push previous limits of operation into new territory, enabling power-efficient, low-cost and portable circuits for emerging applications.
The operation of electronic circuits at the terahertz range is advantageous because the terahertz range has a smaller wavelength compared to lower frequencies, which allows for better resolution in imaging applications. In addition, communication circuits that operate in this frequency range have a larger bandwidth and can achieve higher speed. And finally, the terahertz frequency range is home to many molecular finger-print qualities that make it a good candidate for performing molecular spectroscopy.
Integrated electronic circuits offer the least expensive technology platform to mass produce terahertz-based systems and devices while maintaining high reliability. They are compact in size and extremely power efficient.
“Terahertz technology has many applications in high-resolution biomedical and space exploratory imaging, communications, sensing, short-range radars, molecular spectroscopy, highprecision atomic clocks and more,” Aghasi said. “This research introduces the fundamental ideas that can be adopted in order to realize each of these applications based on electronic circuits.”
UCI Engineers Develop a New AI-based Method for Analyzing Echocardiograms
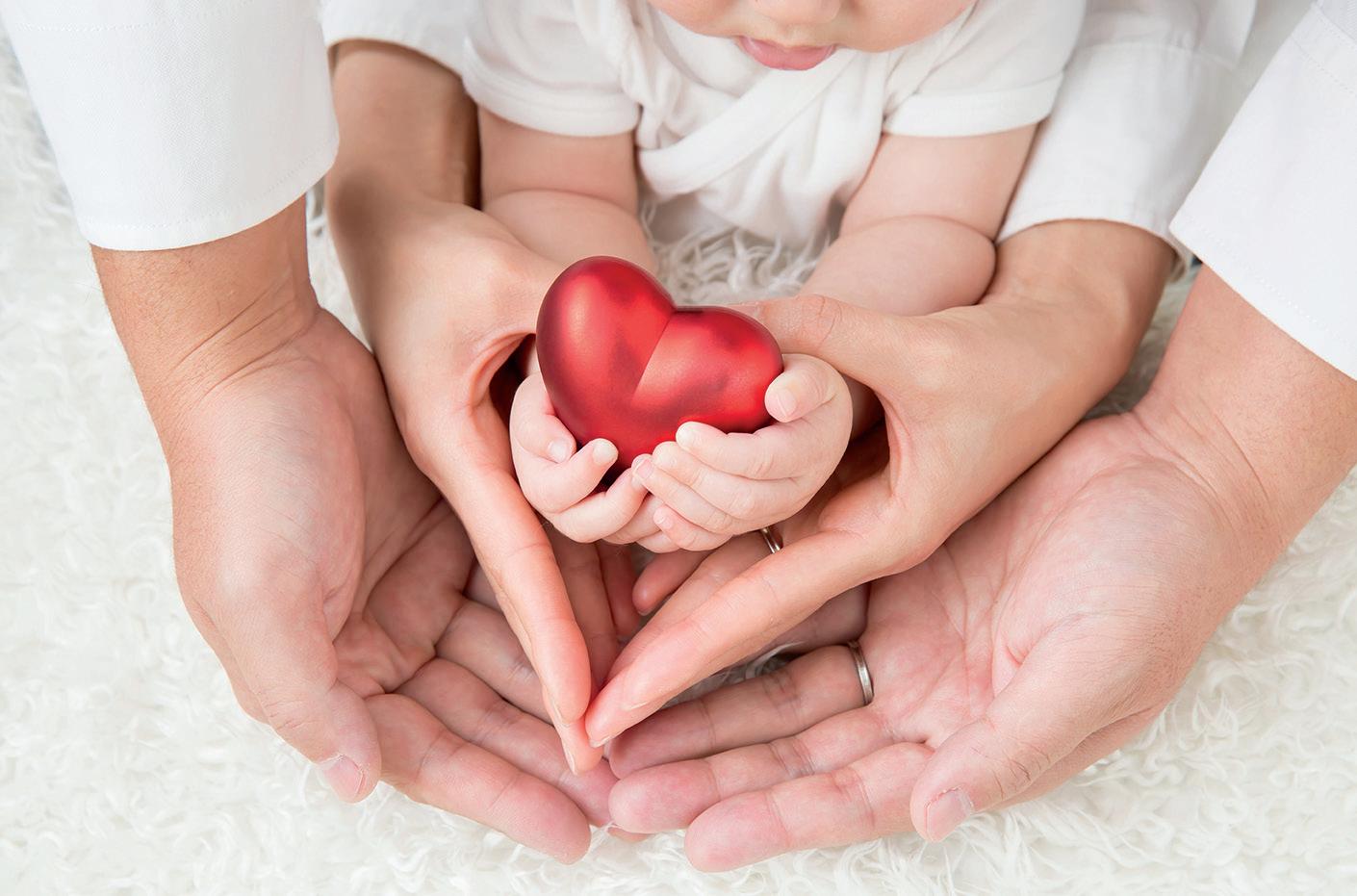
Samueli School engineers have designed a novel artificial intelligence method for assessing echocardiograms that could help cardiologists more accurately determine heart function and disease.
The machine learning platform will automatically segment the heart’s four chambers and calculate their volumes, eliminating the variability in human assessment of cardiac function, using echocardiography data. The research, led Dr. Arash Kheradvar, professor of biomedical engineering, and Hamid Jafarkhani, professor of electrical engineering and computer science, was published in the Journal of the Royal Society Interface.
Kheradvar explains that if 10 cardiologists independently examine an echocardiogram, they will calculate different numbers for the heart chambers’ volume. This may not be very important if the subject’s information is within a normal range; however, if the volume ranges are borderline, this variability can significantly change the course of treatment. Just a couple of percentage points in ejection fraction of a heart chamber could make a difference in the way a patient would be treated, if the number is marginal.
“There have been previous attempts with learning-based medical image analysis, but a major issue in applying artificial intelligence platforms to automatic segmentation of echocardiograms for clinical use is the lack of generalizability,” said Kheradvar.
For example, one can design a very accurate AI algorithm for a dataset from a hospital or a specific group of patients, but if the model is then used on another dataset, it may not perform well. “A generalizable model is one that can perform well on different datasets, not just the one used in training of the model,” Kheradvar said.
Unlike the standard automated approaches, the UCI researchers’ method is based on learning several levels of representations, corresponding to a hierarchy of features, and not speculating on any model or assumption about the image or heart chambers.
“To verify our method’s generalizability in comparison with other existing techniques, we compared its performance with the state-of-the-art method on our dataset in addition to an independent dataset of 450 patients,” said Jafarkhani.
The researchers successfully demonstrated the feasibility and performance of their method through computing validation metrics with respect to the gold standard, that of an expert cardiologist.