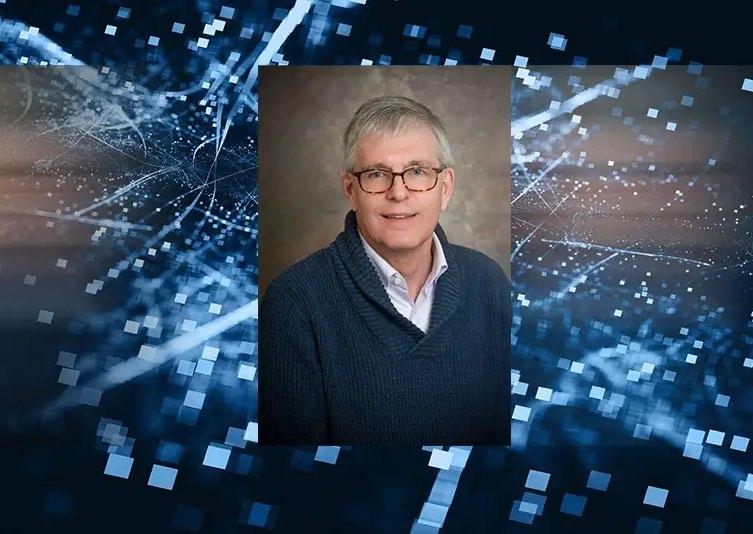
13 minute read
Research
HARNESSING ARTIFICIAL INTELLIGENCE
UD Prof. Rudolf Eigenmann is part of $20 million NSF Cyberinfrastructure Project
It takes real intelligence and plenty of collaborative muscle to harness the potential of artificial intelligence. Most of us can barely grasp the concept of human-made machines learning how to process and analyze enormous amounts of data, then using that mass of information to understand things at new scales and in new combinations, delivering useful insights that our brains would never be able to produce on their own.
Now University of Delaware Prof. Rudolf Eigenmann, interim chair of the Department of Computer and Information Sciences and professor of electrical and computer engineering, is playing a critical role in a new $20 million National Science Foundation-supported project designed to expand access to artificial intelligence.
AI for the masses, you might call it.
The project, called the NSF AI Institute for Intelligent Cyberinfrastructure with Computational Learning in the Environment (ICICLE), is one of 11 new National Artificial Intelligence Research Institutes the NSF announced recently. It is the second year of such investment by NSF. This $220 million in support follows the $140 million that created the first seven AI institutes in 2020. The overall effort now includes partners in 38 states.
“These institutes are hubs for academia, industry and government to accelerate discovery and innovation in AI,” NSF Director Sethuraman Panchanathan said when the new institutes were announced. “They lead to new capabilities that improve our lives from medicine to entertainment to transportation and cybersecurity, while growing the economy and maintaining global competitiveness.”
The goal is to provide this power tool — artificial intelligence — to researchers and many others who would not otherwise have access to the speed, efficiency, insight and accuracy it can provide. NSF also sees the potential to help older adults lead more independent lives and improve the quality of their care; transform AI into a more accessible “plug-andplay” technology; create solutions to improve agriculture and food supply chains; enhance adult online learning by introducing AI as a foundational element; and support underrepresented students from elementary school to postdoctoral levels to improve equity and representation in AI research.
The work is led by NSF in partnership with the U.S. Department of Agriculture National Institute of Food and Agriculture (USDA-NIFA), U.S. Department of Homeland Security (DHS), Google, Amazon, Intel and Accenture.
The institute Eigenmann is part of is led by Ohio State University. His focus will be on cyberinfrastructure, which includes hardware from supercomputers to tiny data-collection devices, the software that drives that hardware, the networks that carry the data and the applications that produce and consume that data — the physics problems, the biology problems, the chemistry problems, for example.
Eigenmann knows his way around all these things. He was an NSF program manager in the Office of Advanced Cyberinfrastructure before joining UD’s faculty in 2017.
“Data is a big word and there is such an enormous amount of data,” he said. “We need to be careful in managing and harnessing the data. That is another big question or element of cyberinfrastructure.”
The principal investigator is Dhabaleswar K. (DK) Panda, professor of computer science and engineering at Ohio State. Eigenmann is one of 46 researchers participating from 13 universities.
“Industry partners will play an important role in what we do for various reasons,” Eigenmann said. “What academics can do is dwarfed by what industry does, so we have to know what industry is doing and how we can synergize.”’
Developing AI capabilities across all colleges is a growing thrust at UD, Eigenmann said. Several students will be working on this new project, he said, and he anticipates involvement by other colleagues.
“When I arrived at UD, we started to build a faculty network in high-performance computing, with colleagues in all colleges involved,” he said. “Colleagues from all over the University participate in the Data Science Institute. It will take more, but this is a very good starting point.”
The ICICLE team will build and prove its system around three application domains: Smart Foodsheds (food produced locally), Digital Agriculture, and Animal Ecology.
In addition to UD and Ohio State, collaborators include: the Ohio Supercomputing Center, Case Western Reserve University, Iowa State University, Indiana University, the University of Wisconsin at Madison, the University of Texas at Austin/Texas Advanced Supercomputing Center, the University of Utah, UC San Diego/San Diego Supercomputing Center, UC Davis, Rensselaer Polytechnic Institute and IC-FOODS.
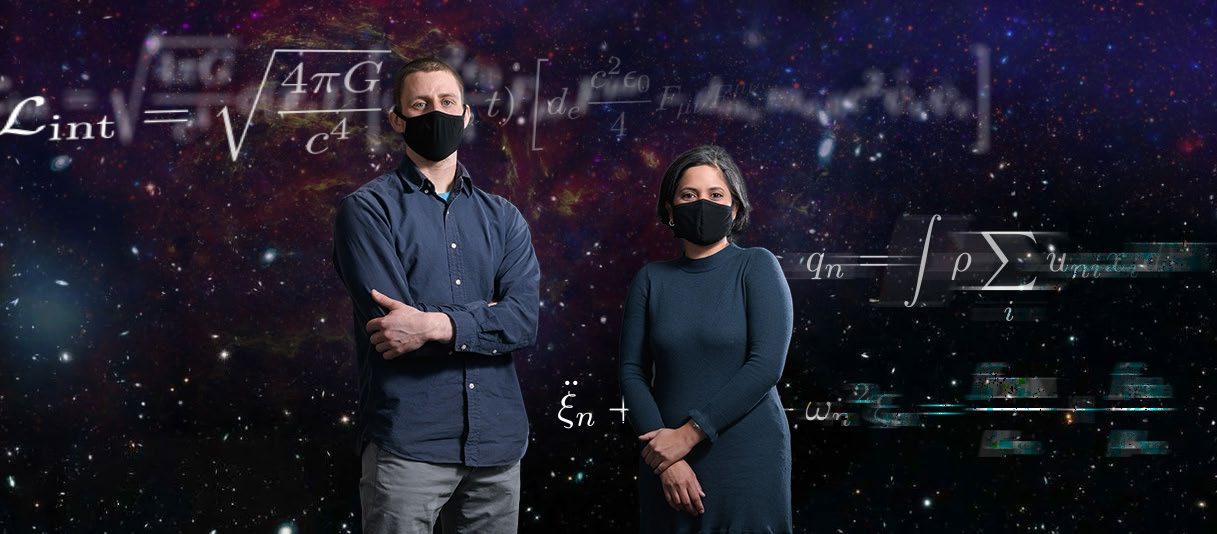
DARK MATTER DETECTION
UD’S SINGH AND COLLABORATORS PROPOSE REPURPOSING TABLETOP SENSORS TO SEARCH FOR DARK MATTER
Scientists are certain that dark matter exists. Yet, after more than 50 years of searching, they still have no direct evidence for the mysterious substance.
University of Delaware’s Swati Singh is among a small group of researchers across the dark matter community that have begun to wonder if they are looking for the right type of dark matter.
“What if dark matter is much lighter than what traditional particle physics experiments are looking for?” said Singh, an assistant professor of electrical and computer engineering at UD.
Now, Singh, Jack Manley, a UD doctoral student, and collaborators at the University of Arizona and Haverford College, have proposed a new way to look for the particles that might make up dark matter by repurposing existing tabletop sensor technology. The team recently reported their approach in a paper published in Physical Review Letters.
Co-authors on the paper include Dalziel Wilson, an assistant professor of optical sciences from Arizona, Mitul Dey Chowdhury, an Arizona doctoral student, and Daniel Grin, an assistant professor of physics at Haverford College.
NO ORDINARY MATTER
Singh explained that if you add up all the things that emit light, such as stars, planets and interstellar gas, it only accounts for about 15% of the matter in the Universe. The other 85% is known as dark matter. It doesn’t emit light, but researchers know it exists by its gravitational effects. They also know it isn’t ordinary matter, such as gas, dust, stars, planets and us.
“It could be made up of black holes, or it could be made up of something trillions of times smaller than an electron, known as ultralight dark matter” said Singh, a quantum theorist known for her pioneering efforts to push forward mechanical dark matter detection.
One possibility is that dark matter is made up of dark photons, a type of dark matter that would exert a weak oscillating force on normal matter, causing a particle to move back and forth. However, since dark matter is everywhere, it exerts that force on everything, making it hard to measure this movement.
Singh and her collaborators said they think they can overcome this obstacle by using optomechanical accelerometers as sensors to detect and amplify this oscillation.
“If the force is material dependent, by using two objects composed of different materials the amount that they are forced will be different, meaning that you would be able to measure that difference in acceleration between the two materials,” said Manley, the paper’s lead author.
Wilson, a quantum experimentalist and one of the UD team’s collaborators, likened an optomechanical accelerometer to a miniature tuning fork. “It’s a vibrating device which, due to its small size, is very sensitive to perturbations from the environment,” he said.
Now, the researchers have proposed an experiment using a membrane made of silicon nitride and a fixed beryllium mirror to bounce light between the two surfaces. If the distance between the two materials changes, the researchers would know from the reflected light that dark photons were present because the silicon nitride and beryllium have different material properties.
Collaboration was a key part of developing the experiment’s design, according to Manley. He and Singh (theorists) worked with Wilson and Dey Chowdhury (experimentalists) on the theoretical calculations that went into the detailed blueprint for building their proposed tabletop accelerometer sensor. Meanwhile, Grin, a cosmologist, helped shed light on the particle physics aspects of ultralight dark matter, such as why it would be ultralight, why it might couple to materials differently and how it might be produced. As a theorist, Manley said the opportunity to learn more about how devices work and how experimentalists build things to prove the theories that he and Singh develop has deepened his expertise while simultaneously widening his exposure to possible career paths.
A GROWING BODY OF WORK
Importantly, this latest work builds on previously published research by the collaborating teams, reported last summer in Physical Review Letters. The paper, which included contributions from former UD graduate student Russell Stump, showed that several existing and near-term laboratory-scale devices are sensitive enough to detect, or rule out, possible particles that could be ultralight dark matter.
The research reported that certain types of ultralight dark matter would connect, or couple, with normal matter in a way that would cause a periodic change in the size of atoms. While small fluctuations in the size of a single atom may be difficult to notice, the effect is amplified in an object composed of many atoms, and further amplification can be achieved if that object is an acoustic resonator. The collaboration evaluated the performance of several resonators made of diverse materials ranging from superfluid helium to single-crystalline sapphire, and found these sensors can be used to detect that dark matter-induced strain signal.
Both projects were supported in part through Singh’s funding from the National Science Foundation to explore emerging ideas around using state-of-the-art quantum devices to detect astrophysical phenomena with tabletop technologies that are smaller and less expensive than other methods.
Together, Singh said, these papers extend the body of work on what is known about possible ways to detect dark matter and suggest the possibility of a new generation of table-top experiments.
Singh and Manley are working with other experimental groups, too, to develop additional tabletop sensors to look for such dark matter or other weak astrophysical signals. They also are actively cultivating broader discussions on this topic within the dark matter and quantum sensors communities.
For example, Singh recently discussed transformational instrumentation advances in particle physics detectors at a virtual workshop organized by the Department of Energy’s Coordinating Panel for Advanced Detectors (CPAD). She also presented these results at a special workshop during the American Physical Society’s April meeting.
“It’s an exciting time, and I am learning a lot from the questions posed by scientists from diverse backgrounds at such workshops,” said Singh. “But it’s worth noting that my most original research ideas still come out of questions posed by curious students.”

University of Delaware Professors Marianna Safronova and Rudolf Eigenmann combine physics and computer science to develop their atomic portal.
new atomic data portal
UD’S SAFRONOVA, EIGENMANN AND COLLABORATORS SHARE THE POWER OF PRECISION ATOMIC PHYSICS
Even if you’re one of the most precise physicists on the planet — as University of Delaware Professor Marianna Safronova is — you still will need collaborators whose skills complement your own and make new opportunities possible.
You will need someone such as UD Professor Rudolf Eigenmann, who can take that precision, add generous amounts of computer science expertise and help to make that high-value information available to any other physicist who wants it.
A project led by Safronova and Eigenmann and supported by the National Science Foundation has done just that, producing a Portal for High-Precision Atomic Data and Computation that provides extraordinary information about atomic properties in user-friendly ways.
It’s the periodic table on steroids and it is already drawing keen interest from researchers who need to know the nitty-gritty details of the materials they work with.
“The properties are key inputs for many research projects, such as quantum simulation, development of quantum sensors, tests of fundamental physics and many others,” Eigenmann said. “Atomic data are important for astrophysicists and plasma physics applications and may help to shed light on how heavy elements such as gold and uranium are produced in the universe.”
Bindiya Arora, a UD alum who now is a physics professor at Guru Nanak Dev University (GNDU) in Punjab, India, worked on the fundamental physics and modeling with Safronova. Eigenmann, computer engineering graduate student Parinaz Barakhshan and physics graduate student Adam Marrs developed the computer mechanisms and interfaces that put the calculations to work.
Knowing the precise atoms in materials is of critical importance in engineering characteristics and science projects. Now a growing body of high-precision data is available online, giving the research community a new power tool for a broad range of applications in physics and engineering.
Safronova’s calculations already have contributed to many such advances, including the development and fundamental research with optical atomic and nuclear clocks, tests of fundamental symmetries, studies of dark matter and other projects that are pushing past standard particle physics models.
“Our group and collaborators have developed high-precision code over the past 20 years,” Safronova. “This portal makes them searchable and easy to access. In many cases, those properties have not been measured before. This data is not provided by any other database.”
The new portal provides access to calculations of properties of atoms and ions, such as matrix elements, transition rates, lifetimes, polarizabilities, hyperfine constants and others.
The computer science challenge included finding efficient ways to link Safronova’s complex computations with an online portal that could automatically include new data.
The first version of the portal included data on 12 elements. Already, scientists and engineers have asked for more. Safronova said the hope is to add 30 more elements in the next year.
The information can be used for fundamental physics, Safronova said, precision measurements, quantum simulation, atomic clocks and astrophysics, to name just a few applications.
“You may wonder why we can provide this information at the University of Delaware and others can’t,” Eigenmann said. “The previous work of Marianna and her collaborators has created these computational techniques that produce precision no one else has.”
The portal is open to all and free to use. And, Safronova said, it would not exist without the help of Eigenmann and his team.
“I had no idea how to get that data online,” she said. “I know how to produce the data and I know how to publish the numbers. But to make a website and release actual codes — I had no idea how to do that. Collaborating with computer scientists taught me so much about how I should write code. We redesigned a lot of codes to make more of the software.”
Marrs said the project has been a good challenge for him, too.
“The collaboration forced my brain to go in two different ways,” he said. “It was very much a learning process. High precision numbers I’m familiar with. But doing it in this way, where we want it to be seen by everyone, motivated me to make the whole process as automatic as possible.”
Arora said she knew from previous studies that Safronova knew how to produce precision code. But Arora had never worked on converting those codes for open access.
“Compiling data from various experiments is part of my job, and this gave me insight to new findings,” she said. “All the complicated codes and software were available, but they were very hard to use. The kind of open access we are trying to provide is easy to use. You just need to know what kind of atom you want and what property you want to work on.” Safronova said the work was done using UD’s high-performance Caviness and Darwin clusters.
“This code requires high-performance computing,” she said.
That means far more muscle than an ordinary computer can muster. Darwin has 6,000 cores available — the approximate capacity of 6,000 computers. Safronova’s team uses more than 500 cores.
And that powerful computation now is available to those who may not have HPC skills or access. The portal is webbased, available to anyone with an Internet connection. Eigenmann said it could one day be accessible with a mobile app.
That could put the power of the Darwin computations into many a pocket.
“This collaboration has been rewarding on all sides,” he said. “I enjoy learning physics and some of it takes me back to high school and the periodic table. What we do in computer science — I find it rewarding when we see the application in physics, biology or chemistry. There are interesting computer science issues there.”