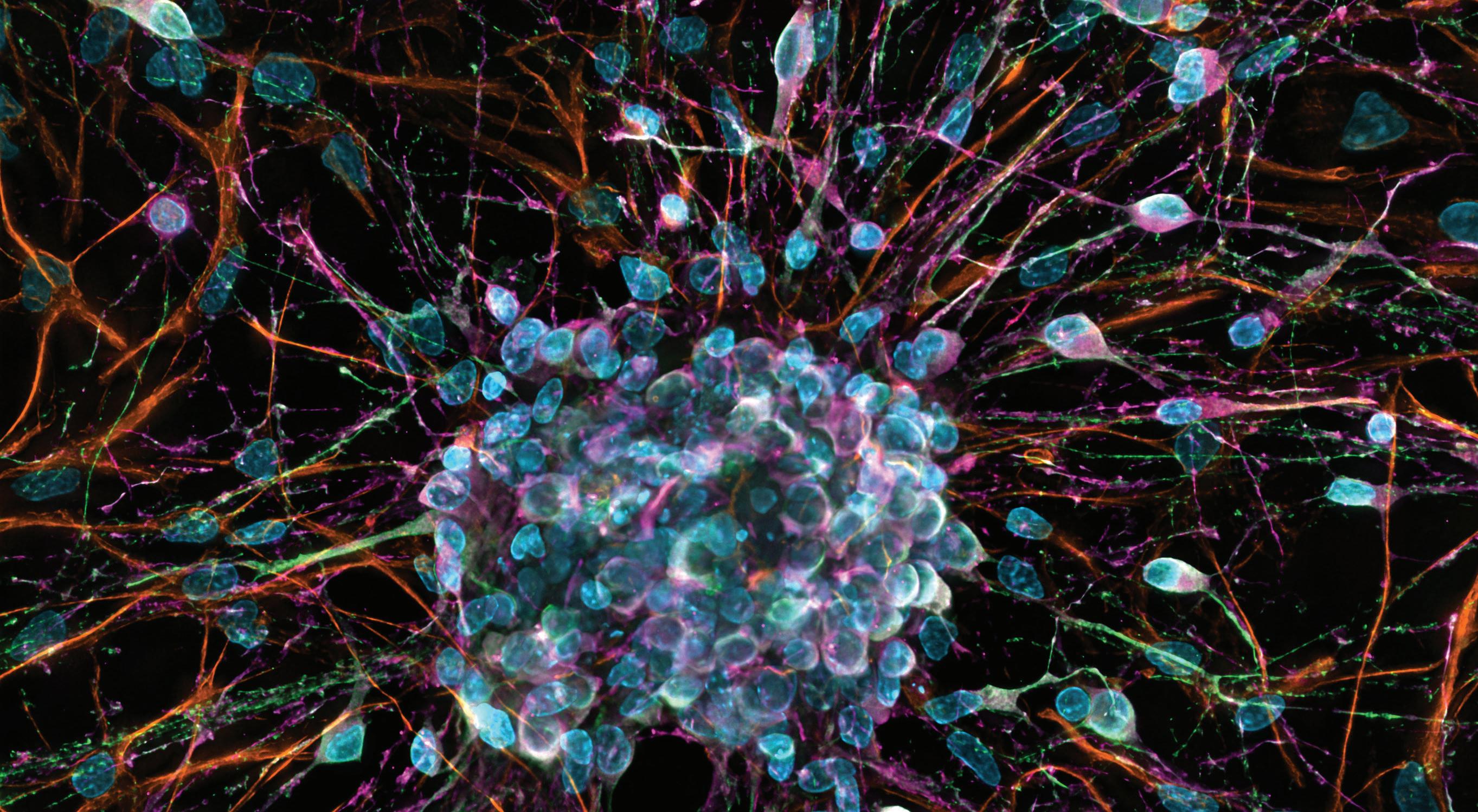
6 minute read
Sneha Makhijani
Using Light to Control the Brain
By Lasya Kambhampati
Advertisement
Image ZEISS Microscopy. [CC BY-2.0]
Imagine a pendulum swinging back and forth on a string. Your job is to poke it in just the right way so that it stops moving. However, you are given no other information about the movement of the pendulum. You would end up just poking around in the dark, hoping that somehow you will be able to nudge in just the right direction with the right amount of force. This is the analogy that Dr. Andrea Giovannucci, an assistant professor in the Joint UNC-NCSU Department of Biomedical Engineering, uses to describe the current field of neuro-engineering. Scientists are sending signals to neurons (brain cells) hoping that one will eventually cause the reaction they are investigating. At the moment, a prominent approach for artificially connecting with the brain is through the implantation of a chip, such as the NeuraLink technology created by Elon Musk. This chip can then be used to get input from sensory neurons (“read”) and send output to nerves (“write”). However, there are multiple issues with this idea – the implanted needles would
Dr. Andrea Giovannucci corrode, and the chip cannot be maintained for long periods. Furthermore, it is difficult to precisely modulate neurons to achieve the desired result. Chips do have positive aspects; for example, the signals to and from the chip can be decoded in real time, which is essential for effective prosthetics. Dr. Giovannucci’s research aims to turn on the light so that we can accurately and precisely poke specific neurons to elicit a particular reaction. He became interested in this particular topic when he realized that by combining his previous experience with algorithms, artificial intelligence (AI), and prosthetics, he could develop algorithms that would control neuroprosthetics. Instead of using a chip to control neural signals, he elected to use light (holography) to stimulate neurons. Although it is important to mention that, currently the technology is unable to read and modulate brain activity within 30 milliseconds, which is essential to have an effective dialog between input and output. Dr. Giovannucci describes the current relay time as “asking you a question and getting a response 20 minutes later.”1 To do this, two important changes need to be made to the brain’s physiology. Using a genome changing mechanism, the brain can express a gene that encodes a fluorescent protein (Figure 1). This protein activates when the neuron fires, signaling neuronal activity and allowing scientists to “read” the brain’s current state. Past literature has shown that this is possible and effective in the brains of mice and primates. In addition, there needs to be a gene that allows light to change the activity of the neuron. This allows researchers to shine a particular wavelength of light on a neuron and either activate it or suppress its activity. After completing these two Figure 1. Neurons labeled with modifications, an optical- fluorescent proteins

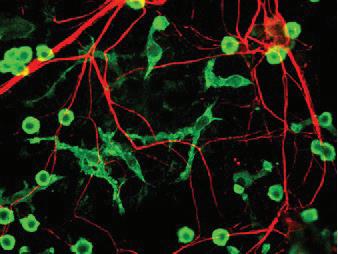
based neural prosthesis can be controlled through light firing on to the brain. However, this technology is years away from becoming a reality; we have the infrastructure but not the computational ability. Furthermore, we need more data on exactly which neurons to target to neurons to target to elicit particular motions, which is the focus of Dr. Giovannucci’s current experiments. He is working on a way of getting a readout of the brain’s current state and integrating algorithms that can modulate the firing or suppression of neurons in collaboration with Dr. Nicolas Pegard. Dr. Pegards’ algorithms use Deep learning – a type of machine learning based on neural networks – to analyze inputs and produce outputs. For example, if you were to input a starting “picture” of the brain at a certain point in time and a state of the brain that you want to reproduce, the algorithm would be able to output a pattern of light (hologram) that would target specific neurons that need to be activated or inactivated. There are two experiments that Dr. Giovannucci would like to carry out using the above technology. The first would be to estimate the connectivity of a specific subset of neurons in areas of the brain related to motor function. Imagine you have a series of pendulums all swinging at different speeds but close enough that changing the motion of one of the pendulums will affect the others. If you only wanted to stop the motion of one of the pendulums, you would need to know how each pendulum is connected to the others so that you could poke it in exactly the right direction and with the correct force to achieve your goal. Researchers face a similar conundrum when it comes to modulating the synaptic activity of the neurons they are targeting. Neurons are all part of a network – changing the activity of only one neuron could affect the behavior of all the neurons that they are connected to. To provide data on the connectivity between the neurons, Dr. Giovannucci will read the status of the brain, modulate specific sets of neurons, measure the response in the surrounding neurons, and then use this data to estimate connectivity. Once he has gathered this data on connectivity, Dr. Giovannucci also plans to try to stimulate neurons so that mice can repeatedly perform skilled movements. Past attempts at this have failed, but Dr. Giovannucci believes it was due to the lack of precise activation and suppression of neurons. Therefore, the results were unreliable, unskilled movements. With the new information and algorithm, the results should be reproducible. To conduct this experiment, the researchers place a panel of clear glass on top of the brain of the mice and use light to stimulate neurons in the motor cortex (an area of the brain necessary for voluntary movement).3,2 See Figures 2 and 3 for more information. Dr. Giovannucci is also planning for the future. He hopes
Figure 2. Light is used to stimulate neurons to create a system that can estimate the internal dynamics
Figure 3. A microscope is used to examine a mouse brain which has been labeled with a fluorescent protein
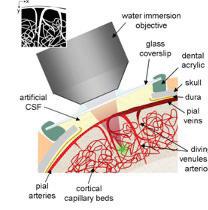
of the brain in terms of a specific set of variables – similar to equations used in physics to determine the movement of particles. With the combination of all of these experiments, there is a possibility of real-life applications, but there are still some obstacles that need to be overcome. Primarily, there are ethical and viability issues with using technology to implant new genetic material into the neurons of human patients along with health issues since these technologies can drastically reduce life span. Furthermore, light can only penetrate a short depth into the brain so it can only regulate the activity of surface-level neurons. Regardless, the therapeutic uses of the technology are boundless. There is literature that shows that seizures can be stopped by modulating activity in the cerebellum, which coordinates a variety of functions including motor activity, perception, and balance.2,4 Optogenetics, the science of using light to control neurons, could also be used to solve “underlying pathological states and recover cognitive functions.”1 One day, amputees could even control their prosthetics seamlessly, as if it were truly a part of their own body.
References
1. Interview with Andrea Giovannucci, Ph.D. 9/23/20. 2. Wnuk A.; Davis A.; Parks C.; et al. Movement. In Brain
Facts, Edition 8; Society for Neuroscience: Washington
DC, 2018; pp 26-32. 3. Heo C.; Park H.; Kim Y.; et al. Nature. 2016, 6. 4. Krook-Magnuson E.; Szabo G.; Armstrong C.; et al. eNeuro. 2014.