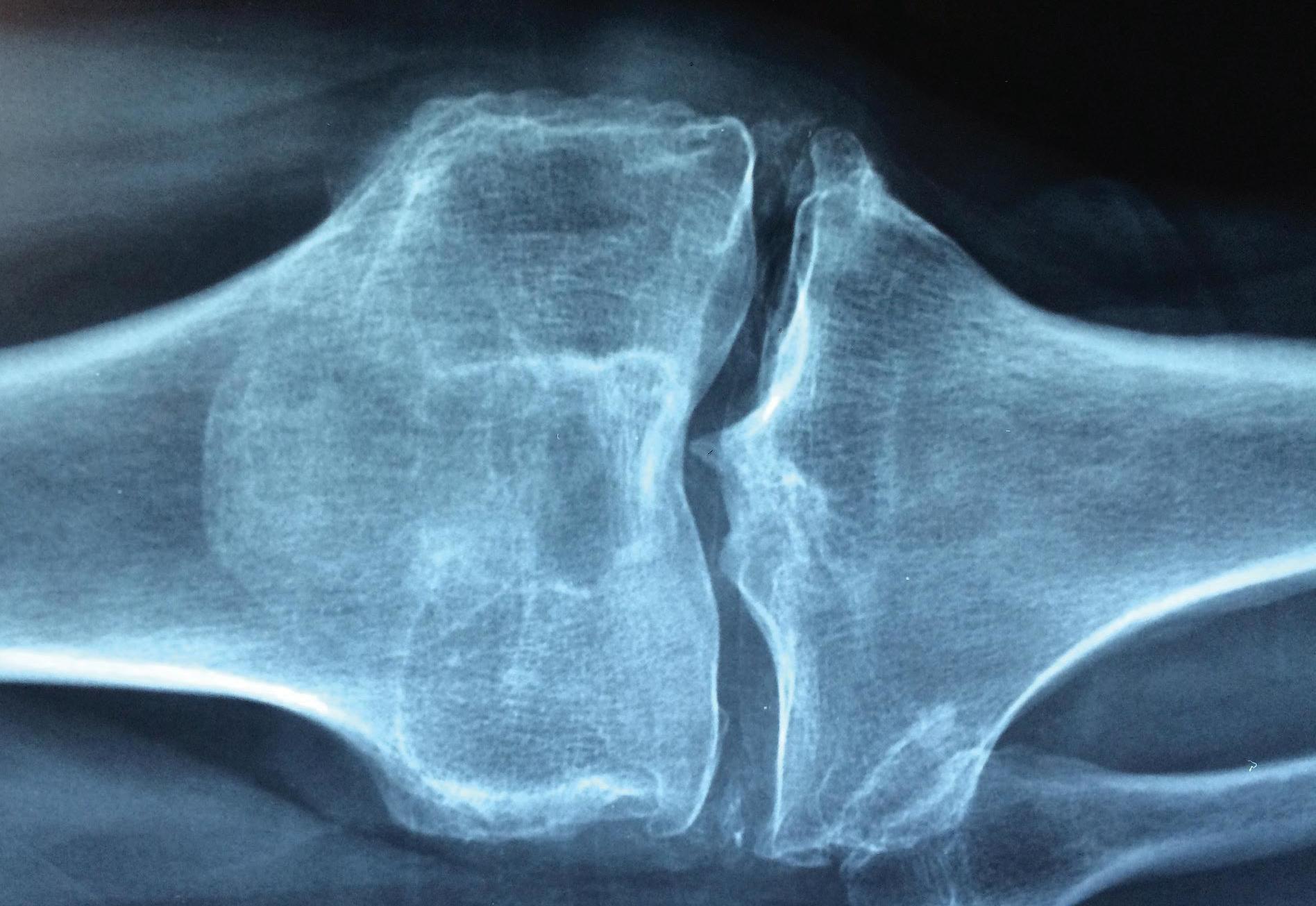
11 minute read
Hannah Koceja
By Henry Bryant
Advertisement
Image by Manuel González Reyes .[CC0]
Although rheumatoid arthritis may be more recognizable to many, osteoarthritis actually affects more people; it is the leading cause of pain and disability in older adults.1 Rheumatoid arthritis is an autoimmune disease, meaning the body’s immune system mistakenly tries to destroy healthy tissue. For rheumatoid arthritis, this includes cells in joint tissues. Osteoarthritis, on the other hand, is commonly misidentified as the “wear and tear” arthritis due to the disease’s prevalence in older individuals, especially after joint injury.2 However, recent research has uncovered complex, intertwined molecular signaling pathways and feedback loops involved in osteoarthritis, changing the medical community’s perception of the disease entirely. These pathways, rather than strictly the mechanical erosion of joint tissues, cause the joint pain and disability associated with the disease. Dr. Richard Loeser, an Eminent Professor of Medicine in the Division of Rheumatology, Allergy, & Immunology at UNC-Chapel Hill and Director of the Thurston Arthritis Research Center, has played a central role in this shift. Dr. Loeser’s lab uses a combination of human joint tissue and mouse models to study osteoarthritis. Joint tissue taken from both healthy young patients and older patients with osteoarthritis facilitate the discovery of physiological processes and potential biomarkers of the disease. Researchers in the Loeser Lab also activate cellsignaling molecules in healthy tissues in an attempt to study relevant pathways when osteoarthritis is induced. Both techniques highlight molecules and pathways of interest that present starting points for further investigation.
The researchers then explore the complex interactions of these signaling pathways in mouse models. Mouse models provide a more realistic picture of what happens inside the human body that tissue samples alone cannot emulate, and many mouse pathways mirror pathways found in the human body. Additionally, an array of biochemical techniques elucidate upstream and downstream molecules in the signaling pathways for a more complete biological picture in mice. One of these techniques involves knocking out specific genes in a pathway to see the effects on other molecules downstream. Together, these studies led Loeser and his lab to discover a specific positive feedback loop that contributes to osteoarthritis. First, mechanical trauma, over-
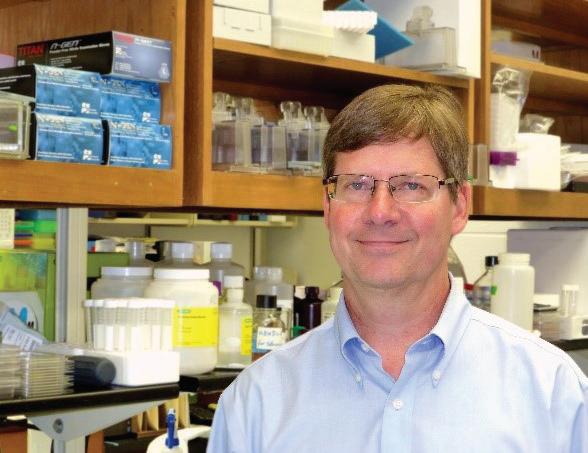
use, and other factors partially destroy the extracellular matrix (ECM) that makes up the majority of a patient’s joint cartilage. The ECM normally consists of proteins and other biomolecules outside of individual cells, giving the joints their strength and providing low-friction surfaces for bones to glide over. However, as fragments of cartilage break off they bind to receptors on the surface of joint cells, causing inflammation and the release of enzymes that break down the ECM further.3 These effects perpetuate the feedback loop; as more of the ECM breaks
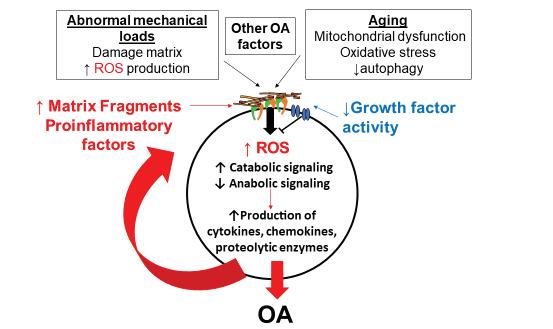
down, more fragments bind to cell receptors, amplifying the damage that exacerbates osteoarthritis. Dr. Loeser’s lab also studies the potential role of reactive oxygen species and the gut microbiome on signaling pathways related to osteoarthritis. Both reactive oxygen species and the gut microbiome induce inflammation, which may contribute to the release of enzymes that destroy the ECM and initiates the ECM destruction feedback loop.⁴,⁵ While many questions surrounding the role of joint components in disease progression and the degree of interdependence between osteoarthritic signaling pathways remain, the Loeser Lab’s work has reshaped how scientists and healthcare providers approach and understand the disease. Dr. Loeser hopes that increased knowledge of the disease can aid researchers to develop effective treatments for osteoarthritis by targeting one of the many molecules involved in the pathways central to the disease such as ROS. Dr. Loeser has recently started a drug discovery project based on his work that will help facilitate the development of new therapeutics.⁵ Dr. Loeser’s work to improve the lives of those suffering from arthritis extends beyond the lab; he also juggles many other roles serving as Director of the UNC Thurston Arthritis Research Center and treating patients in the clinic. Dr. Loeser’s clinical research builds upon the knowledge gained by his lab, seeking to treat patients that have knee osteoarthritis with exercise and weight loss. He also participates in a multidisciplinary project to define osteoarthritis phenotypes. In other words, patients with osteoarthritis may differ from one another and represent different subtypes of the disease. Dr. Loeser has not only made great strides in the advancement of osteoarthritis knowledge; he has also been a mentor for many undergraduates, graduate students, medical students, and post-doctoral fellows. He allows his students to pursue the research in this lab they find most interesting and helps guide the next generation of researchers by assisting them in creating their own projects. Dr. Loeser loves to share his knowledge with others; he describes it as “the fun part” of his work.⁵ By sharing his work with others, he has informed other researchers and clinicians who now better understand the signaling pathways of osteoarthritis and the role aging plays in the development of osteoarthritis. While Dr. Loeser’s goal is ultimately to help people with osteoarthritis live better lives, he also hopes to inspire young scientists to continue his legacy.
Figure 2. A simplified representation of the positive feedback loop discovered by Dr. Loeser’s lab that contributes to osteoarthritis.
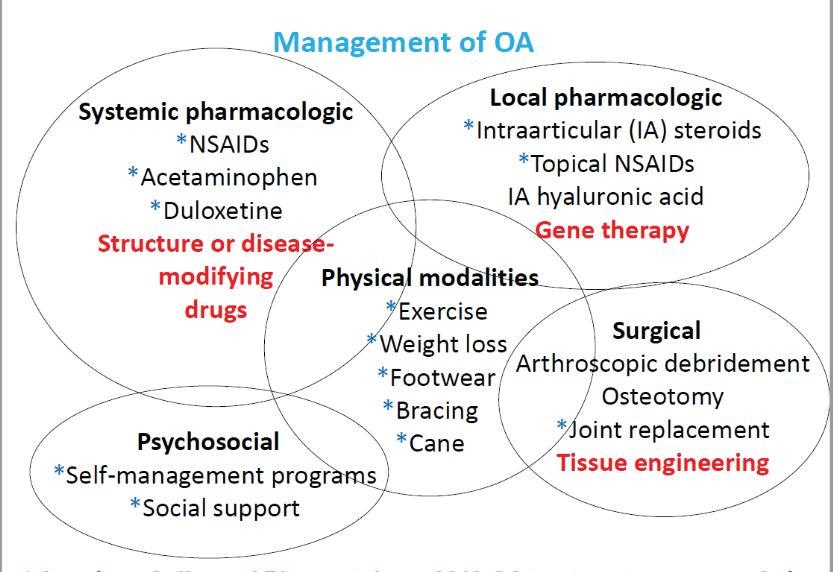
Figure 3. Current and future management and treatment options for osteoarthritis. Items with blue stars are recommended treatment options by the American College of Rheumatology (2019). Red items are being developed and do not currently exist.
References
1. Loeser, Richard F., et al. “Osteoarthritis: A Disease of the
Joint as an Organ.” Arthritis & Rheumatism, vol. 64, no. 6, 2012, pp. 1697–1707., doi:10.1002/art.34453. 2. Katz, Jeffrey N., et al. “Diagnosis and Treatment of Hip and Knee Osteoarthritis.” JAMA, vol. 325, no. 6, 2021, p. 568., doi:10.1001/jama.2020.22171. 3. Loeser, Richard F. “Integrins and Chondrocyte–Matrix
Interactions in Articular Cartilage.” Matrix Biology, vol. 39, 2014, pp. 11–16., doi:10.1016/j.matbio.2014.08.007. 4. Coryell, Philip R., et al. “Mechanisms and Therapeutic
Implications of Cellular Senescence in Osteoarthritis.” Nature Reviews Rheumatology, vol. 17, no. 1, 2020, pp. 47–57., doi:10.1038/s41584-020-00533-7. 5. Interview with Richard F. Loeser, Jr., MD. 02/18/21 17
Medicine & Health The Medical *Potential* of Electrochemical Detection
Improved glucose monitor holds greater implications
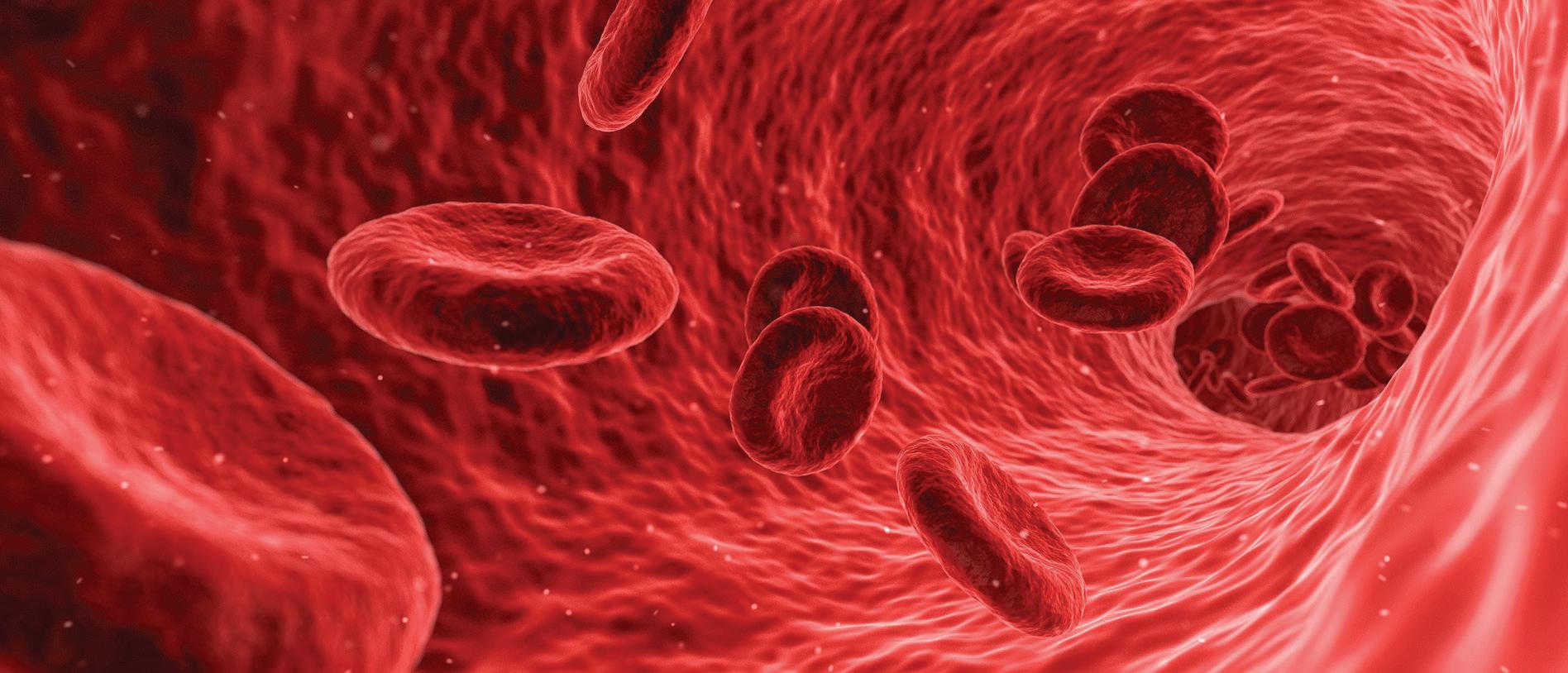
By Maia Vierengel
Image courtesy of Wikimedia Commons
It is estimated that one billion chemical reactions occur in a single cell of your body every second! Within such a complex system, it is extremely difficult to measure just one type of molecule at a time, but for people living with diabetes, it is essential for scientists to be able to measure on this micro-level quickly and accurately. Diabetes is a disease which affects roughly 10% of the U.S. population, causing glucose levels to fluctuate between lower or higher levels than normal.1 Glucose is the body’s most basic sugar, which cells use to make energy. If glucose levels are left unchecked, a diabetic can easily become hypoglycemic or hyperglycemic, both of which are seriously dangerous conditions. A healthy person’s blood glucose levels typically hover around 140 mg/dL. Hypoglycemia occurs when blood glucose levels dip below 70 mg/dL; this condition can become critical within 10-15 minutes, with the possibility of seizure or loss of consciousness. Conversely, hyperglycemia occurs when blood glucose levels are elevated above 180 mg/dL, which rapidly causes nausea or shortness of breath.2 Thus, it is imperative that diabetics continually monitor their blood glucose levels. Most commonly used glucose monitors are electrochemical, as they are inexpensive, rapid, portable, and user-friendly.3 However, current commercial monitors must be replaced regularly as biofouling causes them to degrade over time. Biofouling happens when biomolecules such as proteins and cytokines stick to and cover the electrode surface, making it less sensitive and less reliable. Additionally, modern monitors use amperometry or voltammetry which use high currents to make measurements and thus are more disruptive to living cells.3 Enter Jeffrey E. Dick, PhD, and his graduate student, Nicole L. Walker. These two UNC scientists specialize in nanoelectrochemistry and single-cell biology, and set out to combine the two fields in order to make great strides in biosensor technology. Nanoelectrochemistry investigates electrical properties of materials on the nanoscale level, while single-cell biology studies genomics, proteomics, and metabolism within a single cell. In their recent publication, Dick and Walker outline a new type of glucose monitor: one that is reliable, versatile, specific, and miniturizable. The glucose sensor, an oxidase-loaded hydrogel potentiometer, utilizes oxidase enzymes and a chitosan hydrogel measure electric potential instead of amperage or voltage.3 Electric potential is the amount of work needed to move a unit of electric charge, whereas voltage refers to a difference in potential and amperage refers to an amount of current. The enzymes are embedded in the selfassembling gel, which is laid over the electrode surface as shown in Figure 3. When a glucose molecule comes in contact with one of the enzymes, it oxidizes to form glucanolacetone and hydrogen peroxide, or H₂O₂. When the peroxide reaches the electrode surface, it signals a change in potential to the monitor, measuring a change in glucose concentration. After experimentation, the lab proved that the detector is both highly selective and versatile. This means that the detector only signals when glucose is near the sensor and that in the future it could detect other metabolites important to medical technology. The latter part of Dr. Dick’s paper on
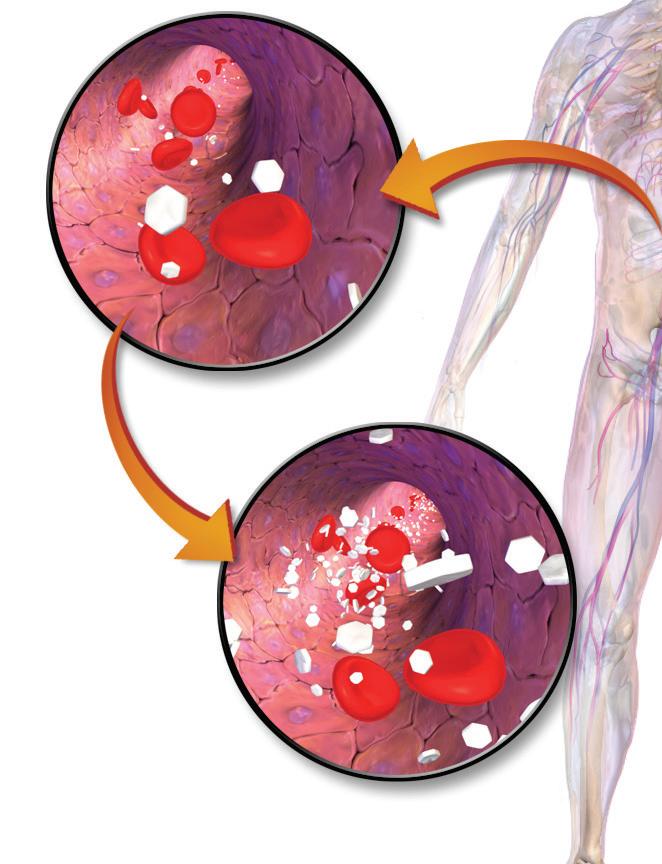
Figure 1. A depiction of glucose levels rising in the blood to cause hyperglycemia. Image courtesy of Blausen Medical Communications Figure 2. A diabetic using a commercial glucose monitor. Photo by David-i98.
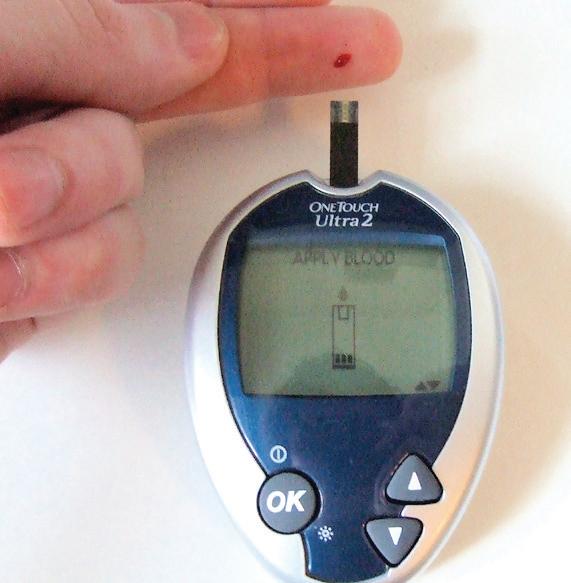
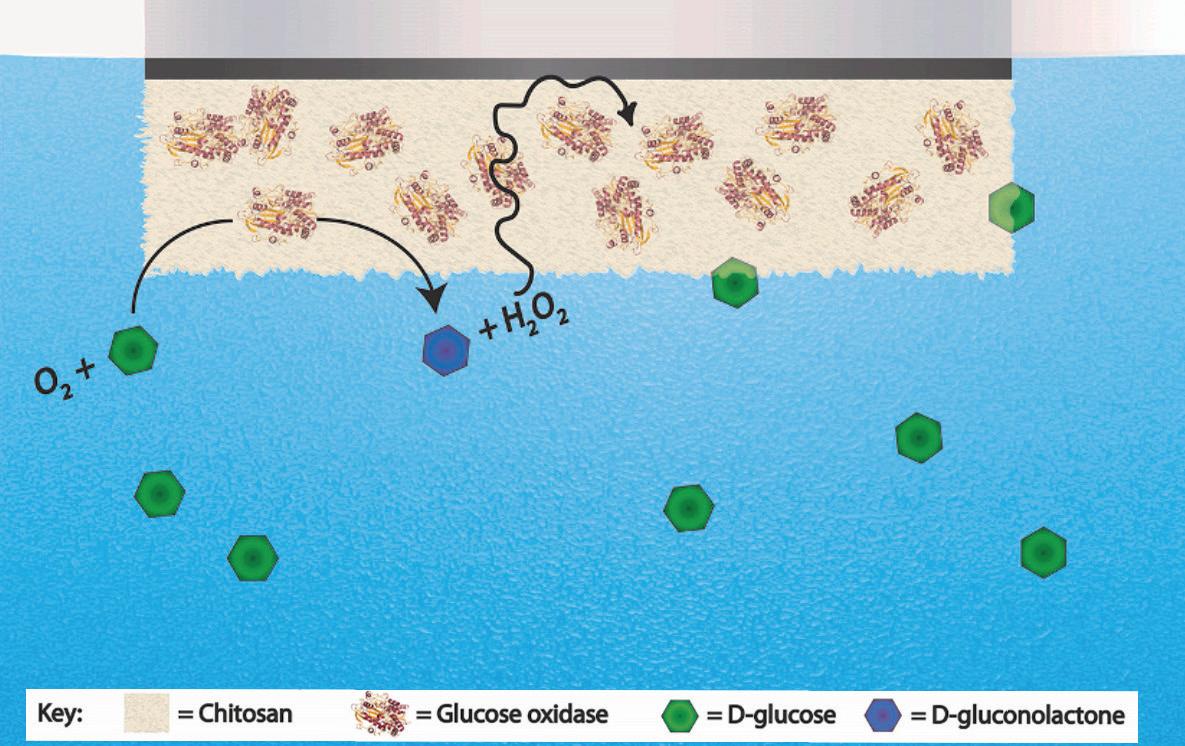
Figure 3. A depiction of the sensor design; on the electrode surface, the chitosan hydrogel keeps the oxidase enzymes in place, while allowing the glucose and hydrogen peroxide (H₂O₂) to move freely. Figure from article by N. L. Walker and J. E. Dick. oxidase-loaded hydrogel potentiometers demonstrated how the same system could be used with galactose, a different kind of sugar. The expansion of the detector to measure galactose levels may be applicable to monitoring individuals with galactosaemia, a genetic disorder that affects one’s ability to metabolize galactose. Furthermore, this potentiometer is miniturizable, meaning it can continue to yield accurate results on the nanoscale. Miniturizability is critical when considering the invasiveness of any kind of medical device. For diabetics, a glucose monitor such as Dr. Dick’s would mean a less-invasive device, faster results, and more accurate readings of glucose levels. Potentiometric biosensors are easy and inexpensive to create, which would make them most accessible to diabetics. Considering the other costs associated with diabetes, such as insulin which can be hundreds of dollars per month, a less expensive monitor could ease the financial burden. Beyond the scope of diabetes or galactosaemia, the monitor could be used specifically for a number of other metabolites; all that’s needed is an enzyme specific to that metabolite, that causes an oxidation-reduction reaction and causes a change in potential.
This device did not come to fruition easily; the team had to overcome more than a few hurdles to bring the idea to reality. One of their biggest challenges was Mixed Potential Theory, which Dr. Dick mentioned tends to be a problem when measuring within a complex organism.⁴ “You have to deal with Mixed Potential Theory, the fact that other things are going to contribute to the potential signal that you’re measuring, like oxygen reduction. Because of that, if you stick an electrode in a cell, you have lots of things that can happen, lots of reactive species that could affect that potential. So, we have to be able to mitigate that.” A single cell contains many chemical reactions, and the presence of oxidative species like oxygen (O₂) can complicate the potential signal. To resolve this problem, they tinkered with different materials for the electrode surface, and found that peroxide specifically reacts favorably on the surface of platinum, making it a great electrode material. When asked about the impact of his device and its research as it pertains to other fields, Dr. Jeffrey Dick insisted that single-cell detection is the future of disease medicine and will impact lots of other fields. “There are extensive heterogeneities between cells. Take the pancreas, for instance; it’s really difficult to diagnose pancreatic cancer because pancreatic cells are very heterogeneous in nature, and understanding how they interact with each other on a single-cell level could lead to that one cell that becomes pancreatic cancer. It’s really necessary to understand disease on the most fundamental level: the cell.” As with most other things in life, generalizations can cause very important details to be washed out! Concerning collaboration, Dick reported that the lab is working with UNC biomedical engineering professor Koji Sode, a world expert on glucose sensors and enzyme manipulation. Additionally, they have compacts with scientists at the UNC Medical school to reach their next goals: lowering limits of metabolite detection, enhancing sensitivity, and understanding how the detector can be applied to different diseases, including those within the worlds of oncology, virology, and stem cell differentiation. Dr. Dick’s research and his inventive ideas clearly show the broad implications of the intersection of electrochemical detection and single-cell biology. Though he has only just begun, his research and innovations could lead to a ripple effect in the lives of ordinary people in addition to the fields. of biochemistry and medicine.
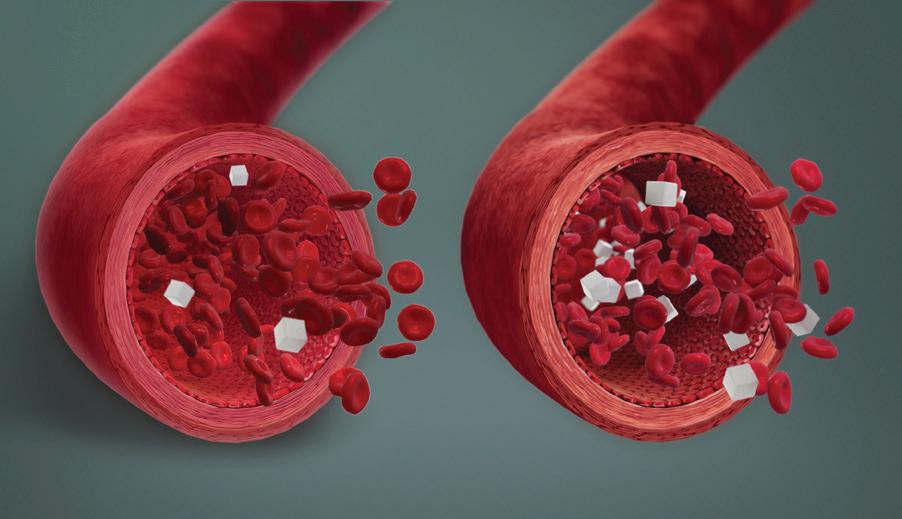
Figure 4. A depiction of low blood sugar levels during hypoglycemia (on the left) and normal blood sugar levels (on the right). Image courtesy of Scientific Animations. Jeffrey E. Dick, PhD
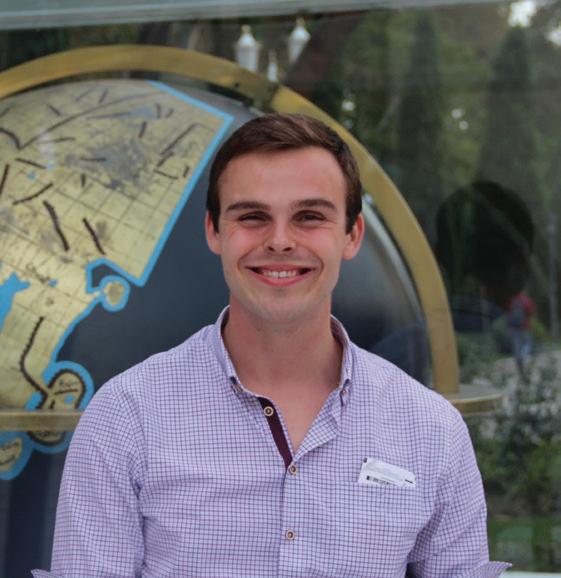
References
1. National Diabetes Statistics Report 2020. US Department of Health and Human Services 2020. 2. Hypoglycemia, Hyperglycemia: Symptoms and Causes. https:// www.mayoclinic.org/diseases-conditions/hypoglycemia/symptoms-causes/syc-20373685 (accessed March 1st, 2021) 3. Walker, N.L.; Dick, J.E. Elsevier: Biosensors and Bioelectronics 2021, 178. 4. Interview with Jeffrey E. Dick, Ph.D. 2/16/21