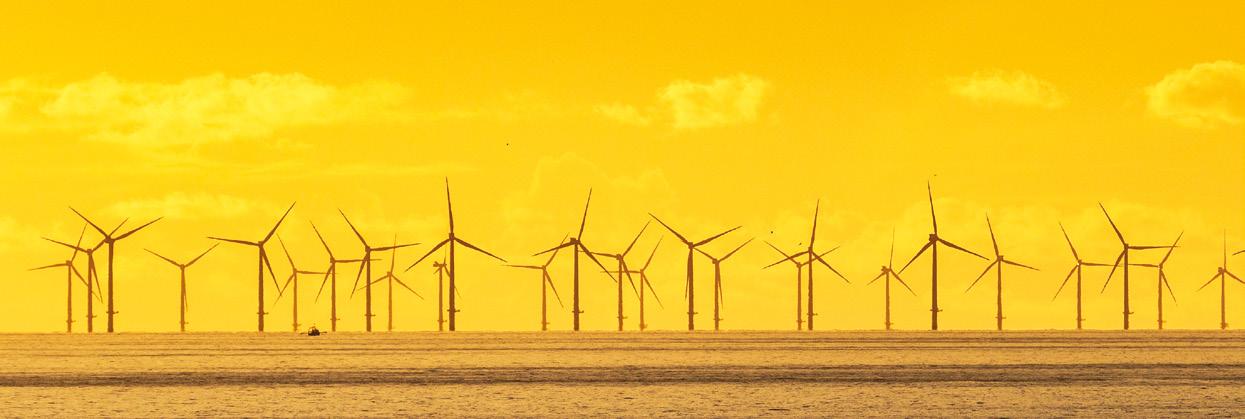
21 minute read
OFFSHORE ENERGY
The race against climate change relies on the energy transition, to create and sustain renewable energy sources, and offshore energy has a significant part to play. Researchers from the University of Southampton are forging ahead, developing integrated and informed solutions that aim to deliver on offshore energy targets.
The UN General Assembly’s Sustainable Development Goals (SDGs), include a dedicated and stand-alone goal on energy, SDG 7, calling to ‘ensure access to affordable, reliable, sustainable and modern energy for all’.
“SDG 7 calls for action in five key areas,” explained Susan Gourvenec, Professor of Offshore Geotechnical Engineering. “Closing the energy access gap, transitioning to decarbonised energy systems, mobilising adequate and predictable finance, leaving no one behind on the path to a netzero future and harnessing innovation, technology and data.”
The challenge to deliver the ambitions for offshore energy are immense. Global forecasts indicate the requirement for 2000 gigawatts (GW) of offshore wind capacity globally by 2050 to meet net-zero targets, a 35-fold-increase in installed capacity compared to current levels, requiring around 5000 new turbines to be installed each year between now and the mid-century.
Considering just the UK, an established leader in offshore wind capacity, around four times the current capacity needs to be installed in the next seven years to reach the Government targets of 50 GW by 2030; an installation rate of three to four times that achieved in the last few years and equivalent to the installation of one offshore wind turbine per day between now and the end of the decade.
Southampton capabilities
The University of Southampton has expertise across all SDG7 action areas, and in particular immense breadth and depth of capability in harnessing the innovation, technology and data required to deliver the offshore renewable energy needed to achieve decarbonisation targets.
“Our technical expertise ranges across engineering disciplines, from mapping the seabed and characterisation of seabed sediments, design of foundations, anchors and mooring systems, and working with the network of cables that are founded on or in the seabed,” said Susan. “We have engineering researchers working in hydrodynamics and fluid-structure interaction of fixed and floating offshore wind infrastructure, aero interactions of turbine blades with the atmosphere, composite blade manufacture and performance, corrosion, sensing, noise, battery storage, and sea water electrolysis to produce hydrogen, not to mention robotics, autonomy, and AI.”
The University of Southampton also has immense offshore energy capability across a wide range of other disciplines.
“In life sciences, colleagues are working to understand the effect of offshore energy on marine ecology. In social sciences, expertise spans the regulatory and policy seascape, logistics for optimisation of inspection, maintenance and repair of offshore infrastructure, and the social and political tensions and considerations around offshore wind developments,” Susan outlined.
“Within arts and humanities, expertise covers cultural and heritage aspects of interventions in the oceans, as well as literature, music and art as media for communicating, sharing and evolving knowledge of our oceans and our interventions within them.”
Through the Southampton Marine & Maritime Institute (SMMI), the University has a unique mechanism for harnessing the interdisciplinary expertise of its academics alongside external stakeholders to develop an integrated response to offshore energy ambitions.
Chair in Emerging Technologies
The Royal Academy of Engineering Chair in Emerging Technologies scheme aims to identify and provide long-term support to global research visionaries, developing emerging technology areas that have potential to deliver economic and social benefit to the UK. Susan was awarded a Royal Academy of Engineering Chair in Emerging Technologies for Intelligent & Resilient Ocean Engineering (IROE) in 2019.
“The activities of my Royal Academy of Engineering Chair in Emerging Technologies centre around addressing technology gaps at each stage of the engineered life cycle of ocean structures, from forecasting ocean and seafloor behaviour to designing and operating novel platforms for ocean facilities,” explained Susan. “By harnessing the potential of sensors, robotics, and AI, a next generation of resilient, engineered systems will unlock ocean resources more efficiently and more sustainably, with less risk to life.”
The Royal Academy of Engineering Chair in Emerging Technologies scheme aims to identify and provide long-term support to global research visionaries, developing emerging technology areas that have potential to deliver economic and social benefit to the UK.
The Centre of Excellence for Intelligent and Resilient Ocean Engineering (IROE) at the University of Southampton, supported by Susan’s Chair in Emerging Technologies, drives activities to create a step-change in ocean engineering design to support responsible and economic use of ocean resources. “Although at the core we develop engineering solutions, our activities reach beyond engineering through engaging across disciplines, and working with the public and policy makers to raise awareness of ocean engineering and guide policy for future use of our oceans.” said Susan.
IROE works with over 30 external partners and, together with academics across multiple schools and faculties including engineering, computer science, ocean and earth science, biological sciences, law, policy, sociology, English, art, and archaeology, undertakes activities to drive forward the development of safe, efficient and responsible offshore energy.
The elements which need to come together to create practical and sustainable offshore energy solutions are varying and complex. Susan and her colleagues from across the university have detailed some of the challenges and solutions below, along with projects they are currently undertaking.
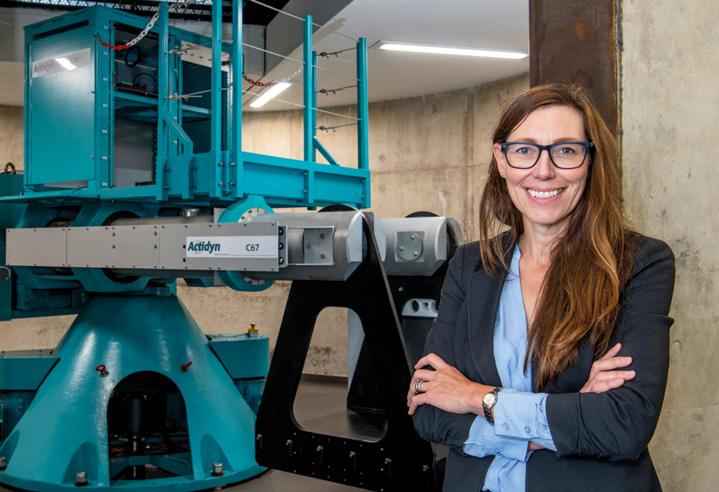
SEABED CHARACTERISATION
Offshore energy facilities, whether fixed wind turbines, floating wind turbines, tidal or wave energy devices, need to be secured to the seabed by foundations or anchoring systems to keep them in place and sufficiently stable, whilst other infrastructure such as cables between turbines are trenched into the seabed. As such, the engineering design of foundations, anchors and cables to facilitate offshore energy relies on accurate characterisation of seabed properties.
Characterising seabed properties sufficient for engineering design at a large scale for offshore wind turbines is a key challenge in the energy transition sector.
Susan explained: “The move from a single offshore hydrocarbon platform to an offshore wind farm of tens or hundreds of turbines, requires characterisation of an area of seabed far greater than that of a single platform.
“A second key challenge is characterising engineering properties of the seabed over a meaningful time scale. As seabed properties change, they evolve in response to the loading applied to the seabed from the installed infrastructure, such as turbines.”
The Centre of Excellence for Intelligent and Resilient Ocean Engineering is home to one of the largest groups in offshore geotechnical engineering in the country and is working on several solutions in this area, using micro-finite element analysis to develop machine learning methods that derive geotechnical properties from geophysical data.
“The benefit of geophysical site data is that it is continuous, either in 2D or 3D, whilst geotechnical site investigation data provides at best a 1D depth profile at discrete and usually sparse locations. However, geophysical data does not provide the engineering properties needed for design,” outlined Susan.
“Some initial work exists on deriving geotechnical properties from the seismic data of a geophysical survey, but the range of properties that can be extracted, and the accuracy, is limited,” notes Dr Jared Charles, an early career researcher in the IROE Centre of Excellence, working on this research.
To address this issue researchers in the School of Engineering and the School of Ocean and Earth Science, in collaboration with industry partner SAND Geophysics, are developing relationships between the fundamental geophysical and geotechnical parameters to enable sufficient accuracy and resolution for engineering design and derivation of a wider range of geotechnical properties.
A second project is developing a theoretical framework for ‘Whole-life geotechnical design’, such that the characterisation of evolving seabed properties can be routinely incorporated into engineering design.
“Experimental and numerical protocols are being used to characterise the changing seabed properties with loading and time. This maps onto a design framework and can be applied to the geotechnical design of foundations and anchors for offshore wind,” said Dr Katherine Kwa, RAEng Research Fellow within the IROE Centre of Excellence, working on this topic.
“The whole-life geotechnical design project is being pursued in collaboration industry partners NGI and Lloyd’s Register, ensuring the research remains relevant and has a pathway for uptake in engineering practice.” said Susan.
FOUNDATIONS, ANCHORS AND MOORINGS
To meet global and national targets for offshore wind at the pace required, foundations for fixed wind turbines and anchors and mooring lines for floating offshore wind need to become more efficient in their design and performance.
Currently, the supply chain is struggling to meet the challenging demand for materials and manufacture involved in delivering offshore wind farms. This is due to a combination of insufficient engineering resource/expertise along with the high costs involved using current technology and approaches.
The University of Southampton is addressing these challenges with a range of expertise and facilities housed within the National Infrastructure Laboratory on UoS’ Boldrewood Innovation Campus.
Geotechnical engineering researchers are working on a range of solutions for next generation foundation and anchoring systems to support offshore wind.
These include:
• Whole-life geotechnical design methods, that take advantage of evolving soil properties to optimise foundation and anchor designs
• Shared anchors – such that several floating wind turbines are attached to a single anchor, therefore reducing the number of anchors required
• Silent anchor solutions, such as ‘screw piles’ and ‘suction caissons’ that minimize impact on marine mammals during installation
• Extensible mooring systems to reduce peak load with extra but controlled compliance, such that smaller anchors can be used
• Integrating hydrodynamic, mooring line and geotechnical foundation or anchor design.
Dr Katherine Kwa is developing integrated station-keeping solutions to enable floating offshore renewable energy: “By integrating floatermooring line and geotechnical response, our research has shown that anchors can carry up to two-times more load compared to that predicted with current design methods, whilst retaining the same reliability.”
In combination with new design approaches that enable more efficient anchor designs, additional efficiency can be achieved by using fewer anchors to secure the floating wind turbines to the seabed.
“The total number of anchors required for large offshore wind farms can be reduced three-fold by utilising shared anchors, for example by attaching three mooring lines belonging to three different floating wind turbines to a single shared anchor,” explained Dr Benjamin Cerfontaine, lecturer in Geotechnical Engineering. “This reduces seabed disturbance in installing the anchors, as well as reducing the raw material used and the installation time, thus reducing the carbon footprint of the turbine, and pressure on the supply chain for anchors.”
Many of the design solutions being developed harness the potential of big data and machine learning to provide optimised outcomes that were previously unachievable for routine design.
This work to develop the next generation of offshore foundations, anchors and mooring systems, particularly to unlock offshore floating wind, is being carried out within the IROE. It is supported by the Royal Academy of Engineering Chair in Emerging Technologies, a RAEng Early Career Fellowship, and the ORE Supergen Hub, in collaboration with various external partners including NGI, Lloyd’s Register, Equinor, Corpower Ocean, ORE Catapult Floating Offshore Wind Centre of Excellence and The Alan Turing Institute.
ENERGY STORAGE AND GREEN HYDROGEN
The source and cost of energy are among the biggest challenges in today’s industrial society, together with environmental pollution, climate change and sustainability.
According to the International Renewable Energy Agency, the investment in renewable energy sources attracted 43% and 35% for solar and wind offshore power respectively, from the total investment in 2020 while the levelized cost of electricity (LCOE) generated from solar and wind, decreased by 85% and 56%, respectively from 2010 to 2020, demonstrating the increasing importance of renewable systems development.
Carlos Ponce De Leon Albarran, Professor of Electrochemical Engineering, is working to address the challenge that the intermittent nature of solar and wind power presents. A key area of focus is the energy storage system, which needs to harvest energy efficiently during periods of high energy generation, and subsequently be able to deploy energy when there is no wind, or at night.
He explained, “Energy can be stored in various forms: chemical, electrical, electrochemical, mechanical, and geothermal. Among these different energy storage systems, the electrochemical method can be used to store the energy in two ways: by generating green hydrogen via seawater electrolysis and by using redox flow batteries.”
The first system, seawater electrolysis, is a challenging process because it can generate toxic oxychloride intermediates and chlorine gas, which are harmful to organisms and very corrosive. At the Electrochemical Engineering Laboratory in the Mechanical Engineering Department, Carlos and his colleagues work on alternative catalysts to suppress the formation of chlorine and oxychloride compounds during seawater electrolysis, helping to generate green hydrogen with no harmful waste.
“The second option, to store energy in Redox Flow Batteries, is typically used for large amounts of electricity,” said Carlos. “At the Electrochemical Engineering Laboratory, we work on Redox Flow Batteries systems such as zin-cerium, vanadium, and soluble lead acid batteries. A current project, Reclaimed Electrolyte Low Cost Flow Battery, is being funded by the Faraday Institution. We’re also working in collaboration with stakeholders in the UK and Africa on a system that provides grid stability and an efficient energy supply which also includes a techno-economic assessment.”
TYING IN TIDAL AND WAVE
Tidal and wave energy could supply a significant proportion of the UK’s electrical power and that of many other countries in the world.
“The environment is challenging with significant forces to consider such as corrosive seawater, and the obvious complication of working both offshore and underwater,” explained Dr Luke Myers, Associate Professor, Energy and Climate Change Division in Civil, Maritime and Environmental Engineering & Science. “Until recently, high costs and high risks for new technology severely restricted development as companies struggled to raise funding for devices to be installed.”
The increase in wholesale electricity prices in recent months has meant the cost issue has been somewhat removed, however the technical challenges and risk remain at this emerging stage of development.
“Tidal energy offers completely predictable, renewable energy generation,” said Luke. “Devices appear and operate in a similar manner to wind turbines but marinized. They are designed to use, and survive in, harsh, corrosive saltwater conditions, extracting the kinetic energy of the fast-moving waters.”
Work within the University’s Energy & Climate Change Division has covered many areas of this technology including blade design, resource assessment and operation within arrays or farms.
Luke outlined, “Our strength lies in our experimental work. Here we design and operate models that we test in large hydraulic facilities across Europe. These turbines are fully instrumented to measure power, forces and other operational parameters which we can then scale up to predict performance of larger turbines.”
Most recently, Luke and his colleagues measured the increase in performance offered by using blade winglets (see image) similar to those we see on commercial aeroplanes. Working within the University of Southampton’s towing tank facility, located on the Boldrewood Innovation Campus, they were able to quantify significant increases in power, results of which can help further reduce the costs and decrease risks.
ECOSYSTEM EFFECTS
The ecological implications of the structural changes to offshore electricity generation are not well understood. They are further complicated by the need to balance growth of the renewable energy sector with competing policy demands, such as biodiversity protection, achieving net environmental gain and restoration, sustainable fisheries and UK Marine Strategy targets.
“Ecosystem-level impacts of offshore renewable development cannot be fully understood without understanding the impact of a range of pressures on the diverse array of species and habitats affected,” explained Martin Solan, Professor of Marine Ecology in Ocean and Earth Sciences. “Coastal and marine waters host a disproportionately large fraction of productivity, maintain high levels of biodiversity, and are of significant importance in regulating major nutrient cycles and greenhouse gas exchange.”
A key challenge is understanding the effects of a multitude of pressures that are associated with renewable energy, such as noise, vibration, electromagnetism and physical disturbance; and then distinguishing their effects from natural variability in the system, other human caused activities and the expression of climate change.
“We have found that previous work in this area has focused on the presence of species, with the assumption that if a species is present then there is no negative impact,” said Martin. “However, this approach lacks information on species-environment interactions and their role in mediating important ecosystem processes within the context of a busy and crowded shelf sea.”
Martin and colleagues are working to understand how a large increase in deployment of offshore wind, combined with other man-made and climate change related pressures, will affect species-environment interactions that underpin the functioning of UK marine ecosystems.
“Our team includes expertise in physics, engineering, ocean and earth science, fish and invertebrate ecophysiology and ecology, marine observation technology, computational modelling and socioeconomics,” outlined Martin. “Our experience and multi-disciplinary expertise are strengthened by early career scientists that bring leadingedge expertise in computation and machine learning, behavioural ecology, physiology, the development of marine technology and seabed-offshore wind infrastructure interactions, including high voltage cables, noise and vibration.”
Together, Southampton researchers and academics are enabling a stepchange in marine observations by combining autonomous underwater vehicle derived datasets with innovative, high-level machine learning techniques to quantify ecological dynamics and test ecosystem outcomes for a range of representative seascapes.
“The research taking place in Southampton is providing understanding of the balance between positive and negative changes for both habitats and species caused by offshore renewable development. Hence, our research outputs offer industry, regulators and policy makers tasked with implementing robust approaches to marine environmental recovery, biodiversity and net environmental gain, the evidence base required to judge the feasibility and sustainability, including feedbacks and trade-offs, of different future scenarios,” concluded Martin.
CULTURAL AND HERITAGE CHALLENGES
“The sea is a complex place, home to remnants of our shared past in the form of submerged landscapes, sites of habitation and shipwrecks. It holds a critical sedimentary archive charting our changing climate and environment, as well a crucial contemporary part of culture – a place people work, visit and imagine,” said Fraser Sturt, Professor of Archaeology. “Each cable or turbine installation has a potential impact on that physical, cultural and environmental archive as well as shaping intangible heritage. The challenge is to identify, quantify and help mitigate those impacts whilst also ensuring a route to sustainability.”
Southampton’s expertise in this area lies in cutting edge data collection and analysis, of both quantitative and qualitative forms.
“Working with colleagues in Electronics and Computer Science, and in collaboration with all national heritage agencies, we are pioneering the use of artificial intelligence to leverage disparate but highly informative existing archives to transform understanding of UK waters, through a project called Unpath’d Waters.” explained Fraser. “This, matched to work on data collection and identification of cultural and heritage assets, also using AI, forms a critical part of the consenting process for offshore development. We are one of only a few institutions in the UK able to field researchers capable to physically work underwater as well on land, engaging with the material record as well as contemporary communities.”
The research Fraser and colleagues are working on is changing data availability and understanding in terms of how cultural and heritage assets are identified, both in terms of quantitative and qualitative data, and from this how the impact from sustainable development can be mitigated.
ROBOTICS AND SENSING
The ever-increasing amounts of offshore wind turbines are connected via hundreds of thousands of kilometres of subsea cables, operating robustly and reliably to fulfil UK energy demands.
University of Southampton researchers have been working on automated methods to inspect and maintain the hundreds of thousands of kilometres of subsea cables that connect offshore wind turbines. These cables are essential to keep wind turbines operating robustly and reliably to fulfil UK energy demands.
Blair Thornton, Associate Professor of Oceanic Engineering, explained, “Underwater robots equipped with high-resolution cameras, laser scanners, and manipulators could perform the inspection and maintenance needed to support offshore renewable operations if they could be deployed at sufficient scale. The challenge is decoupling their operation from crewed ships currently needed to manage robotic operations. Ships account for the vast proportion of present-day operational costs and carbon emissions, and so limit the scalability of robotic operations.”
To decouple submersible robot operations from crewed ships, it is necessary to improve their endurance, reliability and intelligence. Southampton expertise in energy harvesting, risk modelling, sensing, data interpretation and mission planning forms key parts of the puzzle to advance marine autonomy.
“Our research develops the high-resolution sensing and data processing capabilities needed for robots to observe and interpret their surroundings, and understand their own condition,” explained Blair. “This allows robots to make independent decisions that support high-level mission objectives and summarise information so that it can be communicated over low bandwidth and intermittent communication windows for the attention of human experts and operators located anywhere in the world. They can then monitor mission progress and update robot tasks without the need for physical human presence on nearby ships.”
OCEAN SPATIAL PLANNING
David White, Co-Director of the EPSRC Supergen Offshore Renewable Energy Hub, and Professor in Infrastructure Geotechnics, is asking the question: ‘Where in the ocean should new offshore energy infrastructure be placed?’
“Ocean space is already under pressure. Large areas are already set aside for human related uses including fishing, shipping and cable routes,” he explained. “Meanwhile, the ocean hosts a range of ecosystems in the water column, the airspace and the seafloor. There are also hazards beneath the waves that hamper the engineering of infrastructure. UK waters are particularly congested, and new offshore wind sites are already moving over the horizon into deeper water, further from shore, as the most convenient sites for wind farms have already been utilised.”
SMMI has expertise across the range of ocean uses, as well as the breadth of environmental conditions. Drawing on this expertise, David and colleagues have been able to assemble digital models of the ocean, quantifying the overlapping constraints and interactions.
David outlined, “The Supergen Hub scenario modelling allows us to assess the ocean space needed to meet net zero and can simulate the regional distribution of future wind farms, and their interaction with existing ocean activity.”
This future ocean scenario modelling provides a platform for all ocean stakeholders to communicate and collaborate on strategy for offshore wind deployment. Dr Hugo Putuhena, Research Fellow in Offshore Renewable Energy, explains, “Meeting net zero is essential to mitigate climate change, but this must be achieved with minimal negative impact on other human and natural activity”.
Some of the net zero scenarios that Dr Putuhena has analysed would involve more than 50 per cent of the UK’s available ocean space being used for offshore wind by 2050. Much of this growth would be accommodated in the Celtic Sea to the southwest of the UK.
SAFE AND SECURE CABLE CONNECTIONS
Each offshore wind farm has a network of cables that connect the wind turbines to a local sub-station, and an export cable that transmits the power back to shore. These cables are usually buried in a trench to avoid damage from fishing activity or ship’s anchors. This burial provides unwanted thermal insulation that can cause the cables to heat up, limiting their maximum power transmission to avoid damage.
The cable thermal behaviour is controlled by a multitude of factors, ranging from the changing ocean temperature, the geology and seabed conditions, the material properties of the cable components, and the operation of the electrical system. SMMI has experts across all of these disciplines who have been working together for the past decade to support cable designers and wind farm developers. “By better understanding the environment around the cables, we’re able to optimise their design and operation and reduce costs significantly,” said Professor Justin Dix, of the School of Ocean and Earth Science.
Recent work has focused on improving the assessment of seabed properties during cable design. New methods for assessing seabed properties have been evaluated, and guidance has been written for a consortium of wind farm operators.
“We’ve worked with industry to interpret their evidence of the seabed conditions around cable trenches. This has helped us to better predict the insulation effect on operating cables,” said Professor David White.
Meanwhile, laser-based monitoring systems are providing valuable new evidence of the temperature in operating cables. Dr George Callender, of the School of Electronics and Computer Science explained, “We have developed new, efficient computer codes that allow these temperature measurements to be used to assist real-time cable rating and burial-depth prediction. All of these technologies help to connect us more securely to our offshore renewable energy.”