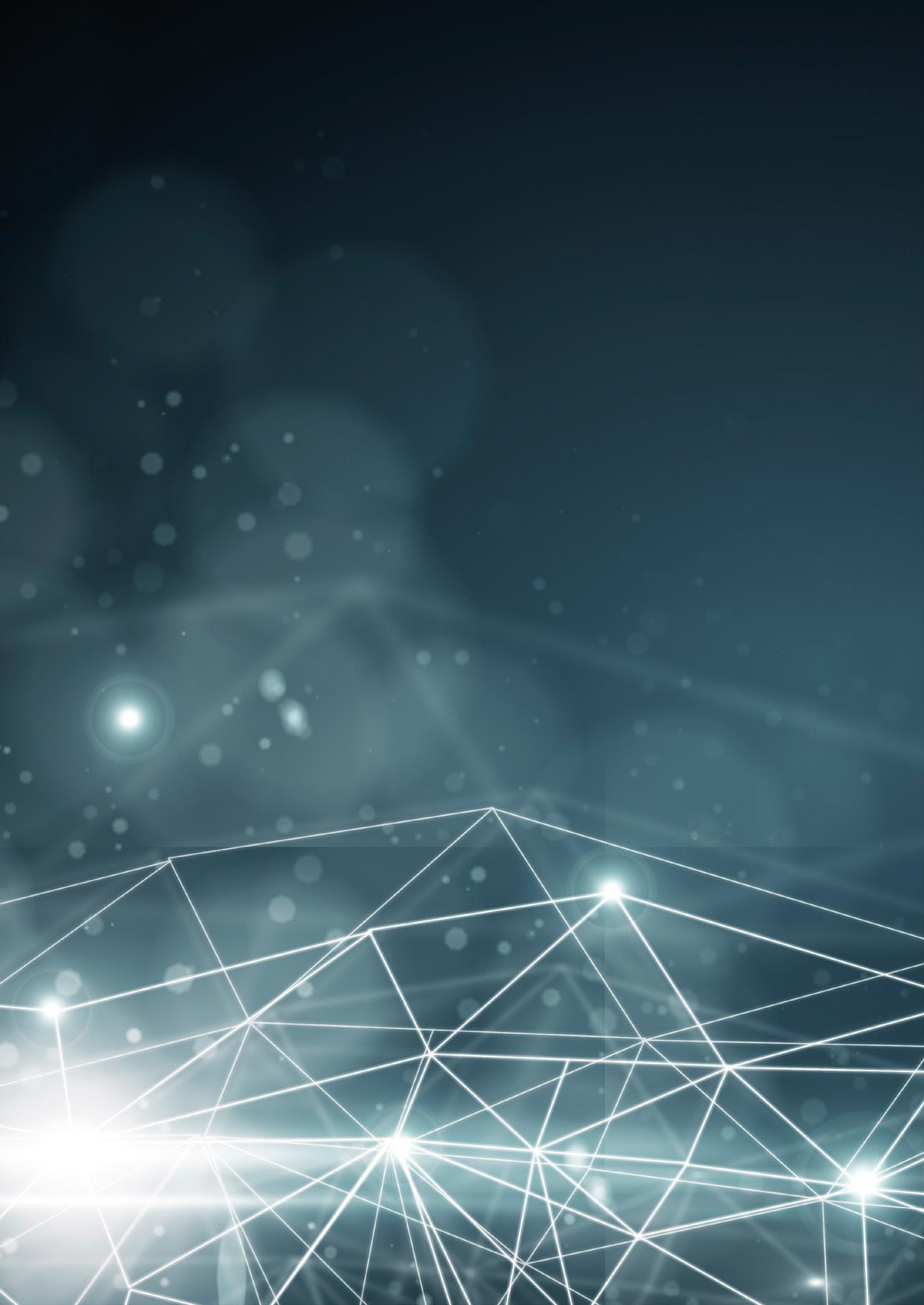
5 minute read
INTRODUCING 6G: THE FUTURE OF WIRELESS COMMUNICATIONS
We’re all familiar with the term ‘5G’, the wireless network which promises us increased capacity, huge connectivity and exciting applications. You may not have heard of 6G, a future information network which has the ambitious objective of integrating satellites, planes, drones and even underwater nodes with the terrestrial, ground-based 5G network to offer truly global wireless communications – anywhere and anytime.
Professor Lajos Hanzo, in the School of Electronics and Computer Science, is working with a team to pioneer the enabling techniques of the 6G network, funded by a European Research Council (ERC) grant of €2.5 million.
“5G wireless systems are still ground-based, so they have the same coverage limitations as other terrestrial networks,” Lajos explained. “Space-communication networks are complementary to terrestrial networks, as they provide vast communication coverage for people and vehicles at sea, as well as in remote rural areas and in the air.”
The challenge
As demand for wireless connectivity increases, the radically new concept of integrating terrestrial networks with space networks is constantly evolving. Creating this space air-ground integrated network (SAGIN) is critically important for the socalled vertical industries such as logistics, mining, agriculture, fisheries and defence. However, there are several significant technological challenges which must be overcome to create this SAGIN system.
“SAGIN is expected to be at the core of 6G communication systems, and how to build a high-capacity yet low-cost system is of significant importance in developing 6G globally,” said Lajos. “One of the bottlenecks is the limited bandwidth available for highrate aerial backbones, this backbone being the high-speed communications link. Another challenge is that the efficiency of direct air-to-ground communications between airborne and ground-based network elements is limited, since the ‘footprint’ of these air-toground beams on the ground is large, which severely splits down the throughput available for a single user in areas of high user density.
“Aerial backbones rely on high-throughput wireless links to connect the major nodes in the SAGIN. They play a pivotal role by handling the aggregation and distribution of various data flows, such as voice, video, Internet and other data sources. Because the airborne network is an indispensable intermediate layer between space-borne and ground-based networks, flexible high-rate and all-weather aerial backbones constitute the most critical infrastructural elements in building the airborne network.”
The solution
Lajos and his team are proposing a novel architecture for realising a high-capacity, low-cost SAGIN by exploiting the existing civil airliner network for breaking these bottlenecks at a low cost.
Lajos explained: “The civil airliner network covers a large area of the global land and sea surface and has sufficient plane density to form a major contributor of a dynamic airborne network. The mm-wave (millimetre wave is the band of radio spectrum between 30 and 300 GHz that can be used for high-speed wireless communications) links needed for a SAGIN potentially provide very high bandwidth both for the backbone and for access to SAGIN services.
“Under this new SAGIN architecture, using mm-wave links in the airborne network, as well as between the airborne and ground-based networks, will significantly increase the overall system capacity,” added Lajos. “Using mm-wave hybrid antenna arrays will strike a balance between performance and cost, plus using civil airliners as the relay nodes for the airborne network will substantially reduce the cost of building a dedicated SAGIN infrastructure.”

Professor Lajos Hanzo
The future
According to FlightAware, the world’s largest flight-tracking data company, there are on average tens of thousands of planes carrying more than 1.2 million people in the sky at any given time. These planes cover most of the land and sea globally, and hence can provide networking services supported by the SAGIN with vast coverage both day and night. In addition, because these airborne platforms are only about 10 kilometres above the ground, the coverage area of a signal beam can be well controlled using mm-wave hybrid antenna arrays to support high user density at a much lower delay than the satellites.
Lajos is excited at the prospect: “The proposed integration of spaceborne and airborne networks, along with ground-based networks, is capable of improving the global communication coverage which is the main objective of 6G networks.” An example scenario for the proposed SAGIN is passengers in a plane who can make phone calls and access the Internet using their mobile phones as if they were at home or at their workplace, enjoying a better user experience and faster download speeds than when using the in-flight Wi-Fi services currently available. Other examples include mining, smart farming, shipping and tracking in sparsely populated remote areas.
Rural and remote areas have been missing out on the wealth-creation benefits of broadband for many years, due to the lack of connectivity. The main obstacle for rural broadband deployment has been the high cost of groundbased infrastructure. The proposed SAGIN provides a solution with its vast coverage at low cost, bringing smart mining, farming and precision-agriculture technologies to rural and remote farms to finally complete the missing link in global connectivity. For supply-chain tracking and shipping applications, low-cost and vast global connectivity will enable detailed tracking of containers at sea and livestock in rural and remote areas, closely monitoring their shipping and roaming conditions respectively.
Naturally, due to the mobility of passenger planes, a dynamic airborne network can provide only a best effort rather than qualityguaranteed enhancement of existing high-rate services. Therefore, there is a trade-off between cost and quality of services. Again, to provide more reliable service, a limited number of dedicated aerial nodes such as tethered airships and so-called high-altitude platforms could be deployed to supplement the scheduled civil airliners.
Building blocks
This project is based on Lajos’ second ERC grant. The first was carried out between 2013 and 2018 under the Beam-Me-Up project, in which he and his team expanded the horizon of classic Radio-Frequency (RF) systems to the previously rarely used 30 – 60 GHz mmwave spectral bands and to optical wireless frequency bands based on both visible light and on free-space optical links, paving the way for this SAGIN concept.
They aimed to overcome the predicament that as the carrier frequency is increased, its wavelength is reduced and hence rain-drops, vegetation and other factors weaken the signal more severely. BeamMe-Up conceived sophisticated, high-gain beamforming solutions for counteracting this increased reduction. For indoor optical wireless communications, Beam-Me-Up designed sophisticated solutions relying on Light-Emitting Diode (LED) based downlink transmitters using state-of-the-art LED-bulbs. These solutions may be expected to find their way into next-generations Beam-Me-Up-style tele-presence systems. The final piece in this challenging jigsaw puzzle is to conceive highsecurity quantum-domain solutions as part of the QuantCom ERC project for ultimately replacing the above-mentioned links of the SAGIN one by one in this cutting-edge multihop network, as QuantCom evolves.
Since research is a collaborative endeavour, in closing Lajos expressed his sincere gratitude to the entire Beam-Me-Up and QuantCom teams for their visionary contributions and for their dedication to the project, including but not limited to Drs Dimitrios Alanis, Panagiotis Botsinis, Zunaira Babar, Daryus Chandra, Jingjing Cui, Dong Liu, Tiep Hoang, Soon-Xin Ng, Chao Xu and Jiankang Zhang.