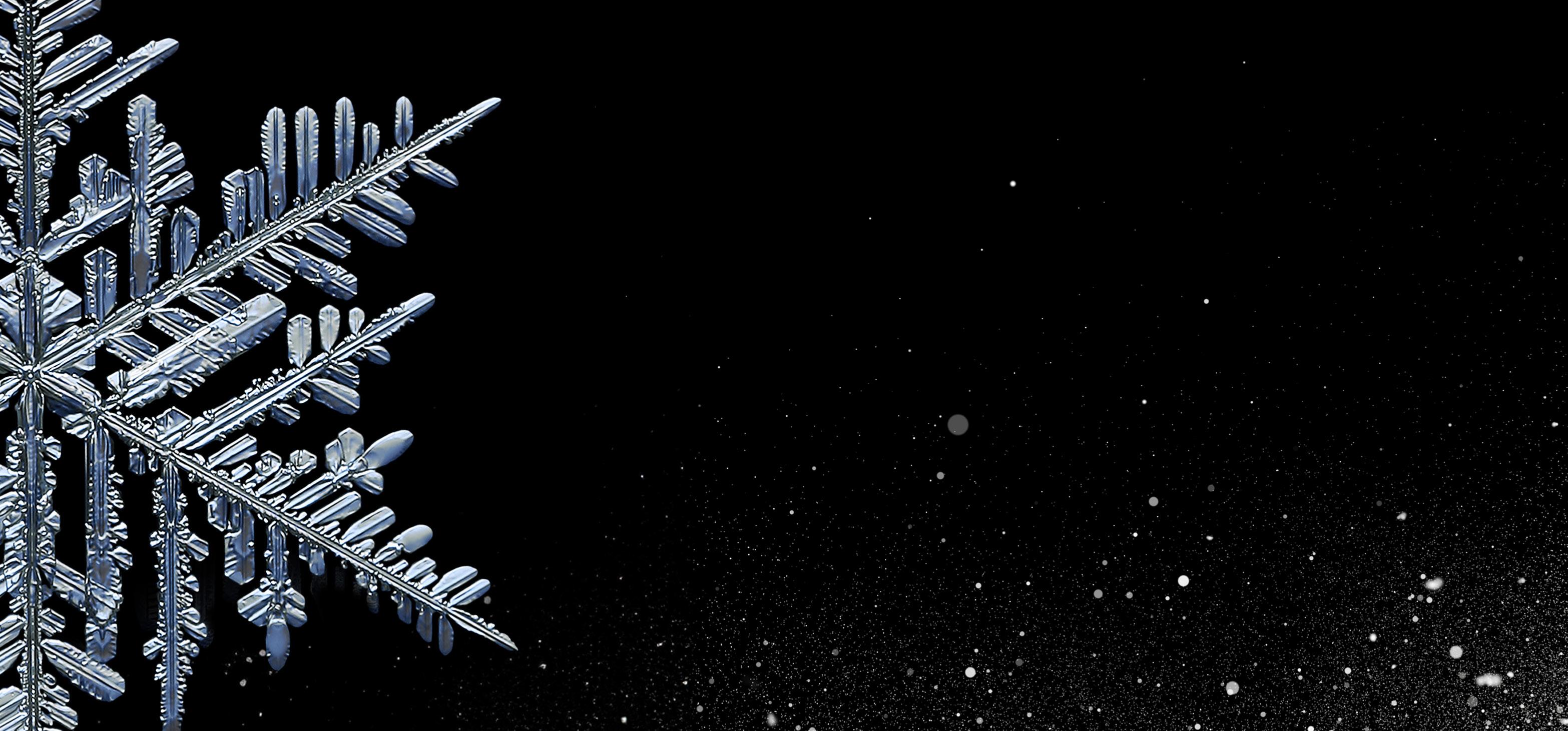
4 minute read
THE SCIENCE BEHIND SNOWFLAKES
Utah's Researchers Discover that Snowflake Movement is Astonishingly Predictable
TIM GARRETT HAS DEVOTED HIS SCIENTIFIC CAREER TO CHARACTERIZING SNOWFLAKES, THE PROTEAN PARTICLES OF ICE THAT FORM IN CLOUDS AND DRAMATICALLY CHANGE AS THEY FALL TO EARTH.
Now the University of Utah atmospheric scientist is unlocking the mystery of how snowflakes move in response to air turbulence that accompanies snowfall using novel instrumentation developed on campus. And after analyzing more than half a million snowflakes, what his team has discovered has left him astonished.
Rather than something incomprehensibly complicated, predicting how snowflakes move proved to be surprisingly simple, they found.
“How snowflakes fall has attracted a lot of interest for many decades because it is a critical parameter for predicting weather and climate change,” Garrett says. “This is related to the speed of the water cycle. How fast moisture falls out of the sky determines the lifetime of storms.”
LETTERS FROM HEAVEN
The famed Japanese physicist Ukichiro Nakaya termed snow crystals “letters sent from heaven” because their delicate structures carry information about temperature and humidity fluctuations in the clouds where crystal basal and prism facets compete for water vapor deposition.
While every snowflake is believed to be unique, how these frosty particles fall through the air—as they accelerate, drift and swirl—follows patterns, according to new research by Garrett and colleagues in the College of Engineering. Snowflake movement has important implications for weather forecasting and climate change, even in the tropics.
“Most precipitation starts as snow. The question of how fast it falls affects predictions of where on the ground precipitation lands, and how long clouds last to reflect radiation to outer space,” says Garrett. “It can even affect forecasts of a hurricane trajectory.”
Also involved with the study published in the journal Physics of Fluids are Dhiraj Singh and Eric Pardyjak of the U’s Department of Mechanical Engineering. The research was funded by the National Science Foundation.
To study snowflake movement, the team needed a way to measure individual snowflakes, which has been a challenging puzzle for years.
“They have very low masses. They may only weigh 10 micrograms, a hundredth of a milligram, so they cannot be weighed with very high precision,” explains Garrett.
Working with engineering faculty, Garrett developed instrumentation called the Differential Emissivity Imaging Disdrometer, or DEID, which measures snowflakes’ hydrometeor mass, size, and density. This device has since been commercialized by a company Garrett co-founded called Particle Flux Analytics. The Utah Department of Transportation has deployed the equipment in Little Cottonwood Canyon to help with avalanche forecasting, he says.
For Garrett’s field experiments, his team set up at Alta, the snowiest place in Utah, for the winter of 2020-21. The instruments were deployed alongside measurements of air temperature, relative humidity, and turbulence and placed directly beneath a particle tracking system consisting of a laser light sheet and a single-lens reflex camera.
“By measuring the turbulence, the mass, density, and size of the snowflakes and watching how they meander in the turbulence,” Garrett says, “we are able to create a comprehensive picture never obtained before in a natural environment before.”
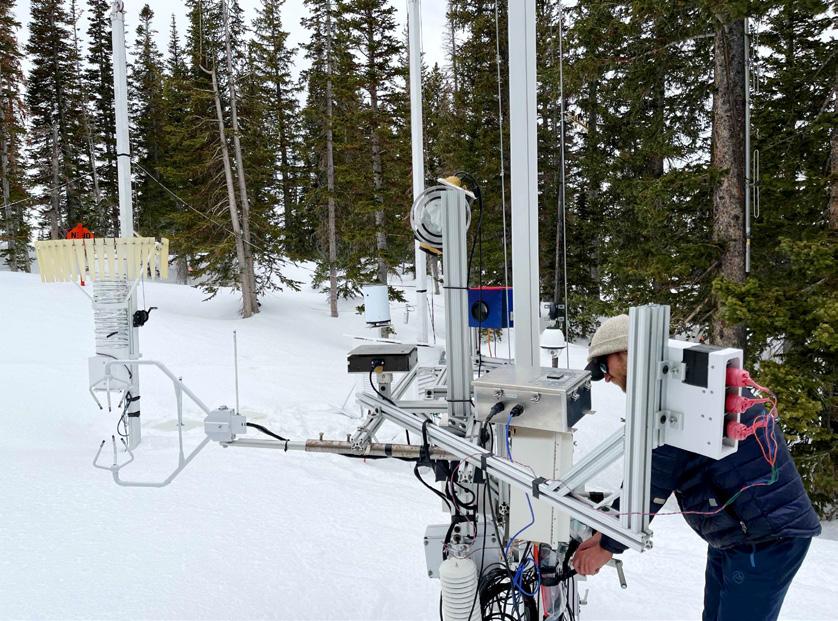
UNEXPECTED FINDINGS
Despite the intricate shapes of snowflakes and the uneven movement of the air they encounter, the researchers found they could predict how snowflakes would accelerate based on a parameter known as the Stokes number (St), which reflects how quickly the particles respond to changes in the surrounding air movements.
When the team analyzed the acceleration of individual snowflakes, the average increased in a nearly linear fashion with the Stokes number. Moreover, the distribution of these accelerations could be described by a single exponential curve independent of the Stokes number.
The researchers found that the same mathematical pattern could be connected to how changing snowflake shapes and sizes affect how fast they fall, suggesting a fundamental connection between the way the air moves and how snowflakes change as they fall from the clouds to the ground.
“That, to me, almost seems mystical,” Garrett says. “There is something deeper going on in the atmosphere that leads to mathematical simplicity rather than the extraordinary complexity we would expect from looking at complicated snowflake structures swirling chaotically in turbulent air. We just have to look at it the right way, and our new instruments enable us to see that.”
This article originally appeared in @TheU.