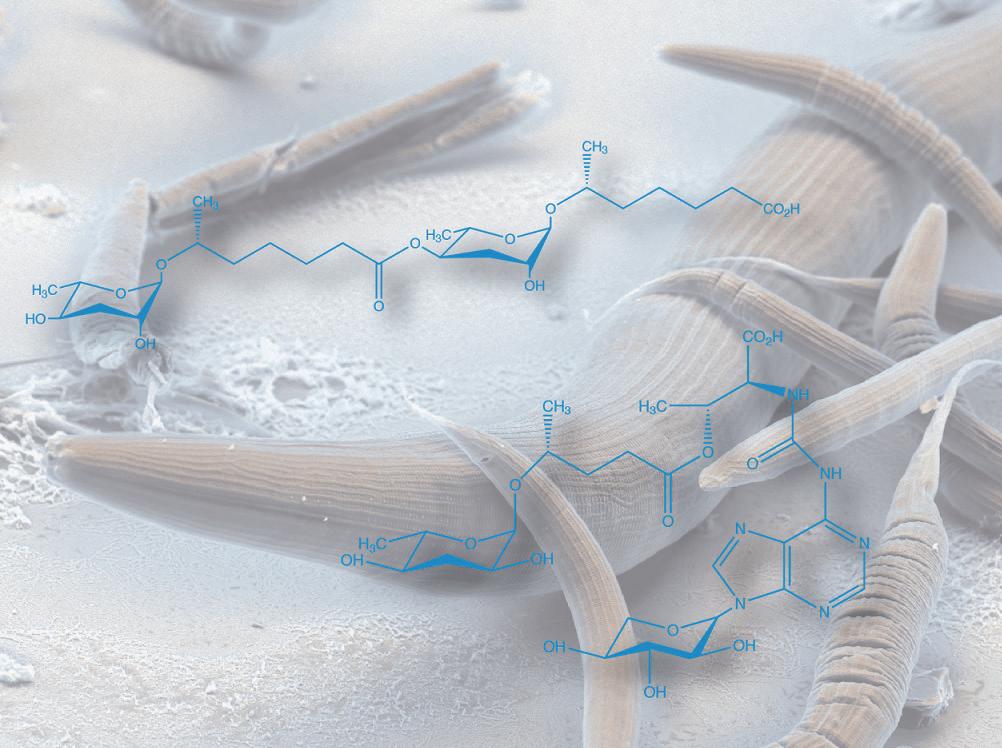
6 minute read
The Nature of Nurture
For years after the discovery of the double-helix molecule DNA most biologists assumed that DNA was the sole hereditary molecule, i.e. the only molecule that contains information which can be passed on to the next generation. While DNA is indeed the molecular carrier of hereditary information, lower or higher expression of genes in that database can be affected by the environment wherein an organism exists.
As a PhD candidate at the University of Chicago, new Assistant Professor Michael S. Werner first studied differentiation of stem cells and leukemia cells determined by differences in gene expression. Some of the factors that affect gene expression to keep genetically identical cells distinct from each other (e.g. a kidney cell vs. a heart cell) are chemical modifications to chromatin–the assemblage of DNA and proteins that constitute the physiological form of heritable information. {read more below}

Michael D. Werner, Assistant Professor, School of Biological Sciences
Matt Crawley
“Some of these modifications,” says Werner, “can be copied through cell division—effectively providing an additional form of heritable information, providing long term changes to gene expression well beyond the initial inductive signal…that results in the stable development of divergent cells, tissues, and ultimately organs.” These mechanisms which do not involve an underlining change in DNA sequence are referred to as epigenetic.
As a post-doctoral fellow at the Max Planck Institute, Werner expanded this inquiry to the study of alternative organismal characteristics. The sets of observable characteristics of an individual resulting from the interaction of its genotype with the environment is known as a phenotype, and the ability to form different phenotypes in response to different environments is referred to as phenotypic plasticity. What Werner finds
most intriguing, and is the major focus of his laboratory, is the potential link between the epigenetic chromatin modifications that provide for alternative cell types, and the unknown molecular mechanisms that provide for alternative organismal phenotypes.
There was another reason Werner took the leap from studying cells to plasticity in organismal phenotypes. “We’ve learned a lot about the mechanisms that provide cellular plasticity, and I think the time is now ripe to expand the scope of some of our questions,” he says. For example, “What are the proximate molecular mechanisms that transmit environmental information into physiological, behavior, and morphological changes at the organismal level?” The implications of this research, according to Werner, may also inform medical scientists who continue to investigate environmental influence on health and well-being, such as “learning, immunity, and the effects of diet and exercise.”
For Werner, the ideal organism subject model would become Pristionchus pacificus, a nematode similar in characteristics to its more famous cousin C. elegans. But depending on conditions it experiences as a juvenile, Pristionchus develops either a narrow mouth, or a wide mouth with an additional “tooth,” the latter of which is useful for killing competitors and expanding the organism’s dietary range. What provides this trait plasticity, and causes the switch in juveniles from the development of a narrow to a wide mouth?
The hypothesis that Werner is tracking down is that they are the same mechanisms that provide cellular plasticity and differentiation he studied in human cell lines as a PhD candidate in Chicago. “The ability to use a genetic model organism like a nematode provides an incredible advantage to study phenotypic plasticity, which has historically been studied in fascinating, yet less experimentally tractable organisms like butterflies, ants, crustaceans, and of course, humans.”
Today, Werner and his lab grow worms in different environmental conditions, switching them from plates to liquid culture in a flask. Depending on how developed each organism is when the switch is made determines which mouth form emerges. This would suggest that there is “memory” of their previous environment. His task is to find which epigenetic mechanisms maintain a record of environmental exposure, which genes are responding to the environment, and when.
After identifying the causative molecular mechanisms, Werner intends to see how “conserved” and general they are, and how chromatin and plasticity have shaped ecological strategies and evolutionary variation. When he’s not publishing his findings in journals like Nature Structural and Molecular Biology and Genome Research, Werner and his lab spend a good deal of their time grinding up biological material, biochemical fractionation and sequencing before going “under the ‘scope.” If you were to ask him what kind of biologist he is, he side-steps firewall divisions, preferring to think of himself as just “a biologist.”
In an age when the life sciences has become more and more specialized, Werner is committed to the idea of research that is cross-disciplinary, which was one of the reasons he found the University of Utah’s School of Biological Sciences an attractive home for his work, and conducive to his scientific imagination. “Biology is no longer a young field,” he says, “as it was in the second half of the 20th century,” during the heyday of molecular biology when the focus was on the huge questions like “what is the molecule of hereditary information, and how does it copy itself?” While there are still important questions delving deeper into each subfield of biology, Werner believes that now the big questions are at the nexus of fields that have been historically separated from each other, “There are still fundamental questions at the interface of molecular biology, ecology, and evolution, and all of these areas can learn from each other.”
Needless to say, Werner is an advocate for integrative biology, both for its power to move science forward, and for its ability to make basic science relatable. It’s a reflection of these disparate but connected fields of study maturing, says Werner. A related concern is that in this era when basic science as a discipline is somewhat contested by the public, and even some governments and political figures, laser-focused myopia risks science losing the general interest of the public, and public funding. Paradoxically perhaps, inter-disciplinary studies not only bridge specialized fields but broaden the meanings, implications and perspectives for public consumption. Not to mention the applications basic science regularly funds through its model of open inquiry.
Meanwhile, Werner is settling into his new digs in the Aline W. Skaggs Biology Building. A native of Florida, he grew up straddling an ethnic divide (His mother is Cuban, his father Anglo) in which the play of nature vs. nurture was in high relief. As an undergraduate at Stetson University, a small liberal arts school, he toyed with a pre-med emphasis. But the fire for research would emerge later, during a nine-week stint doing an NSF-funded research experience undergraduate (REU) program at The University of Notre Dame. The experience fueled his desire to pursue graduate school in nearby Chicago where he met Talia, another new Assistant Professor in the School of Biological Sciences this year. After their PhDs the two of them found Germany and the Max Planck Institute to be “the perfect opportunity” to live abroad while each connecting to research programs that fit their intellectual curiosities.
Exploring environmentally-induced phenotypes in nematodes will continue to animate Michael Werner for the time being, as he always appreciated the idea that we are not genetically predetermined from birth, but rather have environmental influence over our lives. You can expect this forever biologist, fiercely committed to work that transcends academic silos, to look up regularly from his microscope at the broader contours of life and its ways and means, which is his ultimate, big-picture model system.