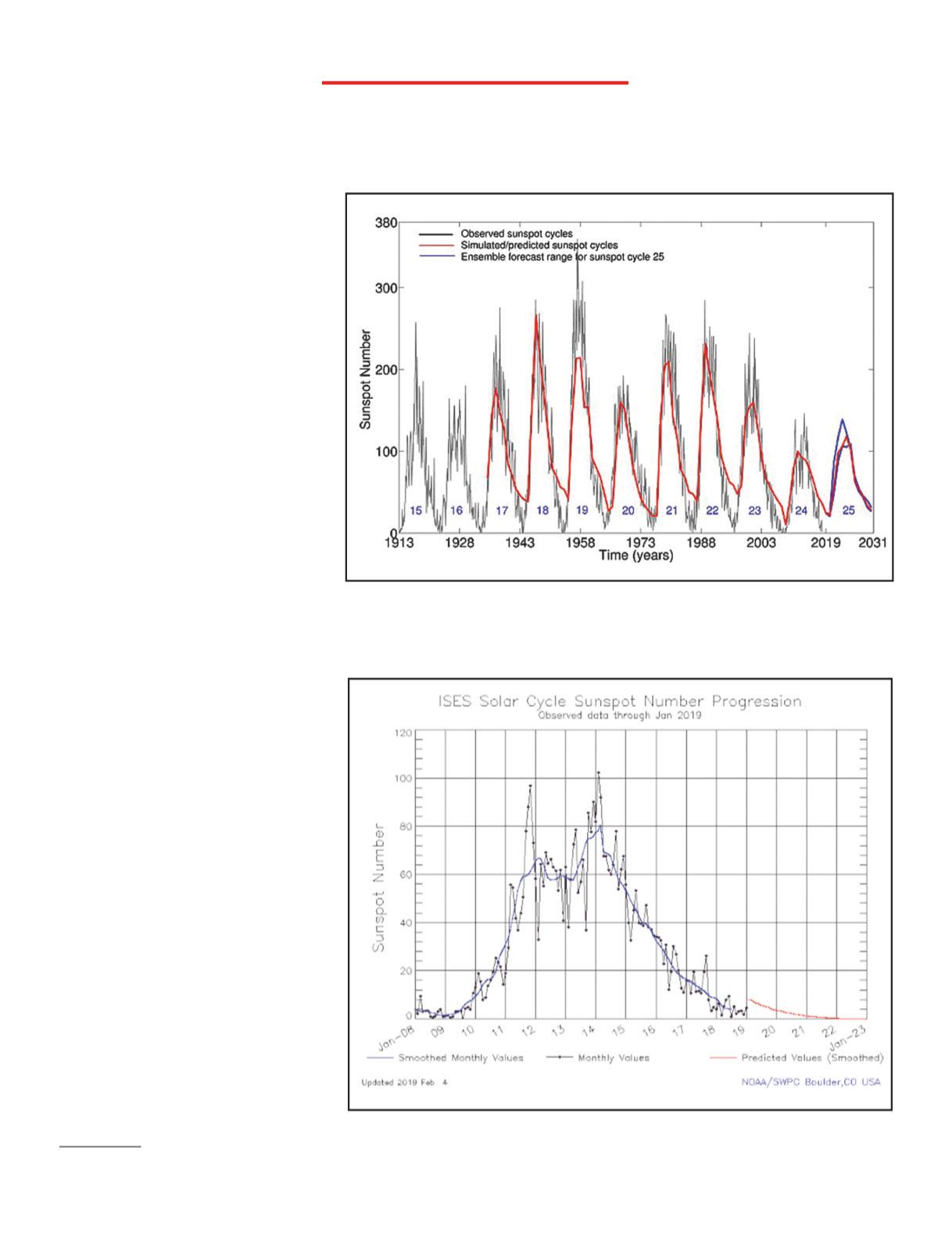
9 minute read
LEARNING C
BY RON OCHU, KOØZ
Space Weather andDX
Advertisement
Although this article is appearing in December, it is being written in October. There is a definite chill in the air and leaves are beginning to change colors. It doesn’t take much to imagine falling leaves soon being replaced with falling snow. Falling outside temperatures serve to remind me that winter is almost upon us in the northern hemisphere. Colder months and inclement weather translate into more indoor time for ham radio and for DXing (long distance communication).
High-frequency (HF) communication is influenced by several space weather factors. A great, one stop DX propagation website to view the influence of space weather on propagation is <https://dx.qsl.net/propagation> (orour own Propagation columnist’s website <www.sunspotwatch.com> –ed). Last month, we looked at great circle bearings and auroral impacts on HF propagation. This month, let’s look at a few more influencing factors.
Solar Cycle 25
You may recall solar cycles last about 11 years (Photo A). We are currently “ramping-up” to the solar maximum stage of Solar Cycle 25. By most accounts, Cycle 25 began in December 2019 and is expected to reach maximum in 2025 and minimum in 2030. Sometimes a solar maximum point turns out to be bimodal (Photo B), meaning that there are two successive high points. Solar maximum is defined as the greatest number of sunspots in each solar cycle (Photo C). Sunspots are temporary, cooler, dark spots in the sun’s photosphere. They are one indicator of the Sun’s activity, which in turn affects Earth’s ionosphere and HF radio propagation.
Solar Flares & Radio Blackouts
As our Sun becomes more active with sunspots, solar flares (PhotoD) tend to increase in both frequency and intensity. A solar flare is a burst of electromagnetic radiation speeding away from the Sun at the speed of light. An Earthdirected solar flare can reach the
*Email: <ko0z@cq-amateur-radio.com> Photo A. Agraphofsuccessive solar cycles. Asolar cycle is typically 11years long. We are currently in Solar Cycle 25. (All photos are public domain, unless noted)
PhotoB. RecentsolarcycleshavebimodalpeaksasseeninCycle24. Thepeaks result from the effects ofthe Sun’s northern andsouthern hemispherespeaking at different times. Will Cycle 25 have a bimodalpeak?
Photo C. Sunspots are agood indicator ofour Sun’s radiation activity. Numerous sunspots indicate more activity.
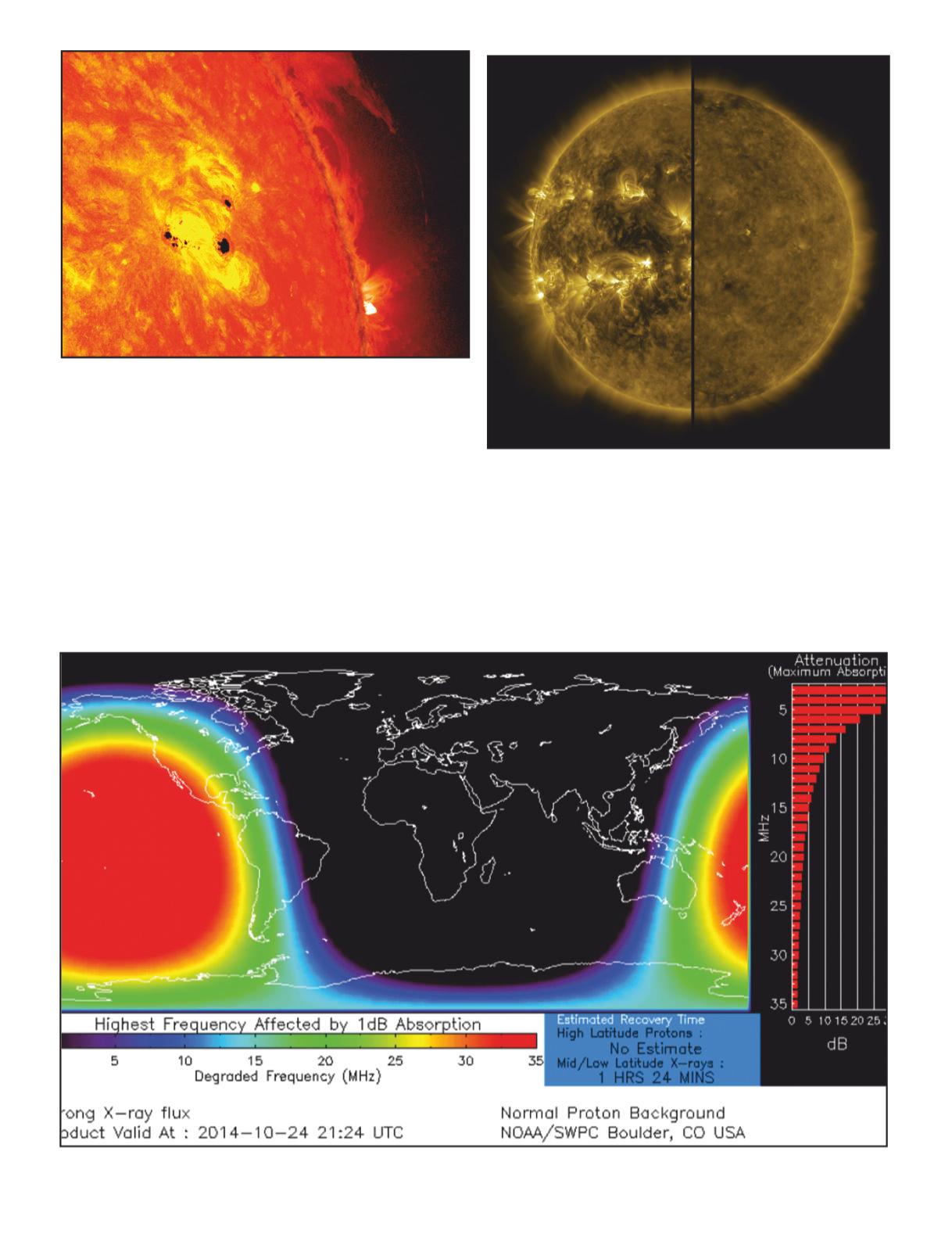
Earth’s atmosphere in just eight minutes. Depending on the radiation’s intensity, it can have a huge impact on Earth, disrupting HF communication and, in extreme cases, electric power grids. Solar flares are responsible for sudden ionospheric disturbances (SIDs) and radio blackouts (Photo E) in the sunlit region of Earth and these disturbances can last several minutes to several hours.
According to National Oceanic and Atmospheric Administration’s (NOAA’s) Space Weather Prediction Center (SWPC), radio blackouts are caused by bursts of X-ray and extreme ultraviolet radiation emitted from solar flares. Radio blackouts primarily affect HF (3-30 MHz) communication, although fading and diminished reception may spill over to Very High Frequency (VHF) (30-300 MHz) and higher frequencies. Someday, if you are having a 10-meter QSO (conversation) with someone and suddenly the band drops out and becomes quite noisy, then there’s a good chance you (and others) are experiencing a SID.
Photo D. Solar flares are evidentin the lefthalfofthephoto; the right half of the picture reveals a much quieter sun at solar minimum.
Table 1. NOAA’s RFBlackoutScale. RFblackouts tendto lastfor a few minutes to a few hours. (Tables courtesy National Oceanic and Atmospheric Administration)
PhotoF. Themostintensesolarflareeverrecordedoccurred on November 4, 2003. It was designated as an X28 flare. Fortunately, this solar flare was not Earth bound.
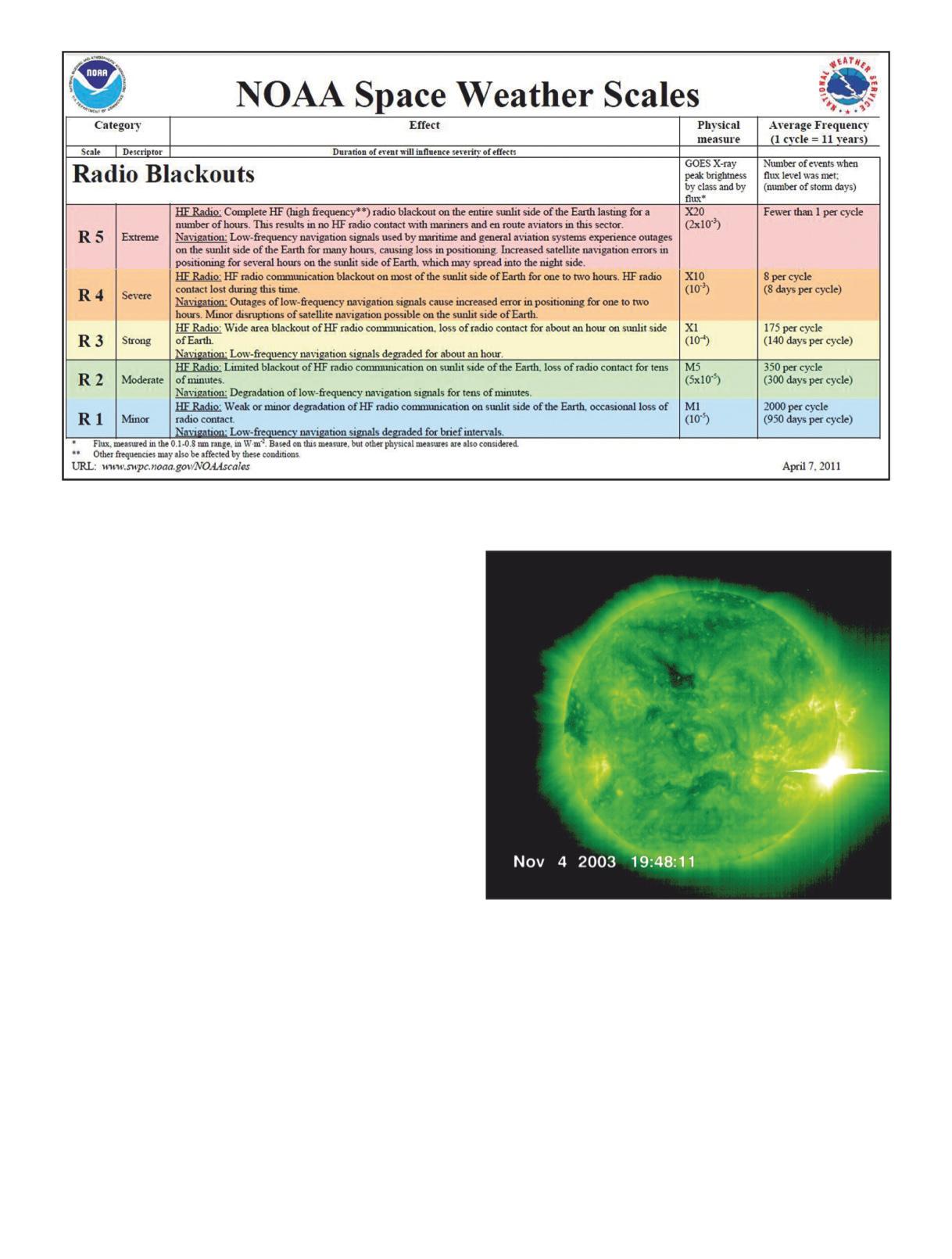
Solar flares are rated according to intensity, with “A” being the lowest and “X” the highest. On November 4, 2003, an X28 flare erupted from the Sun (Photo F) and is the most intense flare measured so far. NOAA also publishes a radio blackout scale ranging from R1 (minor) to R5 (extreme) (Table1). X-class solar flares are dramatic, but so are CMEs.
CME
Coronal mass ejections (CMEs) are solar explosions of plasma and magnetic particles (Photo G) that propel huge amounts of solar material away from the Sun into space, sometimes toward the Earth. Generally, it takes a few days for the material to travel the 92.6 million miles to reach Earth. When it does, our Earth’s magnetic field is affected.
According to NASA’s Goddard Space Flight Center, “Coronal mass ejections are more likely to have a significant effect on our activities than flares because they carry more material into a larger volume of interplanetary space, increasing the likelihood that they will interact with the Earth. While a flare alone produces high-energy particles near the Sun, some of which escape into interplanetary space, a CME drives a shockwave which can continuously produce energetic particles as it propagates through interplanetary space. When a CME reaches the Earth, its impact disturbs the Earth’s magnetosphere, setting off a geomagnetic storm. A CME typically takes 3 to 5 days to reach the Earth after it leaves the Sun; therefore observing the associated solar flare or the ejection of CMEs from the Sun provides an early warning of geomagnetic storms.
Geomagnetic Storms
Many of us regard geomagnetic storms as causing auroras (Photo H). That’s true, but are you aware that during geomagnetic storms, some radio frequencies are absorbed while others are reflected, leading to rapidly fluctuating signals and unexpected propagation paths (reproducing or multiplying in unplanned directions)? Public service radio and amateur radio can be disrupted. The SWPC defines a geomagnetic storm as “a major disturbance of Earth’s magnetosphere that occurs when there is a very efficient exchange of energy from the solar wind into the space environment surrounding Earth. These storms result from variations in the solar wind that produce major changes in the currents, plasmas, and fields in Earth’s magnetosphere. The solar wind conditions that are effective for creating geomagnetic storms are sustained (for several to many hours) periods of high-speed solar wind, and most importantly, a southward directed solar wind magnetic
field (opposite the direction of Earth’s field) at the dayside of the magnetosphere. This condition is effective for transferring energy from the solar wind into Earth’s magnetosphere. ”
“The largest storms that result from these conditions are associated with solar CMEs where a billion tons or so of plasma from the sun, with its embedded magnetic field, arrives at Earth. CMEs typically take several days to arrive at Earth, but have been observed, for some of the most intense storms, to arrive in as short as 18 hours. Another solar wind disturbance that creates con-
Photo G. If Earth directed, coronal mass ejections (CME) take a while to reach our planet. They can create geomagnetic storms andplay havoc with DXpropagation.
Table 2. NOAA’s Geomagnetic Storm Scale. Geomagnetic storms can havepropagation impacts thatcan last2to 8hours in the main phase. The recovery phase can last 8 hours to 7 days.
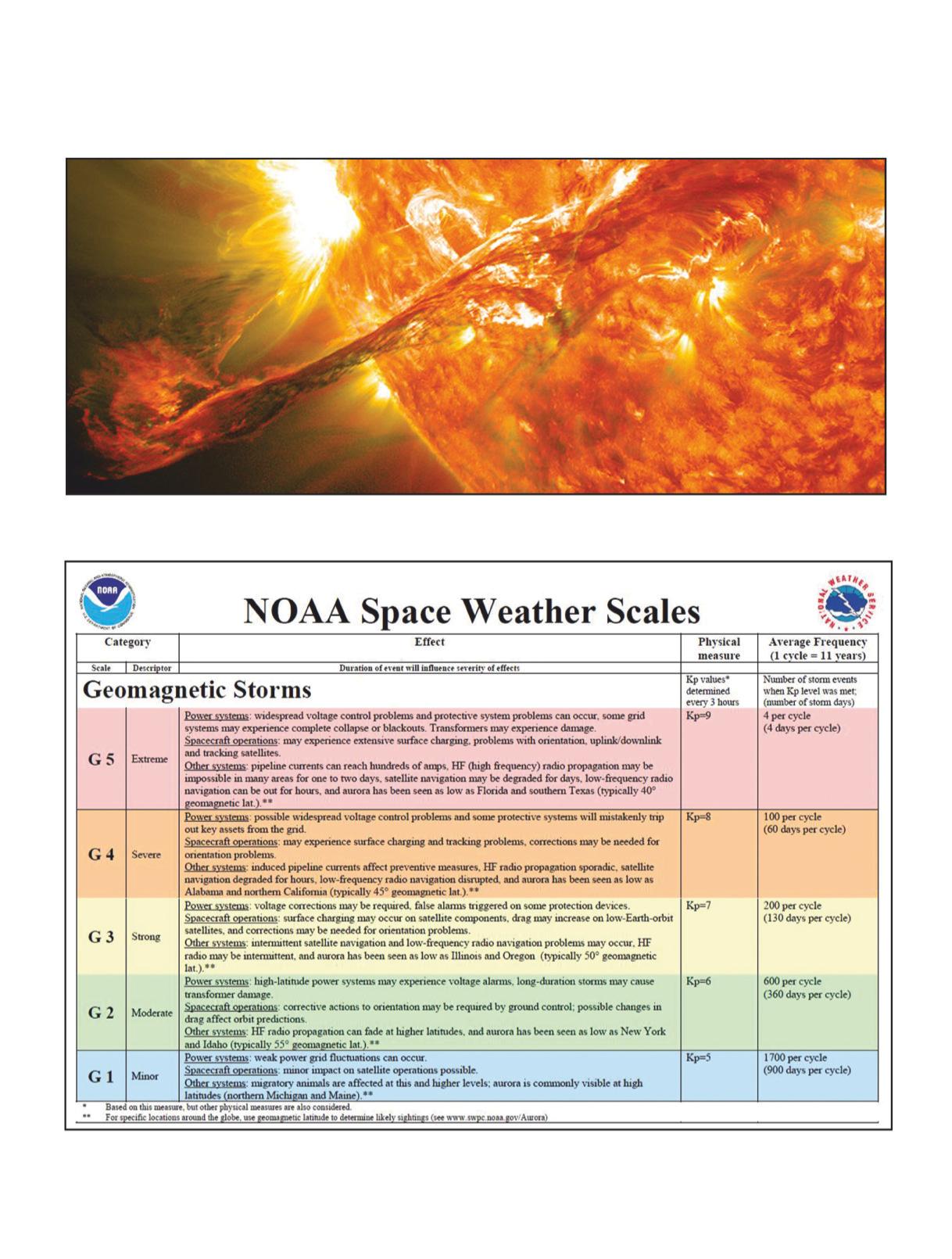
ditions favorable to geomagnetic storms is a high-speed solar wind stream (HSS). HSSs plow into the slower solar wind in front and create co-rotating interaction regions, or CIRs. These regions are often related to geomagnetic storms that, while less intense than CME storms, often can deposit more energy in Earth’s magnetosphere over a longer interval. ” NOAA
Photo H. Space weather interacts with Earth’s magnetic field, and it can create awe inspiring auroras.
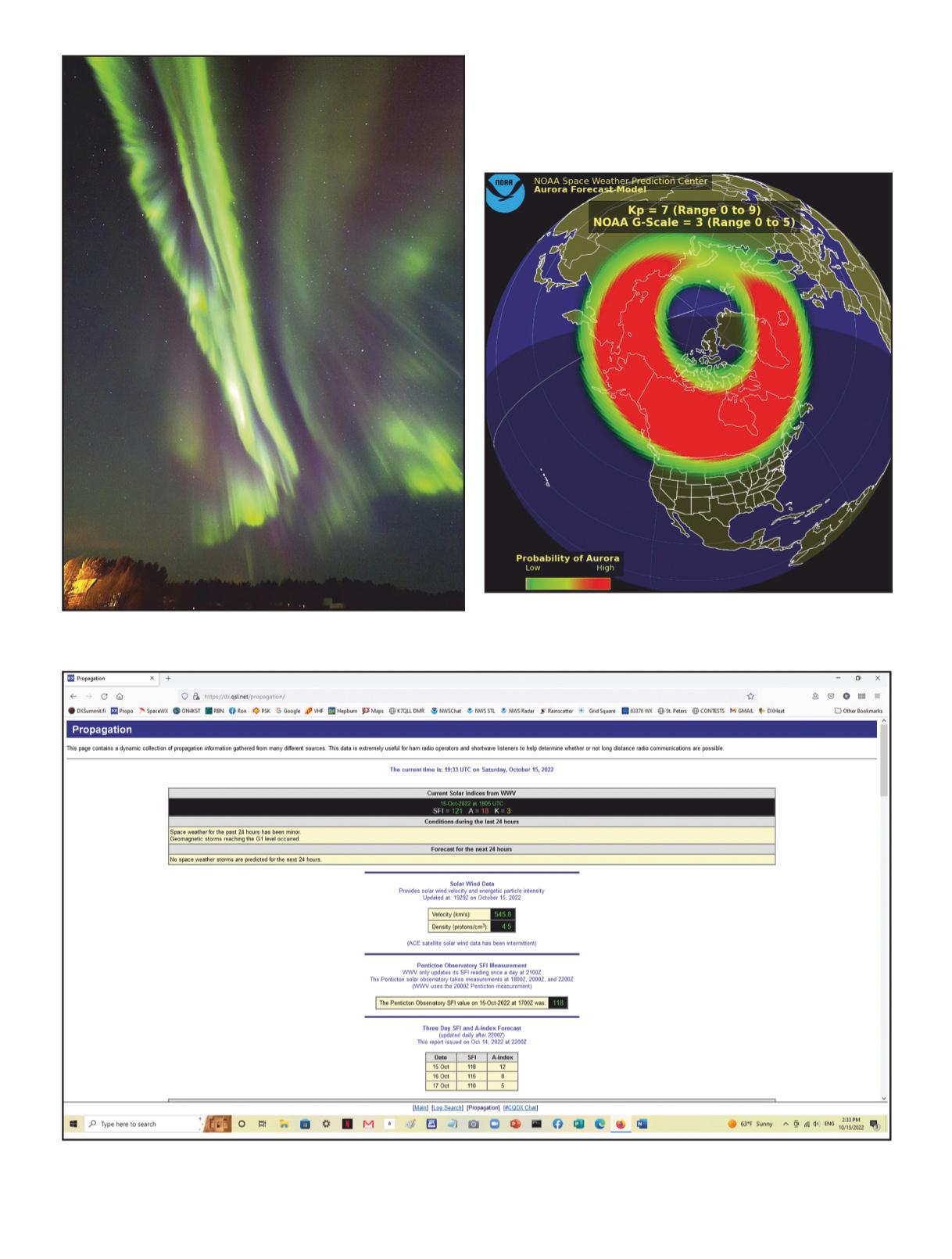
PhotoI. Anexampleofanauroralovalforecast. Whenitisred, DXpaths over the poles may be useless for HF, but for VHF signals, exciting aurorapropagation may be in the offing.
Photo J. DX Net’s propagation website offers a 24-hour space weather storm forecast in addition to many other DXpropagation aides.
Table 3. NOAA’s Solar Radiation Storm Scale. Solar radiation storms tend to last a few hours up to a few days.
publishes a geomagnetic storm scale ranging from G1 (minor) to G5 (extreme) (Table 2).
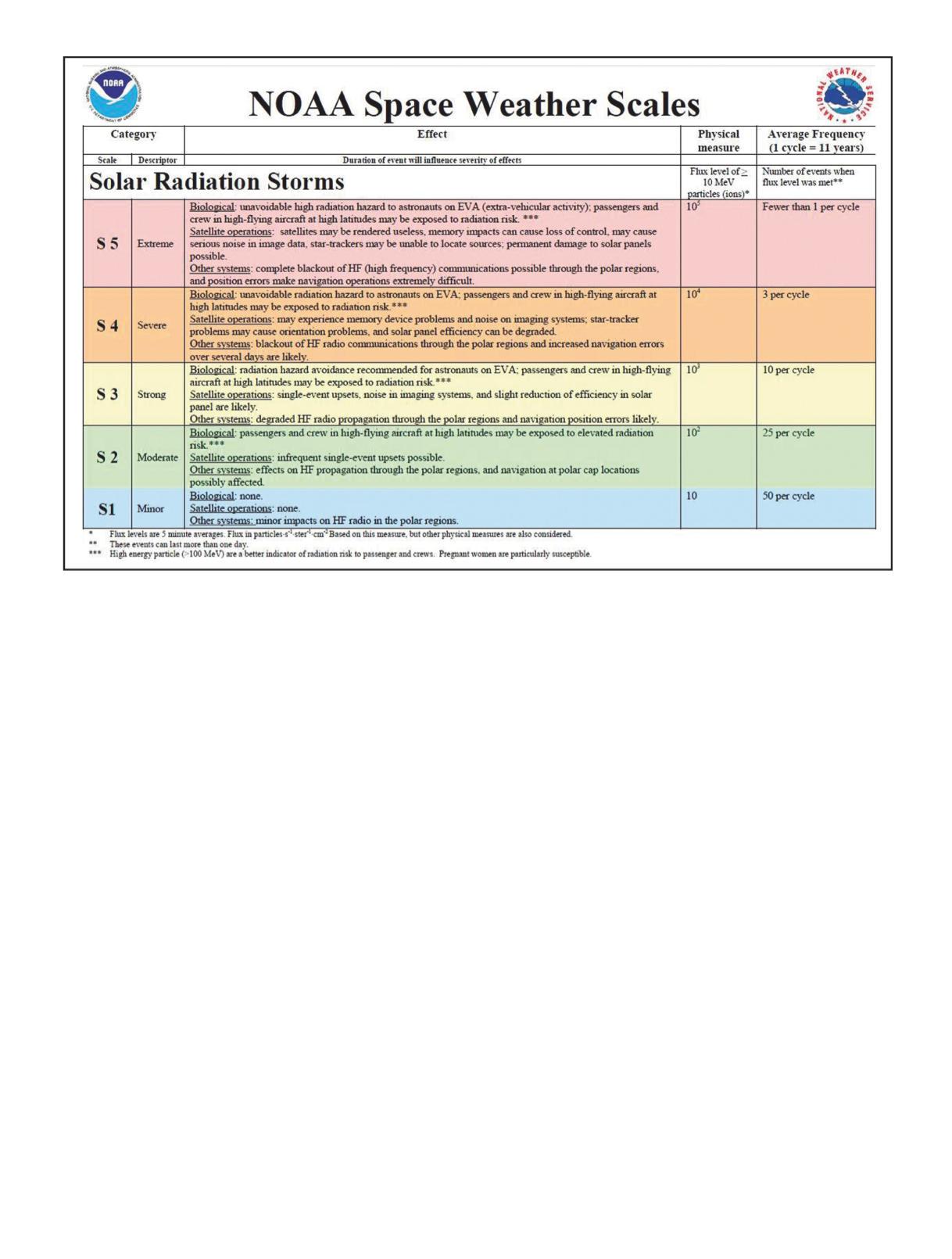
Solar Radiation Storms
Closely related to solar flares, radio blackouts, CMEs and geomagnetic storms is the solar radiation storm. The National Weather Service defines a solar radiation storm as, “when large quantities of charged particles, protons and electrons, are accelerated by processes at or near the Sun. When these processes occur, the near-Earth satellite environment is bathed with high-energy particles. ”
“Earth’s magnetic field and atmosphere offer some protection from this radiation, but the amount of protection is a function of altitude, latitude, and magnetic field strength. The polar regions are most affected by energetic particles because the magnetic field lines at the poles extend vertically downwards, allowing the particles to spiral down the field lines and penetrate into the atmosphere, increasing ionization. ”
“Energetic protons reach Earth a half hour to several hours after a solar eruption. Solar radiation storms can last from a few hours to days, depending on the magnitude of the eruption. Solar radiation storms can occur at any time during the solar cycle but tend to be most common around solar maximum. ”
“Solar radiation storm impacts include loss of HF radio communications through the polar regions, navigation position errors, elevated radiation exposure to astronauts, and to passengers and crew in aircraft at high altitudes and latitudes, and damage to satellite systems. ” Similar to the geomagnetic storms and RF blackout scales, NOAA publishes a Solar Radiation Storm Scale ranging from S1 (minor) to S5 (severe) (Table 3). DX Net’s propagation website, <https://dx.qsl.net/ propagation>, includes a space storm 24-hour forecast (Photo J).
There is a Bright Side
For sure, geomagnetic storms affecting HF propagation can be very annoying to radio amateurs. Simply put, propagation stinks during a storm. Fortunately, geomagnetic storms are temporary, and I’ve found that after the storm subsides, HF propagation can be enhanced. Whenever there is a strong geomagnetic storm and long-distance HF is rendered almost useless, I check for aurora propagation on the 6- or 2-meter bands. It’s not necessary to actually see an aurora in order to propagate VHF signals. Check out the auroral oval propagation on the DX Net website <https://dx.qsl.net/propagation>. When the oval is red near the northern U.S. border, there is a high probability the aurora will be able to support VHF propagation (Photo I).
If you haven’t experienced it, auroral propagation is exciting. Suddenly there is propagation on 10, 6, and even 2 meters. There is a buzz associated with this propagation. Voices sound distorted, almost void of tone. CW (Morse code) sounds more like a buzz. I remember an aurora opening about four years ago while living in central Illinois. I turned my 6-meter Yagi to the northeast and I was working stations in Canada, the U.S. East Coast, and everywhere in between.
The opening lasted for about two hours, and towards the end of opening, a relatively newly minted ham gave me a call on 6 meters. He lived about 30 miles south of my QTH (location). He called me because he was sure his new Kenwood transceiver wasn’t working properly on 6 meters. All of his received signals were distorted and he couldn’t quite tune them in correctly. I was the only station on the band who