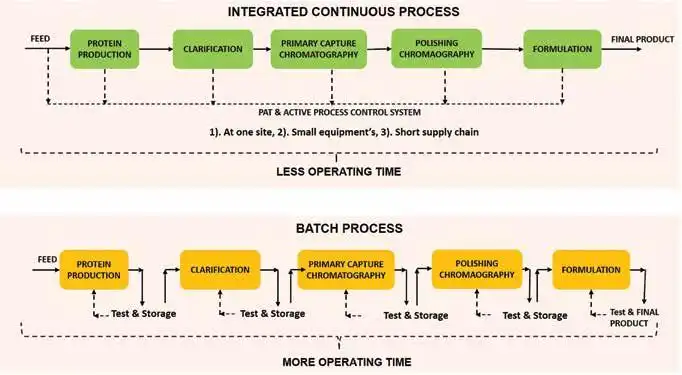
9 minute read
Integrated Continuous Biomanufacturing
Continuous manufacturing provides a new paradigm for biotherapeutics manufacturing. It has been shown to deliver high productivity (10-15X), lower cost of goods (by 50-75 per cent), and a more consistent product quality. This article highlights the key benefits of continuous processing for production of biotherapeutic products as well as the challenges that a practitioner is likely to face and solutions that are available today. It is emphasised that a centralised control platform for an integrated bioprocess is essential for real time data acquisition and on-line process control.
Anurag S Rathore, Professor, Department of Chemical Engineering, Indian Institute of Technology Nikita Saxena, Research Associate, Indian Institute of Technology
Batch manufacturing is the technology platform that is currently used for production of biopharmaceutical products. It involves step-by-step processing wherein one-unit operation must finish before commencement of the next operation. The mobility between the unit operations is slow as a result, affecting the overall productivity. In addition, the biopharmaceutical industry suffers from complexities related to scale-up, technology transfers, and batch to batch variability in product quality. This has prompted interest amongst the manufacturers to migrate from batch to continuous processing. Perhaps the biggest motivator is the significant difference in productivity. While the automation for the shift requires investment in the initial stage however the long-term profitability aspects are compelling. Studies have demonstrated a reduction of 50-75 per cent in the cost of manufacturing due to 10-15X increase in productivity which results in significantly reduced footprint of the manufacturing facility along with reduced capital cost as well as improved use of consumables. In addition, the cost involved with the transportation of batches to lab for analysis is also reduced (Jungbauer & Hammerschmidt, 2017). In the pharmaceutical industry, shifting to continuous manufacturing saved operating cost by 9 per cent to 44 per cent, capital expenditures by 20 per cent to 76 per cent and foot print reduction by 40 per cent to 90 per cent (Spencer et al. 2011). Improved capacity utilisation of the production chain in achieving demand and supply requirements, improved implementation of complex recipes, elimination of transition times anda more consistent upkeep of the schedules are some of the advantages that continuous processing offers over batch manufacturing (Rathore et al. 2015) (Figure 1). In addition, the development of soft sensors (Figure 2) and process analytical tools (PAT) are likely to further enhance control and consistency of product quality (Thakur et al. 2019, Zobel et al. 2017, Hebbi et al. 2019). Further, process automation reduces the need for manual interventions, thereby resulting in fewer human errors and minimum system disturbance. While the benefits of continuous processing are quite significant, the transition from batch to continuous is technically quite complex.
The concept of Quality by design (QbD) is quite aligned to what continuous manufacturing offers with respect to modernisation of production and improvement of consistency in product quality (Rathore & Winkle 2009). The systematic, science and risk-based methodology that is adopted towards experimentation and knowledge generation ensures that the manufacturer utilizes robust control strategies. For a manufacturer to successfully run a continuous process, this knowledge base is essential
Comparison of batch and continuous manufacturing
Figure 1
so as to maintain the process within its design space and ensure that the critical quality attributes (CQA) are consistently maintained (Rathore et al. 2018).
Challenges in implementing continuous manufacturing
Creating a continuous process platform is not merely connecting different unit operations in series. The manufacturing system consists of a number of parts whose assembling and calibration is an extensively time-consuming process. At times, suitable technologies may be required to integrate unit operations for improving the process efficiency. For example, it is becoming common practice to integrate the bioreactor with a cell retention device followed by continuous capture chromatography. Such an approach has been successfully demonstrated for high volume production of a monoclonal antibody (mAb) product as well as a low volume, less stable protein (rhEnzyme) (Warikoo et al. 2012). A recently reported invention is that of a continuous flow inverted reactor (CFIR)
which allows for performing any reactionbased unit operation (such as protein refolding and protein precipitation) in a continuous format (Kateja et al. 2016, Sharma et al. 2016).
The most significant challenge that a user faces in designing a continuous process is that of process control. Batch processing allows the user to take a sample at any process point and do the required analysis before initiating the next process step. Continuous process does not give this opportunity to the user and as a result if a robust control regimen is not put in place, continuous production cannot be realised. For an end to end integrated continuous manufacturing platform, developing an architecture is a challenge (Konstantin & Cooney 2015, Kateja et al. 2017, Kateja et al. 2018). One such example of an integrated architecture is use of a perfusion process in bioreactor combining alternating tangential flow systems (ATF), followed by two four column periodic counter current chromatography for capture, viral inactivation and two polishing steps (Godawat et al. Illustration of soft sensor technology for control purpose
Figure 2
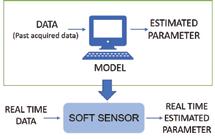
2015). This platform has been shown to offer better resin, buffer and equipment utilisation compared to the traditional processes. However, designing such a platform requires in-depth understanding of the process, the process equipment, as well as that of the product and its attributes. In addition, product recalls in batch manufacturing are easier than in continuous manufacturing as back tracing is relatively straightforward. This creates a need to maintain a quality control system that should be meticulously designed to
Multi level control system – a potential solution for the control related issues. Level 1 & 2 represent the greater process understanding and tight control over process parameters whereas lower level in the pyramid requires more regulatory oversights
Figure 3
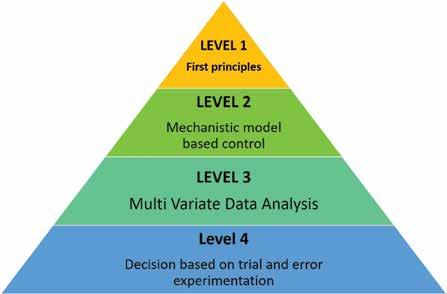
minimise the odds. Significant amount of work is required to transform the concept of continuous processing into systematic industrial strategy.
Potential solution
One of the promising solutions is to develop a robust integrated bioprocess that is operated by a Distributed Control System (DCS) that can be single layer or multilayer control (Figure 3). A centralised platform for the automation of the bioprocess improves real time data acquisition and on-line process control. For example, a computer implemented process control method has been adopted to control continuous production of biopharmaceuticals using proportional integral and derivative controller along with the fuzzy controllers (Patent EP3294856B1). Incorporation of surge tank/break tank increases the sampling points for the inline monitoring of the process parameters. This improves the operational feedback control strategy of the unit operations which includes clarification, chromatography, ultrafiltration, and depth filtration (Patent, US20140255994A1). Commonly, PAT tools that operate in real-time, such as spectroscopic techniques (UV, NIR, Raman) are ideal for performing real time process control. As stated before, the biggest challenge for continuous manufacturing is developing a flexible control system. The centralised control can take the various operating parameters as inputs for the different unit operations, such as flow rates, cycle times, pause times, and cleaning schedules to carry out the normal operation of the continuous train. A python layer with the userfriendly interface can be used for dynamic implementation of different recipes for individual unit operations depending on the task (normal steady-state operation/ deviated operation), molecules (different mAb/protein molecule characteristics) and feed conditions (high/low titer, high/low impurities/aggregates/charge variants). It also assists in dynamic process scheduling wherein intelligent decisions can be enforced. For instance, using pressure/ turbidity sensor for scheduling cleaning cycles and filter switching (Thakur et al. 2020), using pressure sensor for scheduling chromatography column replacement, and using NIR for scheduling loading decisions in Protein A chromatography (Thakur et al. 2019). In addition, prediction made by model-based control can be used to check against real time operation of the process. For instance, product CQAs can be monitored during the bioreactor operation using Raman/ NIR and customised adjustments in the feed and feeding rate can be designed in perfusion systems. Three-way data flow between the model, unit operation and the PAT tool is helpful for achieving optimised performance for the wide range of operating conditions. In case of sufficient data, rule-based models can be implemented which are based on the experimental thresholds and provided different control logic based on the real time status of the different process parameters. However, due to the complexity of the unit operation, hybrid models (combination of empirical model and mechanistic model) for real time control are preferred over others. A provision can be made in the DCS to select the model from the stored library/historian in the real time.
The increased necessity in enhancing the process efficiency, productivity and the product quality has significantly increased interest in Statistical Process Control (SPC).It works on the theory of variation that combines time series analysis with the variable data history and aims to provide insight for a high level of performance. It has been seen that the control loops considered based on the empirical or mechanistic models are not enough to maintain the product quality in case of process uncertainties (Kroll et al. 2017). An effective online statistical process control strategy should be developed to handle the correlations between the input and output variables which are directly or indirectly affecting the product quality. The concept shifts
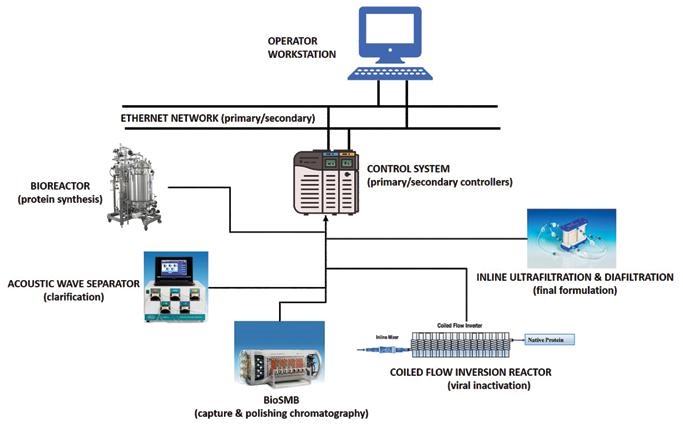
A hypothetical centralized control system for a continuous process. Data flows from equipment to control system unit to operator workstation to unit operation equipment’s via IO’s and ethernet network
the focus from a fault detection-based strategy to a fault prevention-based strategy. Real time monitoring of the variables helps the operator to track the changes in the trend before the effect manifests itself in the product. Statistical tools like control charts, histograms, capability index can predict process variability and contribute to quality control. The approach can be implemented with the help of a centralised system where the data can be acquired and stored. Using the data historian large summation of the errors can be avoided and hypothesis can be formed related to the changes in the variables.
Future perspective on continuous bioprocessing
The increasing interest in commercialisation of continuous manufacturing necessitates that the technical challenges that arise need to be overcome. Despite the benefits of continuous processing, adoption is at a slow pace. Key concerns of the manufacturers include the risk of contamination and challenges associated with process control and increased operational complexity. We are confident that the biopharmaceutical industry will gradually adopt continuous processing due to the significant benefits it offers with respect to increased productivity, reduced cost of manufacturing and improved consistency in product quality. Innovations in approaches taken for control of continuous processes will continue to be in spotlight. References are available at www.pharmafocusasia.com
AUTHOR BIO
Anurag S Rathore is a Professor at the Department of Chemical Engineering, Indian Institute of Technology, Delhi, India. He is also the Coordinator for the DBT COE for Biopharmaceutical Technology. Prior to joining IIT, he held management positions at Amgen Inc., Thousand Oaks, California, and Pfizer Corp., St. Louis, Missouri. Nikita Saxena is Research Associate at Indian Institute of Technology Delhi. She is an expert in process control. She is presently working on a major project that involves automation and digitisation of biopharma processes for continuous production of biopharmaceuticals.