Navigating Climate Challenges
CASE STUDIES IN WATER SECTOR ADAPTATION
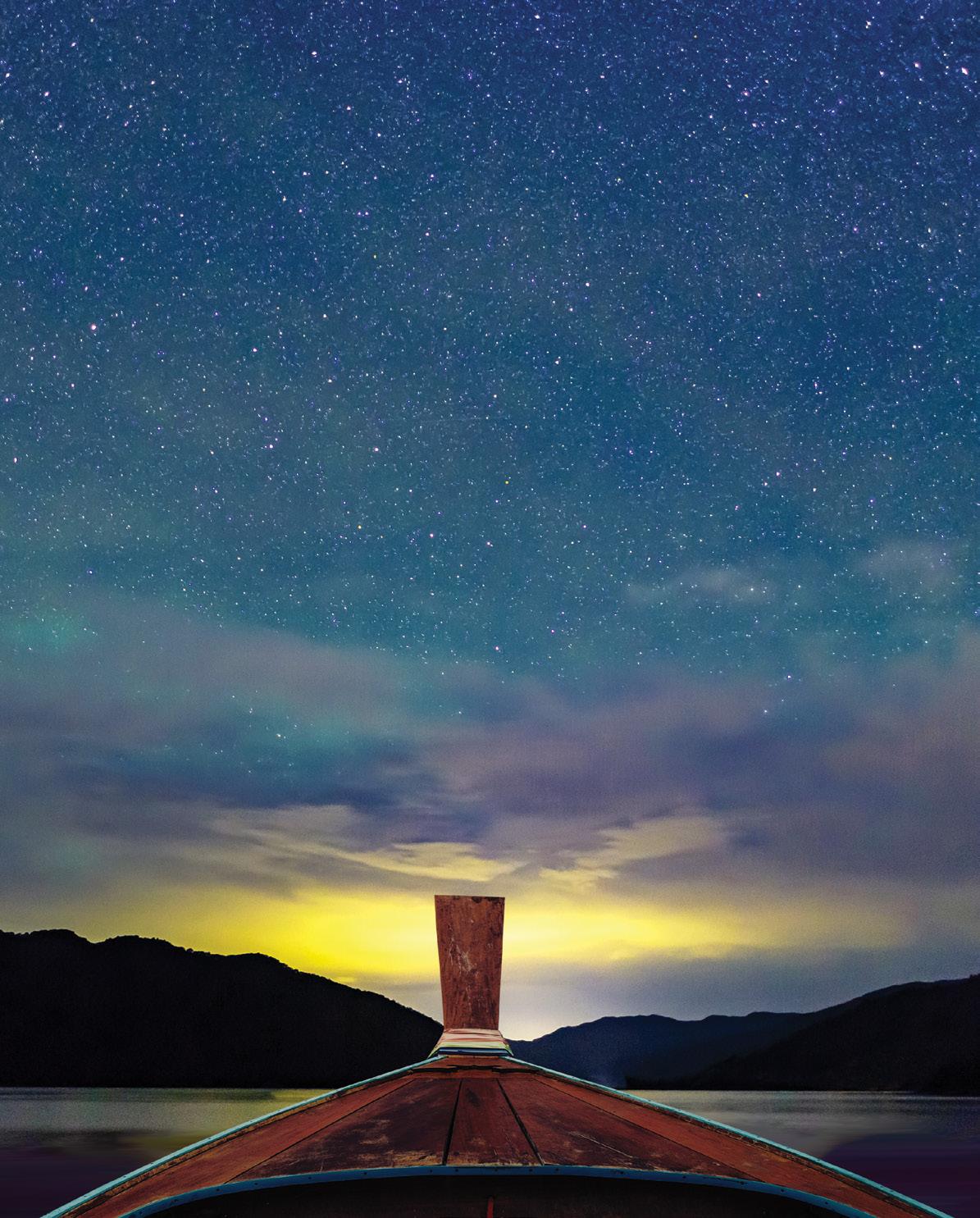
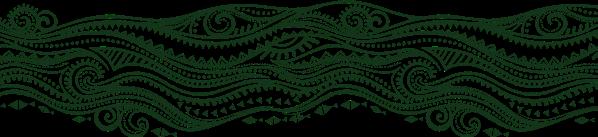
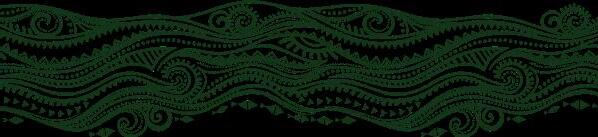
This document is an initiative of the Water New Zealand Climate Change Special Interest Group. Hana Kashkari of Mott MacDonald led its development with input from adaptation group members Matt Balkham of Jacobs, Chris Cameron of PDP and Joyce Thomas of Central Otago District Council. The document has been developed by authors contributing their time pro-bono. Our thanks for their commitment to advances in water sector climate adaptation in New Zealand. Lesley Smith of Water New Zealand has co-ordinated its development.
We are also grateful to the case study providers who generously shared their time and expertise: Liam Wotherspoon (University of Auckland), Stefania Mattea and Nicky McDonald (Market Economics), Judy Lawrence (Victoria University of Wellington), Geoff Williams (Wellington Water), Sharon Danks (Watercare), Mott MacDonald, Jacobs and PDP.
Reproduction, adaptation or issuing of this publication for educational or other non-commercial purposes is authorised without prior permission of Water New Zealand. Reproduction, adaptation or issuing of this publication for resale or other commercial purposes is prohibited without the prior permission of Water New Zealand. Permission can be sought by contacting Water New Zealand at enquiries@waternz.org.nz
Water New Zealand and individual contributors make no representations and give no warranties of any kind, whether expressed or implied, concerning the information contained in this document. The document and all information contained in it are provided “as is” and subject to change without notice. Any risk arising out of its use remains with the recipient. Water New Zealand and individual contributors are not responsible for the results of any actions taken on the basis of information in this guideline or any loss, damage, costs or expenses of any kind that may arise in any way out of, or result from, any use of material or information in this guideline.
Published by
Water New Zealand | PO Box 1316, Wellington 6140 | P: +64 4 472 8925 | E:enquiries@waternz.org.nz | W: www.waternz.org.nz
Publication date: 9 / 2024
ISBN: 978-1-0670357-2-3
Our communities face ever-increasing pressure from climate change impacts. Despite admirable attempts to reduce carbon emissions across the globe, the World Meteorological Organization predicted in June 2024 that annual average global temperatures have an 80% likelihood of temporarily exceeding 1.5°C in the next five years. Reduction of greenhouse gas emissions remains slow and is undermined from many quarters. So, we are faced with the need to protect our communities, and change the way we live and work, to accommodate the changing climate.
In New Zealand, increases in severe weather events have been all too familiar in recent years, with devastating flood events across the country, claiming lives and livelihoods and causing far-reaching disruption to many communities. Although we have limited ability to stop these events from occurring, the infrastructure that we plan, design, construct, operate and maintain has a significant role to play in protecting our communities. The water sector, in particular, has an important role. Clean drinking water, sewage conveyance and treatment and catchment management, are lifeline services for communities and need to be either unaffected by disaster or be back up and running quickly to avoid disease and other threats to life.
Water New Zealand’s Climate Change Special Interest Group has spent recent years identifying the needs of water sector professionals, and gaps in knowledge, with the goal of ensuring that the best and most up-to-date information is available to those making long-term decisions for resilient infrastructure in our communities. The result is this technical publication aimed at showcasing current best practice and advanced thinking in the many layers of resilient infrastructure delivery, from the strategic to the asset level.
The hope is that this document can evolve over time, becoming a repository of growing knowledge to catapult infrastructure in New Zealand into a resilient future.
Nick Dempsey
Technical Director – Water, Mott MacDonald
Chair of Water New Zealand’s Climate Change Special Interest Group
This document aims to help the New Zealand water sector address climate adaptation challenges, providing examples of effective local and global solutions. It focuses on filling knowledge gaps identified by Water New Zealand (WaterNZ) members, and features case studies that highlight successful climate adaptation solutions.
A large proportion of New Zealand’s water infrastructure is managed by local councils, which already face competing priorities and infrastructure deficits that will be exacerbated by climate change. For achievable and effective adaptation to occur, it is essential to share experiences – successes and failures.
Departing from the conventional three-waters framework used in New Zealand, this document focuses on adaptation at the organisational strategy/governance, portfolio and asset levels. The aim is to foster interconnected thinking across all three water disciplines, the water cycle and all facets of water management. Each of the three waters face similar challenges in adapting New Zealand’s aging infrastructure and water professionals must avoid thinking in silos and seek to learn from one another
For example, adapting only at the asset level might address short-term issues for a particular infrastructure network, but is unlikely to help with the long-term strategic issues faced by multiple networks. Indeed, such asset-level responses could result in sunk investments that might not be recouped, or maladaptation.
Effective adaptation requires collaboration across network operators, alongside iwi and local and central government agencies.
Figure 1: Implementation levels for climate adaptation
Focused on the wider New Zealand water industry strategy and governance e.g. reulation, strategies, policies, industry guidence, decision making processes.
Focused on a portfolio of assests e.g. Wellington’s watewater network, or a region’s interconnected network of blue-green and grey stormwater infrastructure.
Focused on a specific asset e.g. optioneering, design and operational plans for a water treatment plant.
Through this collaborative approach, we can collectively address the challenges of upgrading and maintaining New Zealand’s water infrastructure. The case studies included here draw on local and international examples in the water sector.
Rather than being read from start to finish, it is envisaged that readers will dip in and out of the contents to access information most relevant to their circumstances.
1 New Zealand’s Country Profile – Climate Action Tracker (Climate Analytics and NewClimate Institute, 2022) Found at: https://climateactiontracker.org/
2 Yang, D., Yang, Y., & Xia, J. (2021). Hydrological cycle and water resources in a changing world: A review. Geography and Sustainability, 2(2), 115-122.
Our climate is changing. Global policies and actions are putting us on track for a world nearly three degrees warmer than today by the turn of the century1. This will have significant impacts on the hydrological cycle2 before many of our existing water assets have reached the end of their functional lives. Today’s infrastructure is shaped by decisions made generations ago. Even if greenhouse gas emissions were stopped today, we would need to implement climate resilience measures for decades to come to cope with the climatic changes already locked in3
Existing infrastructure systems have been designed based on the past climate, which will not always be adequate to cope with the increasingly extreme events of the future. If we are not prepared for natural disasters, cascading failures can occur across interconnected infrastructure services. Such failures can have significant impacts on people’s lives and asset owners’ ability to provide services.
In addition to climate change impacts and the need to adapt, our infrastructure is ageing, with increasing pressure to address leaks, contamination and upgrades that are well overdue. A further factor is urban intensification, requiring additional water services that can strain existing systems.
New Zealand has a record of historic under-investment in infrastructure, with current estimates for the investment needed before 2050 ranging from $120 billion to $185b4
These challenges are being felt now. In 2023, the extreme weather events during the Auckland Anniversary storm and Cyclone Gabrielle provided New Zealanders with a clear example of the devastating impacts of extreme weather on our water services and infrastructure. Drinking water networks were disrupted and wastewater treatment and stormwater management systems were overwhelmed. Fifteen people died and the lives of many more were forever changed5. Between $9b and $14.5b of physical damage was caused to households, businesses and infrastructure6
As weather extremes increase, climate change threatens the fabric of our society and it is marginalised communities that will bear the brunt of these impacts. They have the least economic means to adapt, and the least resources to participate in adaptation decisions. Māori have deep connections to land and a resulting reliance on natural resources adds particular vulnerability.
It is imperative that steps are taken now to embed climate adaptation into equitable decisions about our water infrastructure. Centring the voices of Māori and others most vulnerable to impacts, building strong partnerships and investing in capacity building will be essential to ensure water services can function for all into the future.
When investment in maintaining, upgrading or adapting infrastructure is delayed, the costs and challenges of coping get pushed out to future generations and increase. Delaying investment also means increased maintenance costs. It is estimated that for every $1 spent on adaptation now, between $2 and $10 are saved7 in the long term.
3 Web Article: Insight: The time is now to build for the future (New Zealand Climate Commission, accessed July 2024 ) Found at: https://www.climatecommission.govt. nz/news/insight-the-time-is-now-to-build-for-the-future/
4 Economic analysis of water service aggregation: final report (Water Industry Commission for Scotland, 2021) Found at: https://wics.scot/system/files/2022-02/ Three%20Waters%20reform%20-%20Economic%20analysis%20of%20water%20services%20aggregation.pdf
5 Final report of the Government Inquiry into the Response to the North Island Severe Weather Events (New Zealand Minister for Emergency Management and Recovery, 2024) Found at: https://www.dia.govt.nz/Government-Inquiry-into-the-Response-to-the-North-Island-Severe-Weather-Events
6 Impacts from the North Island weather events (The Treasury, 2023) Found at: Impacts from the North Island weather events – Information release –27 April 2023 (treasury.govt.nz)
7 Global Commission on Adaptation (2019) Adapt Now: a global call for leadership on climate resilience. Found at: Adapt Now: a global call for leadership on climate resilience - Global Center on Adaptation (gca.org)
Climate change will affect the hydrological cycle in Aotearoa in many ways, including:
• Reduced snowpack formed over winter
• Increased glacial melt over summer
• More intense storm events
• Increased high-intensity rainfall events
• Sea level rise on the coasts impacting ground water aquifers
• More droughts
• Increased ambient temperatures and water temperatures
• Increased evaporation.
Modelling climate impact on the water cycle is complex. Because of this, such impacts are, for the most part, researched and modelled in different ways. However, climate projections for New Zealand systems are available for estimating the changed climate in: high-intensity rainfall, fluvial flooding, pluvial flooding, river flow projections, sea level rise, coastal flooding and groundwater. This factsheet from the Deep South Climate Challenge will help you access sources of data: https://deepsouthchallenge.co.nz/resource/infosheet-water-data/
In addition to changes in the hydrological cycle, climate change will affect water, wastewater and stormwater infrastructure in myriad other ways. Water sources will alter in availability, changing the way we need to manage drinking water networks. Existing infrastructure will face increased risk of damage, failure or insufficiency. These changes will exacerbate existing costs and social pressures, presenting a significant strategic challenge for the water industry.
This paper does not attempt to detail the many risks climate change poses to water service delivery. This work has been undertaken by others. Key climate impacts, used as the basis for a 2022 workshop held by the Water New Zealand Climate Change Special Interest Group8, are outlined in Table 1.
8 Tonkin and Taylor (2022) Water services climate risks and adaptation workshop summary. Found at: https://www.waternz.org.nz/Article?Action=View&Article_id=2383
For a comprehensive overview of climate change effects on core functions of water service delivery refer to the Water Services Association of Australasia Climate Adaptation Guidelines https://www.wsaa.asn.au/publication/climate-change-adaptation-guidelines
For further information on impacts of climate change on stormwater and wastewater systems in New Zealand refer to https://deepsouthchallenge.co.nz/research-project/stormwater-wastewater-and-climate-change/
• Increased flooding of buildings and assets arising from higher peak flows, inundation from sea level rise and raised groundwater levels
• Loss of land (including from landslides) and damage to infrastructure arising from storm damage, temperature extremes, increased peak flows, asset failure, salinity exposure, groundwater level changes causing settlement and flotation and reduced flushing flows causing sedimentation.
• Deterioration of water quality arising from scour, bank/berm erosion, resuspension of historical sediment, altered performance of water quality treatment devices, increased evaporation and dry out of vegetated systems, raised water temperatures and increased salinity exposure.
• Nuisance flooding, spills and odour arising from increased temperatures, changing user behaviour resulting in higher concentration flows and blockages and waterlogging of on-site wastewater treatment plants.
• Water quality deterioration arising from increased uncontrolled wastewater discharges, increased infiltration, increased salinity of wastewater affecting the performance of wastewater treatment plants (WWTPs), behaviour changes resulting in higher concentration flows, which may result in a deterioration in the quality of treated wastewater discharges.
• Damage to infrastructure arising from storm damage, overheating and increased wear of pumping stations, waterlogging, soil structure and ecological changes affecting the performance of onsite systems, temporary or permanent inundation, groundwater fluctuation causing ground settlement or flotation, and changing user behaviour causing increased concentration of wastewater leading to corrosion, blockages and increased maintenance at the WWTPs.
• Reduced asset life or increased operational complexity arising from extreme weather. For example, extreme rainfall may lead to larger and faster inflows with increased sediment loads, increasing temperature may change the nature of biological and chemical reactions during treatment, and increasing temperatures may decrease the quality of raw water inflows to water treatment plants by contributing to algal blooms.
• Limitations on water availability may arise due to drought and increasing frequency and length of peak demand periods during very hot days and extended dry periods.
• Reduced disinfection residuals in water distribution due to increasing temperature.
• Relocation or adaptation of assets may be required due to exposure to increasing coastal or fluvial flooding.
To inform the development of this guideline, challenges faced by the New Zealand water sector were collated from a WaterNZ survey of water professionals and brainstorming sessions with members of the Water New Zealand Climate Change Special Interest Group. Responses have been compiled into Governance/Strategy, Portfolio and Asset levels and are shown in Table 2.
Table 2: Top challenges faced by the New Zealand water sector
Industry strategy and governance level challenges
Portfolio level challenges
• Need for industry/governance guidance on wider risk & resilience to the NZ Water Sector from climate change.
• Unclear accountability pathways and regulation of them i.e. national to regional to local to asset owner
• Lack of staged investment planning for adaptation i.e. investing in adapting as needed throughout a portfolio and updating investment planning as we learn more about how climate will impact assets.
• Current modelling standards, design codes, disaster risk management, policies & planning requirements either do not or inconsistently include climate considerations.
• Difficulties associated with deciding how to prioritise multiple risks e.g. climate change, volcanic, earthquake, flood, coastal erosion etc.
• Challenges around communicating risk to the public due to the complex technical nature of multi-criteria risk assessments & high cost associated with asset construction & upgrades.
• Lack of understanding of risk across portfolios and connecting networks of assets.
• Lack of strategic planning at systems level for improved resilience.
• Cascading risks from interdependent assets not being included within considerations.
Asset level challenges
• Need to integrate climate resilience into optioneering, asset designs and operational plans.
• Difficulties associated with adapting existing assets to be more resilient at a low cost, given brownfield and material availability constraints.
• Need to assess risks & resilience considerations specific to individual assets.
• Need to embed resilience throughout an asset’s lifecycle.
This compilation of case studies provides examples of approaches being applied to known complex challenges. Such examples will increase as our knowledge of how to address them grows and as new challenges emerge. Many of the challenges faced by water infrastructure are global. By growing and maintaining strong international partnerships we have an opportunity to learn from solutions being trialled around the world.
Some things make Aotearoa New Zealand unique. Māori are experts in adaptation, having voyaged across the Pacific and survived colonisation. Tapping into the growing revitalisation of mātauranga Māori (Māori knowledge) offers an opportunity to introduce relevant place-based solutions. It can also support us to frame the adaptation challenge using a wider view, breaking away from siloed western constructs that tend to focus on climate change in isolation from environmental and social factors. This requires all of us in the water sector to deepen our understanding of New Zealand’s foundational document, Te Tiriti o Waitangi, te ao Māori and partnerships with mana whenua.
Reflecting that our body of knowledge will grow over time as our maturity and knowledge as a sector grows, this publication is intended to be a living document. Submission of additional case studies for future revisions via its Climate Change Special Interest Group. Group information and relevant contacts are available at: www.waternz.org.nz/climatechange
The tuatara has proved itself a climate adaptation champion, surviving for over 200 million years since the time of the dinosaurs. It has been adopted as the motif for this document, inspired by its resilience and ability to survive dramatic environmental changes. Whether their species can survive into the future will depend on steps taken today, as warmer temperature influence the tuataras reproduction. Efforts to conserve tuatara and protect their habitats mirror the broader fight against climate change and biodiversity loss, making them a symbol of the need for climate action.
Porirua City is already experiencing the impacts of climate change. In the past, Porirua City Council’s response to severe weather events has been largely reactive. This approach has come at a significant cost to council with more than $30 million spent on clean-ups after floods, slips and other storm damage in the past 10 years.
This costly and inequitable approach is similar to that taken by many councils in New Zealand. There is a better way – adaptation planning enables us to consider overall risk to the network of council assets and how we can change our management approach over time so ratepayers’ money is not diverted to the response.
Porirua City has adopted a three-phase programme to proactively respond to climate change and is currently working through Phase 1.
• Phase 1 involves working with asset managers to understand the exposure and vulnerability of council assets. This relies on existing information prepared for the district plan review and excludes preparation of any new technical information. There are five key steps:
1. Internal engagement with asset managers about assets at risk
2. Identifying any gaps in the datasets
3. Collating all asset and risk information into one place
4. Identification of possible options including a cost-benefit analysis
5. Reporting and recommendations.
• Phase 2 involves incorporating any government direction into the programme prior to community engagement.
• Phase 3 is focused on engaging on adaptation, using the information gathered in Phase 1, with those communities most vulnerable to climate hazards.
Phase 1 is still under way, but the council has started its adaptation journey. It has workshopped the risk to council assets with subject matter experts and is developing a narrative on the risk to the network and individual assets. The output from this phase of the work will include identification of hotspots of known vulnerabilities and risks to the existing infrastructure. It will be used to inform council decision making and asset planning.
At a district-wide scale, these strategies help a range of stakeholders to understand the vulnerabilities of the system and the threats to current levels of service. This will also help prioritise investment in risk reduction and ensure that future investment in water infrastructure considers natural hazards and climate change.
The approach taken by Porirua City Council was to use the knowledge of its asset managers and readily available hazard information to have a first pass look at the risks to its infrastructure. Having facilitated conversations, the council is better placed to plan the future management of these hazards. Capturing the knowledge held by asset managers helps to understand the scale of the challenge and the assets most at risk. By broadening that conversation to a systems level, a deeper understanding of the vulnerabilities and cascading hazards was developed. This more complete picture of exposure helps make the case for investment and identify areas of adaptation priority.
Porirua’s experience shows you don’t need a lot of money or time to get started. Every small step taken contributes to spending less on response and more on preparedness.
Māori are on the frontline of climate change, and bring local knowledge and alternative viewpoints that can inform adaptation responses. The Deep South Science Challenge experienced the benefits and challenges of partnering with Māori researchers in delivering a national science challenge, from 2014 to 2024.
The mission was to enable New Zealanders to adapt, manage risk and thrive in a changing climate. Early in the process, staff heard from prospective Māori research teams who felt significant systemic barriers hindered their participation in this mahi. They cited a lack of organisational capacity among hapū or marae communities to actively compete for funding and the privileging of Pākehā notions of “research excellence” when assessing the capability of Māori teams.
In 2021, the Kāhui Māori of the Deep South Challenge embarked on a new funding process for its Vision Mātauranga research programme to directly address inequities faced by Māori in participating in climate adaptation research.
The programme sought to reflect climate priorities already identified by hapū and iwi as well as encourage a diverse research programme with emphasis on embracing mātauranga, tikanga and te reo Māori. Systemic inequities were addressed in several ways by taking steps to:
• understand and reduce barriers to access
• recognise the capability, expertise, leadership and mātauranga of kairangahau Māori (Māori researchers), tohunga, and community kaimahi who work outside of formal institutions
• changing assessment criteria to reflect Māori values
• ensuring kaupapa rangahau spoke directly to Māori aspirations
• streamlining processes
• front-loading engagement support (ie, working with teams one on one over time to support proposal development, and providing feedback on drafts)
• writing strong copy that wasn’t tokenistic or appropriative of whakaaro Māori.
The process supported the development of 25 successful research projects, many of which addressed issues faced in the water environment, including:
Drinking water in Te Hiku o Te Ika, which explored the quality and sustainability of water supply in Te Hiku o te Ika (Northland) to contribute to the wellbeing and viability of these communities into the future.
Ki te whare tū tonu, ki te whare manawaroa: Towards a climate-resilient meeting house, where Patuheuheu-Ngāti Haka hapū members undertook research aiming to ensure Waiōhau marae is sustainable and resilient to climate impacts, such as changes to the water table that are affecting sewerage and drinking water systems.
Risk management for Māori coastal assets, which combined data about soil, floods, topography, river sedimentation and sea level rise to identify the most vulnerable areas of our coastal farms and adaptation strategies for managing wetlands and landscapes.
Genuine partnership with Māori communities is critical for effective adaptation, and is achievable, but comes with responsibilities, including:
• sufficient resourcing
• transparency, openness and a willingness to challenge common practices
• not raising expectations that cannot be fulfilled
• being responsive, flexible and innovative to find new ways of doing things or to change when needed
• a commitment to reciprocity of some kind.
These responsibilities extend beyond the research sphere and provide instructive guides for others working in adaptation.
Further information on the Vision Mātauranga research outcomes enabled by the project is available here: https://deepsouthchallenge.co.nz/our-research/
This submission outlines lessons from the programme’s development: Submission on Te Ara Paerangi Future Pathways green paper: https://deepsouthchallenge.co.nz/submission-te-ara-paerangi/
Wastewater systems are sensitive to changes in climate. However, sewage collection, treatment and disposal systems have been largely designed according to past climate conditions where climate has been assumed to be stationary for the service life of the asset. With most components of wastewater systems having long operating lives, they will now likely be exposed to significant changes in climate over those periods.
Assessing the potential impact of climate change on wastewater systems is complex. There are many inter-dependent components, and many climate-related parameters that affect the design and ongoing operation of the assets.
In 2018 the water sector in Victoria, Australia, recognised a need to enhance knowledge on the impact of climate change on sewerage systems. Best practices in science and engineering were brought together to assist sewerage planners, managers and operators to make business decisions that took a changing climate into account. The resulting Guidelines for the Adaptive Management of Wastewater Systems under Climate Change in Victoria provides a systematic approach for considering risks and planning for adaptive management.
The guidelines were written to help water corporations identify, assess and effectively manage or adapt to priority climate change risks. The work commenced with the preparation of five technical discussion papers considering the effects of climate change on wastewater systems. Following this, a scope for the guidelines was developed with the Victorian water corporations.
Designed to serve a broad cross-section of people, including water corporation personnel, board members and technical advisers, the content primarily focuses on wastewater practitioners as they consider the potential effects of climate change on wastewater infrastructure design.
Building on existing guidance and climate science literature, analysis processes and datasets, the guidelines outline how climate change could be considered in planning and management of wastewater systems. They establish the context and need to plan for a changing climate and provide information to assist understanding of the potential impacts of climate conditions on wastewater infrastructure, water reuse and biosolids reuse.
Methods for prioritising and investigating climate impacts throughout wastewater systems are presented through an adaptive planning cycle, an essential framework for long-term resilience in response to change and uncertainty. Case studies are provided to assist practitioners’ understanding.
The processes outlined and explained in the guidelines are broadly applicable to Aotearoa New Zealand, making them a useful resource for practitioners, water entities and consent authorities in understanding the risks posed to this infrastructure. Further, it offers advice on practical approaches to managing the exposure of these systems and a framework for adaptive management, especially helpful to those who are planning the future management of water infrastructure and are unsure of what process to follow and where to start.
The guidelines are the first that are specific to climate change adaptation of wastewater systems in Victoria and reflect a developing practice that will evolve through implementation. There is a future opportunity to update and amend this guidance to be specifically relevant to the challenges faced by the water industry in New Zealand.
Planning and design without further consideration of climate change conditions.
Are the external and internal drivers for consideration of climate change understood?
Have they changed or are they likely to change?
What component/s of the wastewater system are being considered?
What
Review service obligations and objections.
Will climate hazard conditions hinder achievement of objectives?
Assess the potential impact of climate change on wastewater systems.
Assess the risks to onjectives.
Are there risks that require interventions to adapt to climate change?
Establish operating conditions for the wastewater system and planning timeframe.
Characterise future climate hazard conditions and establish the base case.
Create reference period of conditions.
Select, understand and work with climate change scenarios.
Source & apply climate change scaling factors.
Identify interventions and the associated knowledge, values and rules to align for an intervention to proceed.
Identify triggers, decision points and limits to define and assess future pathways.
Specification of monitoring regimes.
• Increased peak sewer flow rates due to inflow and infiltration.
• Flooding of sewer network, pump stations, treatment infrastructure and recycling water and biosolid storages.
average and extreme temperatures,
Sewage more highly concentrated –alteration of sewer corrosion, odour and treatment process Dust generation from biosolid stockpile Service disruption due to grid power supply failures Spontaneous combustion and fire Altered treatment process efficiency
• Increased odour generation and emissions.
• Accelerated corrosion of sewer pipes.
• Mechanical failure of infrastructure. Heat-related failure of electrical and /or electronic infrastructure.
• Increased/peaks in water and recycled water use/ demand by customers.
• Altered rates of biological treatment processes.
• Increased power requirement/usage to control temperature of mechanical and electrical equipment.
Increased average and extreme temperatures, heatwaves
• Increased incidence of spontaneous combustion of biosolids stockpiles.
• Temporary reduction/loss of grid power supply due to excessive demand and associated load shedding.
• Dry soils lead tree roots to seek water
• Enhance shrink-swell of soils under alternate dry/ wet conditions.
• Ground water levels fall • Reduced soil corrosivity Sewer damage to soil movement, soil corrosivity and tree root
• Low flows in waterways to which treated water/ spills discharged.
• Reduced sewer flow and change in sewage concentrations.
• Increased/peaks in water and recycled water used/ demand by customers.
Drier conditions (reduced rainfall, greater evaporation), more severe droughts
Physical impacts from climate change have the potential to deteriorate the performance and condition of water assets, thus affecting the level of service and cost. It is important that governments, regulators and water utilities understand these climate risks to enable effective management and planning to optimise long-term investment. Physical climate change impacts should be considered during business and asset management planning, but this requires that climate resilience is embedded in all service areas at an appropriate level of granularity.
One water utility embarking on a journey to improve its climate resilience implemented organisational changes to:
• Enable the identification and assignment of responsibility for managing physical climate risks in business and asset management planning
• Identify the scale of investment required in resilience over time
• Consider climate resilience in management approaches for physical assets
• Include climate resilience as a criterion in project investment appraisals
• Embed the latest climate science and modelling approaches within decision making and delivery
• Improve the communication of climate risk and adaptation plans to stakeholders.
In the first phase of its work, guidance on the choice of emission scenarios for use in business and asset management planning was developed. A decision tree was created to guide the choice between a high and medium GHG emissions scenario, taking account of the sensitivity of the decision/application to climate change as well as the level of infrastructure adaptability and criticality. Regional changes in climate variables relevant to the company’s operations were also analysed and summarised in a set of climate projection data tables and maps.
In the second phase, a corporate scale Climate Change Risk Assessment was undertaken, engaging with stakeholders across all business areas to identify, record and assess climate risks under two GHG emissions scenarios. A semi-quantitative approach to risk scoring was used, based on climate projection data, modelled impacts of climate hazards where available (eg, water resources planning), supplemented by review of available operational performance records (eg, pollution incidents relating to weather events), and outputs of the stakeholder engagement. As part of the corporate risk assessment, more detailed quantitative assessments of key risks were undertaken to update risk scores. These risks included:
• Number of water and wastewater assets at risk of surface water, coastal and river flooding
• Number of properties at risk of sewer flooding
• Areas at risk of water supply deficit and level of deficit
• Water treatment works at risk of deteriorating performance due to deteriorating water quality
• Wastewater treatment works at risk of non-compliance under future low-flow conditions in receiving waterbodies.
The output of the risk assessment was a prioritised list of climate risks that was used to develop an adaptation plan that has been shared with regulators and customers. The plan describes how climate is projected to change, the priority risks faced and current and planned actions. The quantitative assessments of key risks are also being used to estimate the investment needed to improve resilience to key risks – one of the inputs to development of the long-term (25 year) investment strategy.
Work is ongoing to quantify other risks, including disruptions to the treatment process, sewer overflows and increased deterioration of assets. Engagement is ongoing with key stakeholders within the different business areas to embed climate risk and resilience considerations into planning and delivery processes.
In addition, management approaches are being developed for key asset types and the criteria used in project investment appraisal are being updated to include climate resilience. Guidance has been prepared on how to identify the impact of climate change on the need for investment, the potential costs associated with climate risks for different options and the benefits of more resilient options. This is being trialled on water and wastewater project appraisals with the intention to include these considerations in project investment appraisal as standard.
To bring together the increasing quantity of work and evidence on climate risks and resilience-building measures, an Adaptation Hub is being created. This helps staff source climate change adaptation analysis, data and advice relevant to their roles within the business.
This work has enabled the utility to plan and invest in mitigating against physical climate risks over time. Outcomes include:
• Improved understanding of and accessibility to climate data
• Guidance to delivery teams on modelling and accounting for climate scenarios to better refine the materiality of key risks, where and when to invest to mitigate potential future service disruptions
• Improved visibility and quantification of priority risks that need long-term investments. Quantitative assessments have enabled the utility to account for these in the latest round of long-term investment planning, leading to more targeted investment in resilience
• Inclusion of climate resilience criteria in all stages of investment appraisal alongside all other decision-making criteria
• Engagement across a wide range of business functions and teams for mainstreaming climate resilience to deliver undisrupted water and wastewater services to the public while protecting the environment.
This case study illustrates how governments and water utilities can drive change and investment in resilience within the water sector. Embedding climate resilience as a key metric can be used to evaluate the long-term performance of business operations and inform the investments required to maintain service levels.
Internationally, many towns, cities and utility providers are publicly stating that they are working to improve their resilience. However, it is often unclear how this will be achieved and what their current resilience level might be. Unfortunately, resilience is often not easily or effectively measured. There are strong reasons to change this and better understand and manage our levels of resilience. Benefits include:
• Risk avoidance (reducing exposure to risks that in some cases will worsen over time)
• Developing appropriate response strategies to threats (fight or flight)
• Ensuring that the most vulnerable people in our communities, such as the elderly, are supported
• Increasing our coping ability (for the next Cyclone Gabrielle).
Having good metrics for resilience should allow best practice to be shared and improve performance across and between communities, infrastructure providers and local and central government, considering the broader implications of what it means to operate in high-risk places. This case study examines existing approaches to measure and compare resilience.
At its most simplistic, resilience can be seen as the flip side of risk – that is, the less risk a network, asset or community is exposed to, the more resilient it is. More elaborate definitions incorporate concepts such as ‘advancing despite adversity’, ‘emerging stronger through disruption’, ‘adjusting easily to misfortune or change’. These suggest bouncing back from adversity.
For climate change, one definition is: ‘The capacity of social, economic and ecosystems to cope with a hazardous event or trend or disturbance’ (IPCC). This introduces a multi-faceted approach and suggests that ‘coping capacity’ needs to be understood – perhaps even measured.
The IPCC definition goes on to say that coping capacity is achieved ‘by responding or reorganising in ways that maintain their essential function, identity and structure … while also maintaining the capacity for adaptation, learning and transformation’. This may be understood as providing for the continued survival of those systems in some recognisable form, and for their continued ability to adjust as further changes occur.
A NZ Treasury report9 concluded that the NZ economy is resilient to climate change, finding that we are likely to be better able to adapt than many other countries because of our strong institutions and track record of economic and fiscal resilience. However, the report did not assess climate impacts on our infrastructure and communities.
9 https://www.treasury.govt.nz/publications/climate-economic-fiscal-assessment/nga-korero-ahuarangi-me-te-ohanga-2023
The following points outline some of the complexities of resilience.
• It exists across several dimensions: For example, it may be viewed across the “four wellbeings” – social, cultural, environmental and economic. An area may have strong resilience in one of these components but be low in others.
• Broad collaboration is needed: System-wide and collaborative resilience development is likely to be the most successful (‘it takes a community to ensure resilience’). This means it should occur across individuals, community groups, businesses, government agencies, international organisations, etc.
• Adaptive capacity is the ability to adapt or change – being change-ready. As climate change impacts increase and accelerate, we need to ensure that our infrastructure and governance organisations are ready to change and fit for purpose.
• Physical resilience may relate to the appropriateness of existing infrastructure for coping with expected shocks or slow onset stresses. For example, understanding the:
• status (failure thresholds, strengths and weaknesses, etc) of critical elements such as stopbanks
• expectations that communities have for infrastructure to protect lives and property
• quality of buildings and infrastructure
• affordability of infrastructure services
• availability of early warning systems
• appropriate regulatory frameworks for resilience measures to be applied (building standards, controls on development in high-risk areas, etc).
• Social resilience is typically less tangible and more difficult to measure, incorporating elements such as community cohesion, willingness to support your neighbours in times of need, the sufficiency of incomes to invest, the availability of safety nets (eg, social security, public hazard insurance). On the flip side, these can include measures such as socio-economic disparity, homelessness, crime rates, trust in government.
• Cultural resilience operates in both a NZ-specific sense (reflecting the needs of mana whenua and embracing mātauranga) as well as a broader cultural wellbeing sense (accounting for ethnic diversity, varied spiritual needs).
• Economic resilience relates to the ability to afford the levels of service expected, as well as to recover from shocks and stresses that may occur with greater frequency or intensity in the future.
• Environmental resilience is about how well ecosystems, and our environment, can cope with change and recover from stresses and shocks. Unfortunately, just as some of our human systems struggle with an accelerating rate of change, many of our natural systems are also not able to change at the pace required.
Resilience measurement is a new and rapidly developing area. Some tools take narrower resilience definitions using ‘objective methods’. For example, relying on large-scale data collection and standardised metrics – which may risk having little input from those being measured and be based on general assumptions (eg, higher wealth = greater resilience). A 2016 comparison of community resilience tools10 found that the environmental dimension is often not adequately included, and that relationships across various scales are largely neglected.
10 Ayoob Sharifi (2016) A critical review of selected tools for assessing community resilience. Found at: https://www.sciencedirect.com/science/article/pii/S1470160X16302588
Other tools are attempting to be broader using ‘subjective methods’. These may encompass all four wellbeings and rely on people’s self-assessment of their capacity to deal with challenges. However, they can be more difficult to quantify, may have limited comparability and typically involve high effort and cost to obtain data. Some examples of selected resilience tools are shown below.
The Disaster Resilience Scorecard for Cities11 (UNDRR) provides assessments (preliminary and detailed) that allow local governments to assess their disaster resilience, structured around UNDRR’s Ten Essentials for Making Cities Resilient12
Organise for disaster
Identify, understand and use current and future risk scenarios
Strengthen financial capacity for resilience
Pursue resilient urban development and design
Safeguard natural buffer to enhance the protective functions offered by natural ecosystems
Strengthen institutional capacity for resilience
Understand and strengthen social capacity for resilience
Increase infrastructure resilience
Ensure
The OECD13 has a model for city resilience which incorporates the four wellbeings and includes key measures for each. It captures aspects such as economic growth, proximity to key services, access to greenspace, population density, government representation and unemployment.
The City Resilience Profiling Tool14 (UN-Habitat) gathers four sets of data covering: city characteristics; local government and stakeholders; shocks, stresses and challenges; urban elements (across the four wellbeings).
The Quick Risk Estimation (QRE) tool15 (UNDRR) aims to identify and understand current and future risks / stress / shocks and exposure threats to both human and physical assets. It requires
11 UN Office for Disaster Risk Reduction (2017) Disaster Resilience Scorecard for Cities. Found at: https://mcr2030.undrr.org/disaster-resilience-scorecard-cities
12 UN Office for Disaster Risk Reduction, The Ten Essentials for Making Cities Resilient. Found at: https://mcr2030.undrr.org/ten-essentials-making-cities-resilient
13 OECD, Climate and resilience in cities. Found at: https://www.oecd.org/en/topics/sub-issues/climate-and-resilience-in-cities.html
14 UN Habitat (2018) City Resilience Profiling Tool. Found at: https://unhabitat.org/guide-to-the-city-resilience-profiling-tool
15 UN Office for Disaster Risk Reduction (2023) Quick Risk Estimation (QRE) Tool. Found at: https://mcr2030.undrr.org/quick-risk-estimation-tool
It requires input for likely hazards and events, exposure, vulnerability and adaptation measures. A recent addition provides assessment of integrated climate and disaster risks (Climate QRE16).
The Enabling Resilience Investment Framework17 includes engagement, assessment and learning activities, methodologies and processes to address knowledge gaps, reveal tensions and trade-offs and create governance that generates wider adaptive options and pathways.
The Urban Resilience Tool17 (World Government Summit) provides a numeric index of a city’s resilience level, based on the exposure to hazards, vulnerabilities and the institutional capacity to respond, recover and transform.
16 CSIRO, Enabling Resilience Investment Framework. Found at: https://research.csiro.au/enabling-resilience-investment/
17 World Governments Summit, Urban Resilience Tool. Found at: https://www.worldgovernmentsummit.org/observer/resilience-tool
As natural disasters increase in intensity and frequency, and failures of our aging infrastructure become more common, effective communication of hazards and risks to the public is important to:
• Enable the public to make informed decisions about how to protect themselves from harm
• Reduce the fear and anxiety people may feel about a potential hazard
• Build trust between the public and the organisations managing the hazard.
Safeswim (www.safeswim.org) was set up in February 2017 to provide a public-focused web platform with real-time forecasted water-quality risk indicators for swimmers. Safeswim has proved to be an important means of communication for Auckland Council during flooding incidents, informing the public about the health risks related to floodwater. The Safeswim initiative has greatly increased public awareness around water quality issues and causes, and as a result there has been increased funding and political support to improve water quality. Successful approaches implemented within Safeswim include:
• A diverse delivery team – data integration specialists, modellers, water quality scientists, communication specialists and web designers.
• The creation of a purpose-built web platform that is easy to navigate and understand, summarising complex technical information in an easy-to-digest format.
• The ability to access up-to-date information about the risks of swimming in a particular place at a particular time. Aucklanders can access current and forecasted water quality for a three-day period alongside climate and other local safety information. Details about the beach environment and facilities and short videos on water quality in the region also contribute to this effective public communication tool.
• Information is also relayed onsite, with digital signs at main Auckland swimming spots.
Key to the success of this programme was development of a large-scale, predictive water quality digital twin covering the interaction between atmospheric conditions, the wastewater/stormwater network and the marine environment.
Lessons from the development of Safeswim include the importance of effectively communicating hazards and risks in real time to the public, having a single source of truth and translating public health risk into easy-to-understand outputs.
For further information on this case study refer to: Wohlleb, Tomasi, Magalhaes, Tryon, Ellman (2018) Safeswim: Integrating real-time monitoring and modelling for near-term predictions of water quality.
Assessing and prioritising adaptation actions requires a good understanding of the interrelationships and interactions between the climate, ecological and human systems. While global climate change adaptation has been widely studied, many policies fail to comprehensively consider the interdependencies within these systems. This is largely because climate impacts are complex and challenging to tackle all at once.
This case study, part of the Deep South National Science Challenge (GNS-IN210), involves a collaboration between Market Economics, GNS Science, the University of Otago, Resilient Organisations and EM Consulting. A visual platform was developed using the Hawke’s Bay region as an example to communicate complex system relationships in a user-friendly manner.
Modelling examined cause-and-effect relationships, interdependencies and feedback processes related to droughts and floods affecting water supply and wellbeing in Hawke’s Bay.
To link human and environmental systems to exposure, vulnerability or impacts and feedback, the study employed graphical methods, including System Dynamics (SD) and Bayesian Networks (BN) (Figure 7). SD models capture direct and indirect impacts on socio-economic systems through time-dependent feedback loops. BNs are probabilistic graphical models that represent knowledge about a system and the causal relationships between its components through conditional probabilities.
Figure 7: Methodological approach
Through collaborative workshops with experts and integration of previous knowledge, the complex system was graphically mapped using SD, illustrating connections among environmental systems (atmospheric, hydrological), human systems (economic, social, built environment, governance) and wellbeing outcomes (income, health, etc). Information was brought together in an interactive visual Wellbeing Platform (Figure 8). By tracing connections and causal loops, users can explore how changes in the hydrological system due to climate change affect wellbeing.
Using BN, quantitative relationships between precipitation and groundwater modelling data were incorporated into the Wellbeing Platform to demonstrate connections between climate scenarios and the groundwater system. Precipitation and health modelling were integrated to analyse the relationship between campylobacter infection risk and extreme rainfall, and brought together in an interactive map.
Consolidating information from various sources provides a systemic view of climate change impacts on the economy, environment, public health and wellbeing. Users can explore subsystems and understand interconnections. It allows users to qualitatively assess the impacts of potential adaptation options and explore individual and collective wellbeing implications associated with water-related climate change concerns.
The platform supports a shared understanding of very complex systems and interconnections and feedback to visualise the outcomes and trade-offs of potential human actions and choices. This knowledge-integrating communication tool, and its extensions, can be used to address water-related climate challenges across New Zealand to:
• Test ideas and support discussions about potential outcomes of future projects
• Provide an educational, communication and engagement tool to foster shared understanding within communities
• Support the scoping and design of models
• Facilitate the integration of research components, offering structure for collaboration and interdisciplinary approaches
• Identify potential knowledge gaps
Further information on the project is available here: https://deepsouthchallenge.co.nz/research-project/ supporting-community-wellbeing-when-water-is-scarce/
Explore the Wellbeing Platform at the following link: https://exchange.iseesystems.com/public/nicolamcdonald/ deep-south-project-interface/index.html#page1
Relying on historic data to extrapolate future conditions has the potential to lead to significant under estimation of future extreme weather events. Integrating climate change considerations into modelling approaches remains challenging due to the lack of guidance and large uncertainties in climate projections. Despite this, it is vital that we begin to plan and adapt for a changing climate.
An example of using data to better support the management of extreme events is Mott MacDonald’s Flood Management System (FMS) Moata Smart Water platform piloted in Piha. Although not using future projections, the FMS utilises machine learning and a digital twin to better predict flood events and implement more effective emergency management during such events.
The February 2023 events on Auckland’s west coast showed that flooding from extreme rainfall presents a high risk to people’s safety and is damaging to assets and public property. Reliable information about the timing, location and intensity of rainfall is critical for emergency flood management. For many regional authorities, deploying sufficient rain gauges to adequately capture the spatial variability of rainfall and manage large areas is difficult, if not impossible.
The FMS integrates rainfall radar and rain gauge data and applies a novel radar calibration approach to improve spatial rainfall estimates and generate automatic rainfall maps. Nowcasting machine learning (ML) is applied to a calibrated flood model, speeding up simulation times from hours to seconds while retaining the accuracy of the physically based hydraulic model. Nowcasts are applied as inputs to the ML model to produce real-time flood forecasting maps with lead times sufficient to target an operational response.
This method provides a single source of truth for observed hydrometric and radar data as well as forecast information, providing immediate visual insights for flood managers. The data is consolidated and presented in a web-based, geospatial interface. Geospatial map layers give context to better visualise flood-related information such as overland flow paths, flood plains and flood prone areas.
The FMS displays rainfall forecast for the next two hours (the nowcast) spatially, showing the total depth of rain anticipated. Forecasted flood depths and extents generated by the flood forecasting model are also displayed as a geospatial layer, with animation to clearly represent how flooding is unfolding in the next two hours.
The risk to life for people alongside Piha Stream is largely dependent on decisions made during storm events, and their preparation for such situations. The two-hour warning window provides information that can help authorities make critical decisions, such as when to close roads and evacuate residents in danger zones.
The improved rainfall estimation and nowcasting provided by the FMS enables automated, near real-time, high-resolution rainfall depth maps for Piha, providing Auckland Council with an immediate understanding of forecast and unfolding rainfall events. It consolidates all information related to flooding in a central platform to support Auckland Council’s operators and their planning teams.
With the intensification of extreme rain events leading to widespread catastrophic impacts in Auckland and around the globe, the pilot FMS developed for Piha could be widely applied to other flood forecasting systems.
An important advantage of using a ML model is the ability to run an ensemble of models to better understand the probability of flooding, which allows authorities to mobilise adaptation with more confidence. Improved warnings, by even a few hours, can significantly improve flood response and protect lives and properties.
Moreover, having access to a digital twin platform such as Moata allows flood managers to optimise their flood models by tapping into multiple data streams such as the network of rain gauges, level monitoring devices, cloud-connected cameras (CCTV) and social media. The extra information enables verification of flood extents and flood depths and provides feedback to ML models to improve future forecasting of the system’s response to extreme events.
The FMS has the potential to integrate with other systems such as transportation digital twins, to improve management of traffic and emergency response routing in flood events, or public communication channels. Forecast flood extents and depths can be used to assess economic losses, social disruption and populations at risk, providing a complete picture for decision makers.
For further information on this case study refer to: S. Ho, N. Tomasi, N. Brown, K. Fordham (2024) Moata Smart Water: Flood forecasting using machine leaning and digital twin
The resilience of critical infrastructure networks (electric power, transportation, telecommunications, three waters and fuel) has a significant impact on the ability of society to rapidly recover after a natural hazard event. A key aspect of infrastructure network resilience is the dependencies between networks, where components of one network rely on components of another network to function. This can lead to further cascading failures and feedback loops to those initiating infrastructures. The nature and magnitude of these dependencies are generally not well understood, often leading to unforeseen social and economic consequences.
Systems-of-systems models of critical infrastructure networks have been developed at various scales, nationally down to a city scale. Using a structured framework, geospatial models of the different infrastructure networks were developed, modelling the connectivity and flow of individual networks, links between networks that represent dependencies and affected populations. When exposed to different natural hazard events, the effect on individual network components can be defined, and if aspects of functionality are reduced this propagates through its own network and out into other dependant networks.
This approach provides a quantifiable assessment of direct and indirect impacts of shock and slow-onset hazards on infrastructure networks. An example application to a region of Christchurch is shown in the figure from Brunner et al (2024), where the effect of sea level rise and coastal flooding on infrastructure service was assessed. Yellow areas are properties affected by coastal flooding directly (direct inundation). The other points are where loss of service of one or more infrastructure networks has occurred (indirect impact due to components affected by the coastal flooding at another location within each network). The indirectly impacted properties are well in excess of the directly impacted properties for this example.
These approaches rely on access to infrastructure network data for any region of interest. As it can be difficult to gain access to data from different network providers, there needs to be a higher-level strategy put in place to collect, store and maintain the underpinning data. Given the continual evolution of these networks, this up-to-date data is needed to ensure resilience investment decisions are made based on the current and future status of these networks.
For further information on this case study refer to: L.G. Brunner, R.A.M. Peer, C. Zorn, R. Paulik, T.M. Logan. (2024). Understanding cascading risks through real-world interdependent urban infrastructure, Reliability Engineering & System Safety, 241, https://deepsouthchallenge. co.nz/resource/cascading-risks-real-world-urban-infrastructure/
The effects of climate change, urbanisation and densification of cities have raised concerns about the ability of existing stormwater management systems to withstand, adapt and recover from storm events and unexpected disturbances. To help support decision making, a structured approach is needed to evaluate the resilience of stormwater management systems considering all relevant dimensions.
An index-based framework was developed to quantify the resilience of stormwater management systems during rainfall events. This framework focused on the following dimensions:
• Hydrological characteristics of catchments – the ability of the catchment to absorb rainfall (hydrology dimension)
• Hydraulic characteristics of primary stormwater systems – the ability of the piped network to deal with runoff (primary hydraulic dimension)
• Hydraulic characteristics of secondary stormwater systems – the ability of surface flow paths to deal with runoff (secondary hydraulic dimension)
• Structure of primary stormwater systems – the influence of network structure on resilience (network structure dimension)
Each approach was based on the outputs of standard stormwater system modelling software and readily available geospatial data characterising catchments. Because of this, it can be used for existing networks, the addition of devices and controls in the network, changes to network structure and to assess the effect of network deterioration. This can be assessed across different design rainfall events and the changing characteristics of these because of climate change.
This framework provides a set of tools to evaluate the resilience of each dimension of the stormwater management system for different rainfall events. It provides a more structured approach to quantify the resilience of existing networks and to test the impact of potential resilience interventions. This supports the identification of the best practicable options to improve the resilience of each dimension and reduce the consequence of flood hazards due to vulnerable stormwater management systems.
As this framework can be applied to typical stormwater modelling outputs, the approach can be applied to any project where stormwater modelling is being undertaken.
Figure 10: The four dimensions of stormwater management systems
For further information on this case study refer to: Valizadeh, N., Shamseldin, A.Y. & Wotherspoon, L. Quantification of the hydraulic dimension of stormwater management system resilience to flooding. Water Resources Management 33, 4417–4429 (2019). https://doi-org/10.1007/s11269-019-02361-1 Public Link: https://resiliencechallenge.nz/outputs/ quantification-of-the-hydraulic-dimension-of-stormwater-management-system-resilience-to-flooding/
Valizadeh, N., Shamseldin, A. Y., Wotherspoon, L., & Raith, A. (2023). Indicator-based resilience assessment of stormwater infrastructure network structure. Urban Water Journal, 21(3), 337–348. https://resiliencechallenge.nz/outputs/ quantification-of-the-hydraulic-dimension-of-stormwater-management-system-resilience-to-flooding/
Owners, operators and investors need to have confidence that infrastructure assets are resilient to the hazards posed by climate change, while minimising disruption, repair costs and protecting performance of vital services.
There are increasing requirements emerging to measure and report climate change risks. However, there is a lack of understanding, frameworks and methodologies to support decision makers undertaking this work. It is difficult to decide when managed retreat should be considered, when to build elsewhere and when to invest in adaptation.
Presently, infrastructure owners and investors often fail to properly incorporate physical climate change risks into infrastructure optioneering and design. Current industry approaches to assessing climate risks do not quantify impacts in ways that support cost-benefit analysis or inform business case decision making. PCRAM (Physical Climate Risk Assessment Methodology) addresses this problem.
PCRAM is a methodology for quantitative climate change risk assessments to support investments in resilient infrastructure. It was developed by Mott Macdonald under the Coalition for Climate Resilient Investment in the run up to COP26. The four-step methodology translates physical climate risks into quantifiable impacts on asset performance to integrate into infrastructure investment decision making. It is a multidisciplinary exercise requiring climate data scientists, engineers, asset owners, managers, operators and finance practitioners to collaborate equally and closely from the outset.
The assessment process relies on professional judgement with input from multiple stakeholders to quantify the impact of climate change physical climate risks on critical component(s) of an asset. Practitioners are required to make and communicate pragmatic assumptions where information may not be available and to accept and highlight uncertainty. The assessment is agnostic to the nature of value (economic v social v environmental), can be applied by public or private sector entities and is applicable to all types of structures and infrastructure.
The four key steps and three gates involved in the process are shown in the diagram (Figure 11). The methodology should not be seen as a one-off exercise but as a live, iterative process that is regularly updated as new information becomes available.
In Step 1, the project team defines the objectives, identifies the scope of the assessment and collects essential information about the climate, the infrastructure asset(s) and the associated critical components, as well as the key performance indicators (KPIs). This data collection is used to develop the Base Case performance of the asset. At the end of Step 1, the project team arrives at Decision Gate (A) and decides if the data is robust, complete and sufficient to proceed to the detailed materiality assessment. If not, the project team returns to Step 1 to collect further information or modify the scope of the assessment to better reflect data availability.
In Step 2, the project team develops the inputs for the Climate Case(s) by taking the data and assessing the severity of impact to the infrastructure stemming from relevant climate hazard(s) using the KPIs to quantify the risk. At the end of Step 2, the project team arrives at Decision Gate (B) and decides if the physical climate risks are material to the asset(s) given the KPIs. In the event the physical climate risks are not material, the assessment is complete.
In Step 3, the project team develops the Resilience Case(s). Initially, a list of options to improve resilience of the asset to material physical climate risks is generated through discussion between the climate practitioner, engineers and asset managers. Resilience Case(s) are composed of feasible resilience options that may reduce the severity of impact of material climate hazards. These resilience options (effectively an improved infrastructure asset) are re-run through the Step 2 Materiality Assessment to measure projected benefits against the cost of the options (CAPEX and OPEX). At the end of Step 3, the project team arrives at Decision Gate (C) and decides if there are resilience options available to reduce the severity of impact.
In Step 4, the project team undertakes an economic and financial analysis to de-risk asset exposure to physical climate risks and recommends resilience options.
New Zealand’s infrastructure is highly vulnerable to climate change. Recent extreme weather events have caused damage to assets and impacted services and communities. Without action these impacts will become more severe as climate change worsens. With action we can build more resilient infrastructure – reducing costs, securing revenues and improving performance. The aim of PCRAM is to encourage the identification of sound valuation practices to unlock investment in climate resilience through guiding and encouraging asset owners, operators and investors to monitor and disclose physical climate risk exposure in their portfolios.
The methodology has been made publicly available on the Mott MacDonald website to support the infrastructure industry with thinking about physical climate risks.
For further information on this case study refer to: CCRI & Mott MacDonald (2023) Physical Climate Risk Assessment Methodology (PCRAM): Guidelines for Integrating Physical Climate Risks in Infrastructure Investment Appraisal
Water services delivery in New Zealand is facing significant future challenges that are influenced by the choices made today. Such decision making relies on anticipating change, which is difficult when the number of variables increase in tandem with climate changes. For example, preferences change over time, rare events cannot be ruled out and large investments of public money are involved.
A Dynamic Adaptive Policy Pathways (DAPP) approach is a method that addresses uncertainty, where decisions are made over time in dynamic interaction with the system of concern and where decisions cannot be considered independent of each other. It is an anticipatory planning process used proactively for flexible adaptation over time and in response to how the future unfolds.
It considers the potential for the locking in of decisions that can reduce flexibility in the future. It is based on the premise that decisions and policies have a design life and can fail when the operating conditions change and a lead time for implementation of decisions exists. DAPP can be used in any decision setting where there are uncertainties about the future and risk is progressively changing.
The DAPP process, summarised in the diagram, was used to support strategic planning for Wellington Water’s water supply. In addition to the diagram, the questions listed should be considered throughout the DAPP process to support both long- and short-term adaptation objectives:
• What are the first impacts we will face as a result of climate change?
• Under what conditions will current strategies become ineffective in meeting objectives?
• When will alternative strategies be needed, given that implementation has a lead time?
• What alternative decisions can be taken to achieve the same objectives?
• How robust are the options over future climate/sea-level rise scenarios?
• Are we able to change a path easily and with minimum disruption and cost?
Currently, the implementation of DAPP has proven difficult; sectors are at different stages of thinking about climate risk and adaptation. Current governance mechanisms are a major impediment to embedding DAPP in practice; we make policy and legislation for now – static in time and space – and we need to move to more adaptive modes of governance and decision making as climate impacts change and intensify. There are many opportunities to get wider risk assessment processes, emergency management and investment planning and adaptation better aligned. Depending on the context, community engagement and mana whenua can be integrated throughout the DAPP process. Systematic approaches such as DAPP can support more holistic decision making within the context of future uncertainty where risk is progressively changing.
• Define system boundary
• Define objective
• Set planning horizon
• Document uncertainties and high level assumptions (embrace uncertainty – avoid reducing or simplifying)
• Identify decision-making scale and investment cycle timing (so DAPP is developed at an appropriate level of robustness and delivered at the right time to inform investment planning)
• Decide how system performance will be assessed
• Develop strategic planning tools
• Assess existing system performance
• Assess baseline future performance if no changes are made
• Determine the magnitude for interventions to be of strategic significance (so options/portfolios are developed at an appropriate scale)
• Long list of options for achieving objectives over planning horizon (with high level and/or qualitative assessment of cost, benefit, deliverability and lead time)
• Develop short list of options using multi-criteria analysis or other process
• Assess feasibility of short list options (improve understanding of cost, benefit, deliverability and lead time)
Wellington Metropolitan Water Supply
Baseline supply/demand balance
Over 100 options were considered
• Sequence options into pathways that represent themes or general approaches
• Stress test pathways (test performance of each option combination against all combinations of future scenarios using strategic planning tool)
• Review and update options and pathways, and finalise stress test
• Assess performance of pathways (e.g. robustness to future scenarios, population supported, cost, etc
• Identify path dependencies and potential for unintended negative consequences (including if assumptions are wrong).
• Select robust pathway
• Determine short term “do now” interventions
• Determine investigations needed to prepare for future options
• Develop monitoring plan of signals, triggers to initiate actions and performance thresholds
• Complete adaptive plan
• Summarise “do now” interventions and investigations needed to prepare for future decisions
• Develop and present investment advice (delivered at the right time and with the right level of supporting information)
Implementation plan
The three key interventions were summarised as “Keep-Reduce-Add’. These referred to ...
• Keep water in the pipes by managing water loss and replacing infastructure
• Reduce water demand through universal metering
• Add more supply by constructing the proposed Pākuratahi Lakes 1 and 2
7. Monitor plan and reassess
• Incorporate outcomes of investment process back into the plan
• Annual review of signals, changes in context or supporting information/projections
• Review/update vulnerability assessment
• Review/update adaptive plan and monitoring plan
• Revise investment advice (timing aligned with investment cycles)
Monitoring and triggers
Backwards looking i.e are we still on plan
Forward looking i.e forecasting plan changes
Population (census) Demand side
Water demand
• Peak demand in summer/winter
• Water demand
• Demand in dry/wet years
Leakage reduction New connections/ a proxy variable to forecast demand increase
Metering progress
Yield from new supply options (compared to modelled yield in SYM)
External factors
• Precipitation anomaly
• Groundwater levels
• Temperature increase
• Sea level rise Supply side
Wellington Water would like to acknowledge the involvement and support from Connect Water, National Institute of Water and Atmospheric Research and Dr Judy Lawrence PSConsulting in the development of the DAPP for the Wellington metropolitan water supply.
For further information on DAPP refer to: J. Lawrence & A. Allison (2024) Guidance on adaptive decision making for addressing compound climate change impacts for infrastructure. Deep South National Science Challenge: https://environment.govt.nz/ publications/coastal-hazards-and-climate-change-guidance/
Floods can damage water services operations and assets, with significant clean-up costs. With climate change expected to significantly increase the occurrence and magnitude of flooding events in the future, it is important for water utilities to find ways to:
• Learn from extreme events to build resilience
• Prioritise hotspots that are likely to be impacted
• Leverage existing budgets to support adaptation measures.
On Auckland Anniversary Weekend 2023, a cyclone resulted in major flooding in the city. Over four days, more than a half a year’s worth of rain doused the city, resulting in four deaths and widespread devastation. Water and wastewater assets manged by Watercare were hit hard; pump stations flooded, pipelines were knocked out by slips and reservoirs and treatment plants suffered source water issues. The floods highlighted the need to future proof assets, but funding constraints mean that gold plating such infrastructure will never be possible. To re-instate its assets, Watercare sought cost-effective options and utilised existing operational budgets to minimise the impact of future events, improve asset resilience and long-term risk understanding.
Improving access to assets for future asset reinstatement: Among the infrastructure taken out by the cyclone were several pipelines running across the rugged Hunua and Waitakere ranges. The remote locations, accessible only by old forestry trails, impeded asset reinstatement. Improvements have now been made to access roads near slip-prone assets, such as pipe bridges, to speed up reinstatement times in the future.
Waterproofing pump stations: The floods inundated several Watercare wastewater pump stations. Reinstatement provided an opportunity to waterproof some of the most flood-prone stations. For example, Wairau pump stations received a waterproof door, Glen Dowie pumps were dry mounted and improvements were made to the watertightness of pump housing.
Upgrading wastewater models to prepare for bigger flood events: Following the flooding, it was found that previous flood models, run at a one in 200-year recurrence interval, had correctly identified many flooding hot spots. Auckland Council’s Healthy Water teams have since upgraded and published these flood models and Watercare is investing in similar extensions of its own wastewater models. The new models will give decision makers another source of information on where to target future works.
Watercare’s experience with the flooding has provided lessons that can be broadly applied to assist in flood management:
1. Use flood models to prioritise hotspots within the network. Even the 1:200 and 1:500 event modelling can provide important information to help prepare for future events.
2. Leveraging operational budgets to ensure access and backup systems can be quickly reinstated
3. Weather proofing of flood-prone pump stations during operational upgrades, particularly at low points in the gravity network.
Changes to the world’s climate this century may lead to altered infectious disease incidence and the spread of diseases outside their historical regions. Drinking water can be a pathway for the transmission of infectious disease. Drinking-water treatment processes remove the microorganisms that cause disease. Droughts and floods, which are expected to become more frequent and intense in New Zealand with climate change, will create challenges for the supply of water.
Water supplies can be adversely affected by too much or too little rain, both of which create problems with water quality and quantity. The likelihood of a water supply and its community experiencing such problems can be reduced by identifying the “chain” of events that could lead to these problems and, where possible, taking steps to break the chain.
The Vulnerability Assessment Tool Handbook was produced as part of a research programme on the impact of climate change on infectious diseases in New Zealand. The first iteration of the tool was developed after a workshop with the community in Kaeo. Subsequent versions were never applied, but the tool contains a framework that could be trialled by small communities wishing to mitigate the risks climate change poses to their water supplies.
The handbook provides guidance to small communities and their water suppliers to:
• Assess the vulnerability of their community to being without wholesome water (water that is safe and pleasant to drink) because of heavy rain or droughts
• Understand the characteristics of their water supply and community that are contributing to the vulnerability
• Decide what actions to take to reduce the vulnerability of the water supply.
Though the handbook is targeted at assisting small suppliers, it also has lessons for larger suppliers. Water supply challenges due to climate change, no matter what the scale, will mean that a clear understanding of the infrastructure’s vulnerability is critical. Recognising what is causing that vulnerability and addressing the causes are logical steps for all water suppliers.
For further information on the Water Supply Climate Change Vulnerability Assessment Tool Handbook refer to: https://haifa.esr.cri.nz/ assets/Uploads/Docs/Water-Supply-Vulnerability-Assessment-Tool.pdf
Adaptation
Asset level adaptation
Climate projections / emissions scenarios
Adaptation refers to actions to manage the risk of climate change impacts. The process of adjustment to actual or expected climate and its effects to moderate harm or exploit beneficial opportunities and improve resilience.
Focused on adaptation at a specific asset level, eg, optioneering, design and operational plans for a water treatment plant.
Simulated climate system models based on differing scenarios of the future.
DAPP Dynamic Adaptive Policy Pathways.
Digital twin
A digital twin as a virtual representation of a physical object or system across its lifecycle, using real-time data to enable understanding, learning, and reasoning.
GHG Greenhouse gas(es).
Governance and strategy level adaptation
Hazards
Hydrological cycle
IPCC
Maladaptation
Mitigation
OECD
Portfolio level adaptation
Focused on adaptation at the wider New Zealand water industry strategy and governance level – regulation, strategies, policies, industry guidance, decision making processes.
Climate-related physical events or their potential impacts. Hazards can be acute (rapidly developing climate extremes such as heatwaves)or chronic (slow-onset developments such as rising temperatures).
The continuous circulation of water a from the ground to the atmosphere and back again.
The Intergovernmental Panel on Climate Change (IPCC) is the United Nations body for assessing the science related to climate change.
Actions that may lead to increased risk of adverse climate-related outcomes, including via increased greenhouse gas emissions, increased or shifted vulnerability to climate change, inequitable outcomes or diminished welfare, now or in the future. Most often, maladaptation is an unintended consequence.
Action to reduce emissions that cause climate change (ie, decarbonisation, emissions reductions, emission sinks).
The Organization for Economic Co-operation and Development (OECD) is a forum where the governments of 37 democracies with market-based economies collaborate to develop policy standards to promote sustainable economic growth.
Focused on adaptation at a portfolio of assets level, eg, Wellington’s wastewater network, or a region’s interconnected network of blue-green and grey stormwater infrastructure.
PCRAM Physical Climate Risk Assessment Methodology.
Risk / Physical climate risks (PCRs)
Risk is the likelihood of climate hazards occurring and the associated impact on receptors / assets (equivalent to exposure, likelihood, vulnerability). Dependent on location, funding sources, legislation, policies, author preference, etc, the wording used to define risk varies. Physical climate risks are hazards such as temperature rise and sea level rise.
UNDRR The United Nations Office for Disaster Risk Reduction is the lead agency within the United Nations system for the coordination of disaster risk reduction.
WaterNZ Water New Zealand is the country’s largest water industry body and provides leadership in the water sector through collaboration, professional development and networking.