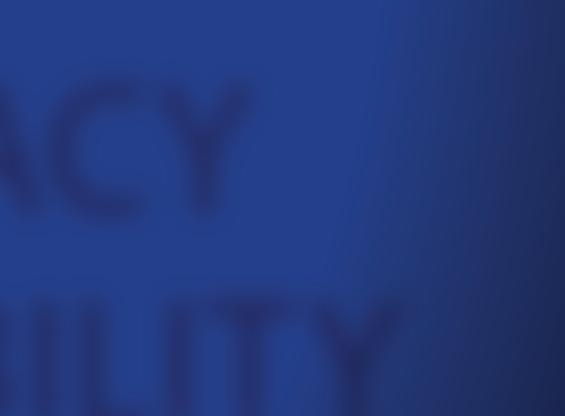
4 minute read
ACCURACY REPEATABILITY CUSTOMIZATION

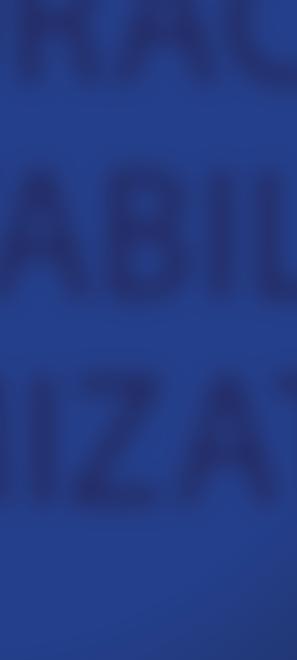
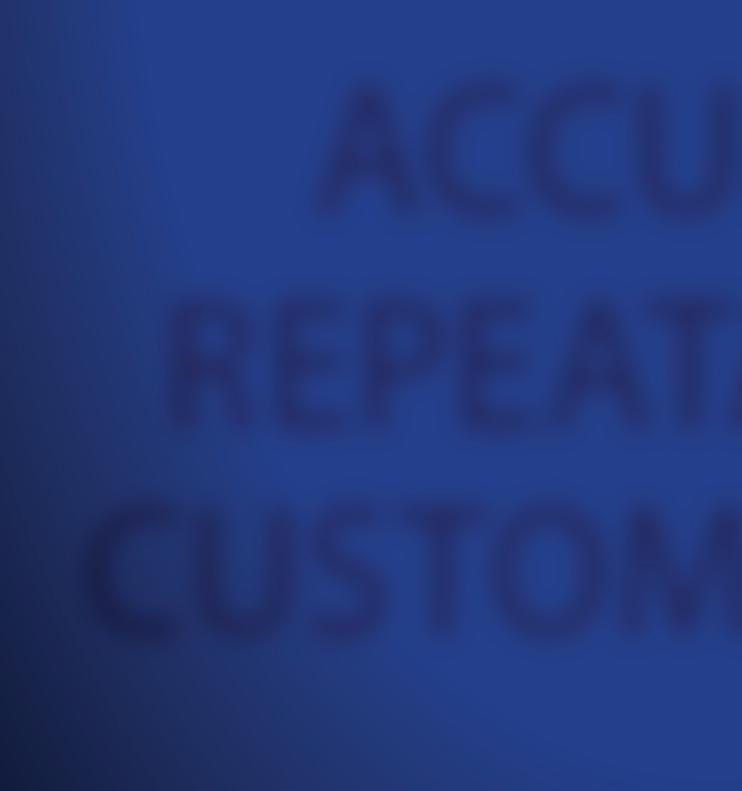
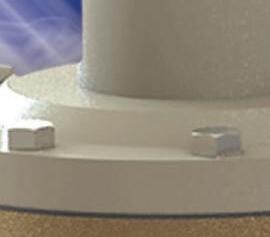
respectively. Magnets made with 1:5 have an energy range of about 15-20 mega-gauss-Oersted (MGOe), and 2:17 magnets have an energy range of about 22-30 MGOe. SmCo magnets are not easily demagnetized. They have low mechanical strength and are expensive but can operate up to 350° C higher than most other types of permanent magnets. They are best suited for extremely high-temperature environments such as downhole oil and gas exploration.
Neodymium iron boron (NdFeB) magnets are also rare earth magnets. NdFeB magnets feature very high energy products, up to 45 MGOe, and have extremely high coercive forces. These magnets can be used up to about 200° C, and their high energy products enable the use of smaller magnets to achieve the same energy product. However, NdFeB magnets have low mechanical strength and are very brittle. They find use in highperformance applications like motive motors in electric vehicles.
Samarium iron nickel (SmFeN) magnets are still mostly under development. They have a saturation magnetization strength comparable to NdFeB but can operate over 400° C. They have superior oxidation and corrosion resistance and do not need cobalt or other heavy rare earth elements, potentially giving them superior sustainability performance. Most applications have operating temperature ranges between -40 and 150° C. All the common permanent magnet materials discussed previously can operate over that temperature range.
Sustainable permanent magnets
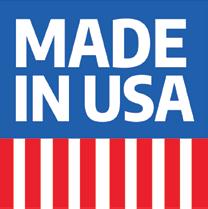
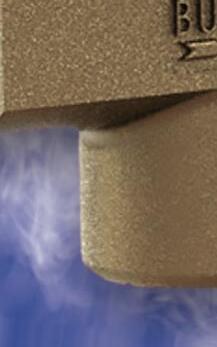
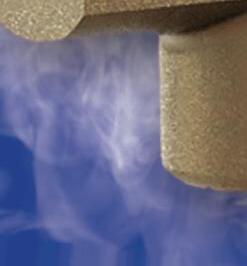
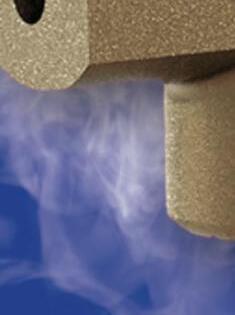
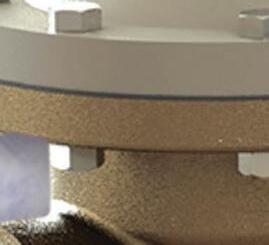
Using rare earths to fabricate highperformance permanent magnets is fraught with sustainability challenges. Most notably, the mining and processing of rare earths produces toxic byproducts, leading to ecological and sustainability challenges with rare- earth mines and refineries. Demand for high-performance magnets with improved sustainability is driving numerous research efforts.
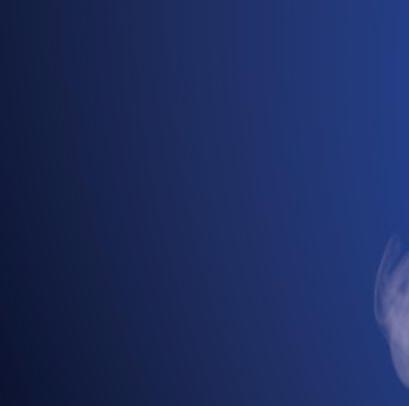
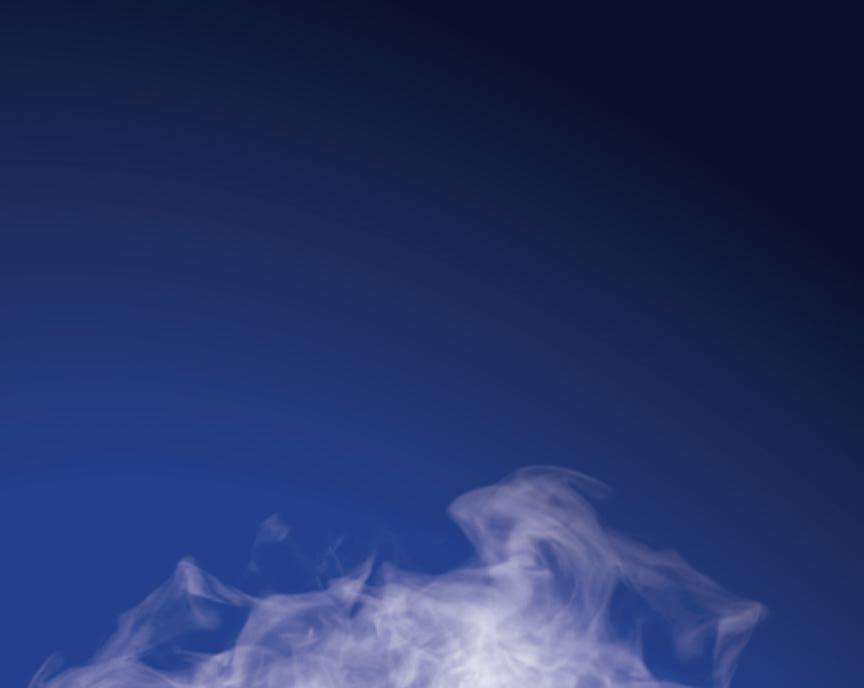
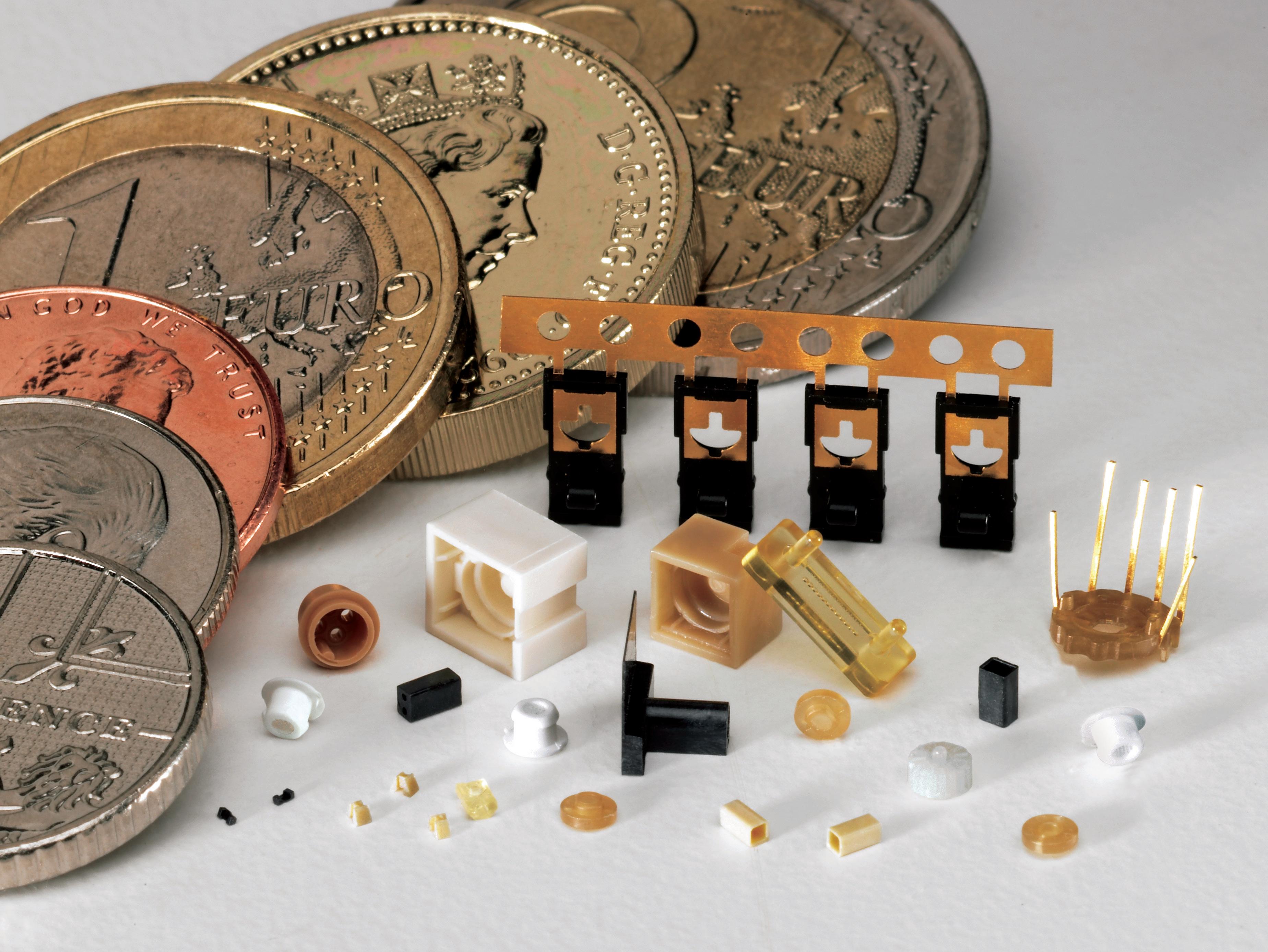
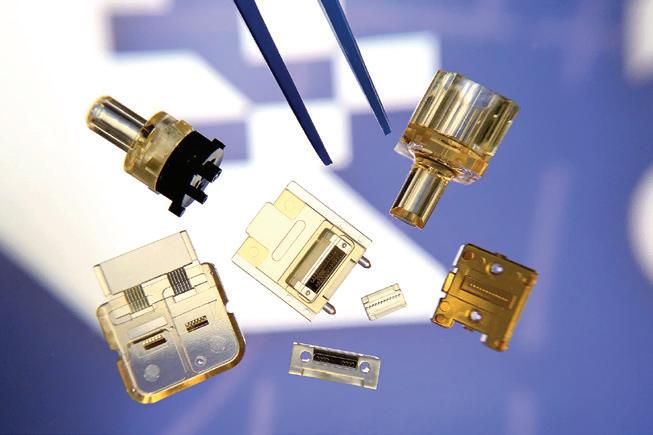
Electromagnets are made with a coil of insulated copper wire, a ferromagnetic or ferrimagnetic material core, and an ac or dc power supply. Current flowing through the wire coil creates a magnetic field. The magnetic core concentrates the magnetic flux and makes a more powerful magnet.
For example, scientists at the University of Leeds are developing a hybrid film from a thin layer of cobalt (which is ferromagnetic) covered with Buckminsterfullerene, a form of carbon. While carbon is not magnetic, when bonded to the cobalt surface, the carbon causes a magnetic pinning effect, preventing the magnetism in the cobalt from changing directions, even in the presence of strong opposing fields. The carbon layer boosts the energy product of the resulting structure by five times at low temperatures. The next step in the research aims to increase the operating temperature of these magnets to room temperature.
In another instance, a team at the Critical Materials Institute at Ames Laboratory has identified two forms of cerium cobalt, CeCo3, and CeCo5, as potential materials for making strong permanent magnets. Although cerium is a rare-earth element, it is abundant and easy to obtain without detrimental environmental impact
EPMs can be controlled to have no net magnetization or produce a strong external magnetic field.
| Wikipedia
and with good sustainability. While CeCo3 is a paramagnetic material, adding magnesium transforms it into a ferromagnet. CeCo5 is natively a strong ferromagnet. Adding copper and iron to CeCo3 and CeCo5 can increase the materials’ ferromagnetic capabilities and potentially make them candidates to replace NdFeB rare earth permanent magnets.
Electromagnets
Electromagnets are made with a coil of insulated copper wire, a ferromagnetic or ferrimagnetic material core, and an ac or dc power supply. Current flowing through the wire coil creates a magnetic field. The magnetic core concentrates the magnetic flux and makes a more powerful magnet.
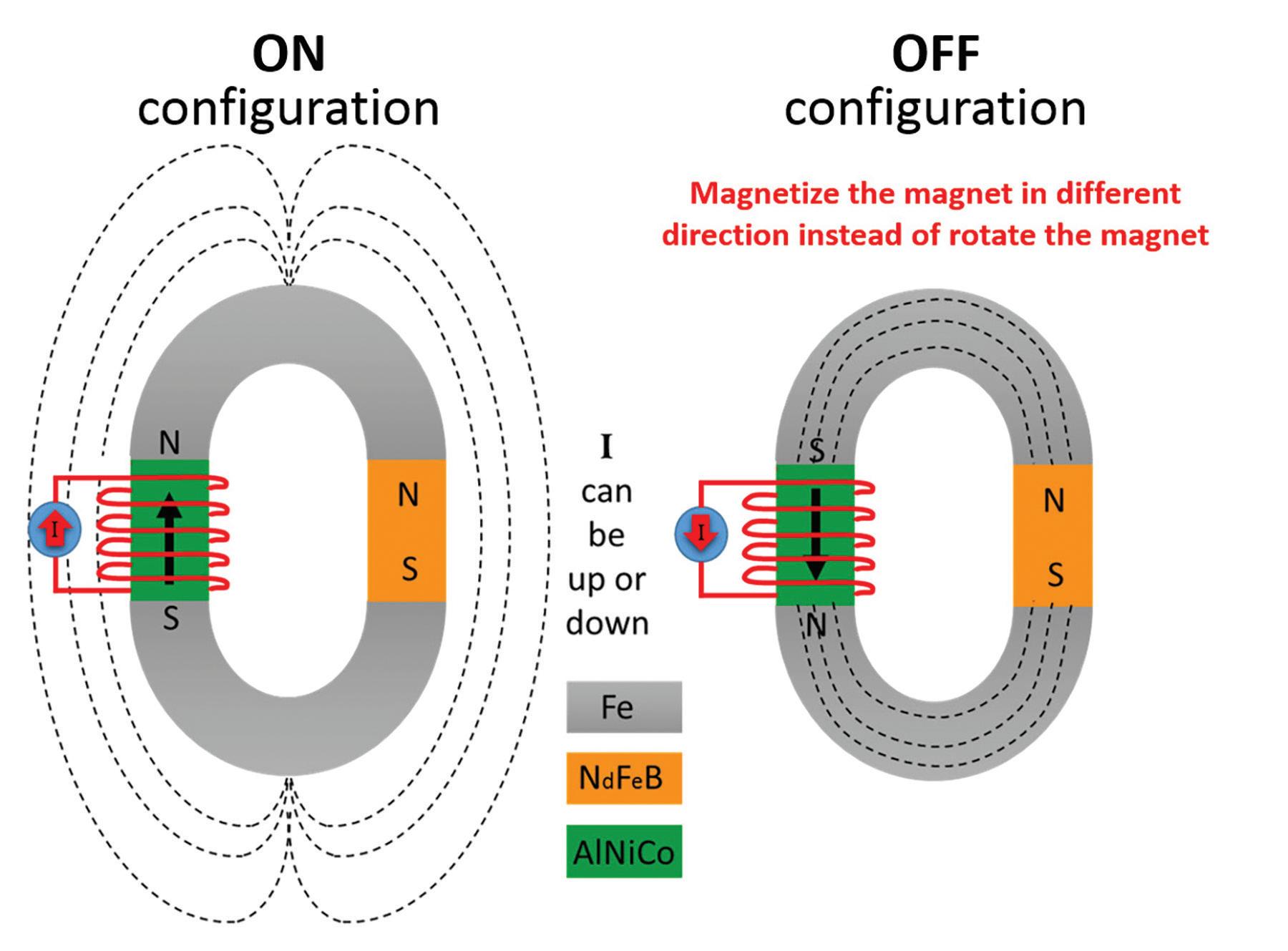
Electromagnets are often used in place of permanent magnets. The strength of the magnetic field can be increased or decreased by increasing or decreasing the current flow in the coil, while the magnetic fields of permanent magnets are fixed. The magnetic field can be reversed by reversing the current flow direction. In addition, an electromagnet can be turned completely off with no remaining field. Disadvantages of electromagnets include the need for a continuous supply of power and their tendency to get hot when current flows are high. Combining an electromagnetic section with permanent magnets can result in improved sustainability.
Electro-permanent magnets for sustainability
An electro-permanent magnet (EPM) consists of two sections: a very hard, high coercivity (H) magnetic material such as neodymium iron boron (NdFeB) with an H of about 1,120 kA/m, and a lower coercivity material such as alnico (AlNiCo) with an H of about 50 kA/m connected with two iron horseshoes. The direction of magnetization in the soft material can be changed by a current flowing in a winding. When the two types of magnetic materials have opposing magnetizations, the EPM produces no net external field across its poles. The field is restricted to the interior of the iron horseshoes. When the magnetizations in the two materials are aligned, the EPM can produce a strong external field.
EPMs can be a form of a programmable or controllable magnet. A common use of EPMs is in industrial holding magnets. When the magnetic circuit is off, it allows for less current (no power flow) and safe holding of magnetic workpieces. When the coil is turned on, the permanent magnetic field is canceled at the holding surface, and the workpiece can be easily removed. These controllable magnetic systems are very energy efficient and sustainable. The current flows only briefly to allow the removal of the workpiece. At all other times, there is no current flow. This design is especially efficient for applications that require long holding times or when loads or workpieces must be safely held, even during a power failure.
EPMs are commonly used for highly energyefficient industrial lifting of ferrous metal objects. Before EPMs were developed, the only way to produce a programmable or controllable magnetic field was to use electromagnets that consume large amounts of power when in operation. The development of powerful NdFeB rare earth permanent magnets made EPMs possible. Researchers are also investigating the possibility of enabling self-building structures using EPMs. DW
References
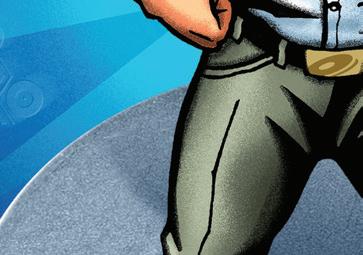
3 Key Parameters to Consider when Choosing a Permanent Magnet, Arnold Magnetic Technologies
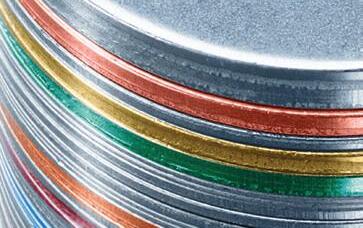
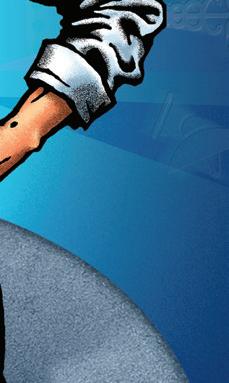
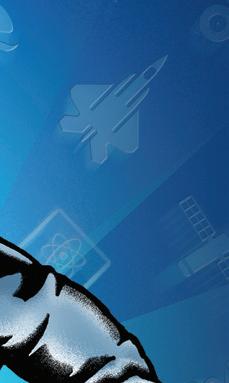
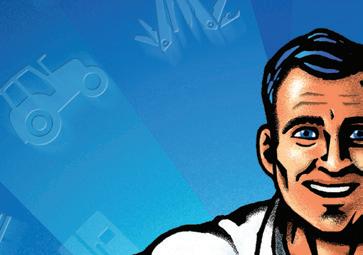
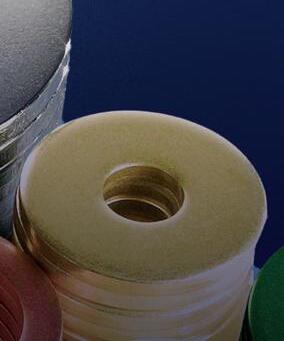
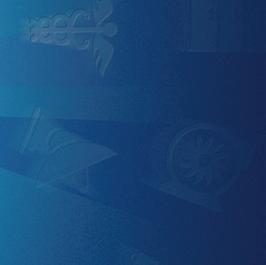
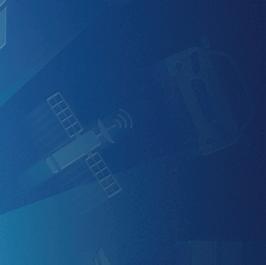
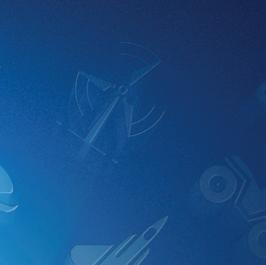
Atomic Cooperation in Enhancing Magnetism: (Fe, Cu)- doped CeCo5, ScienceDirect
Electromagnet, Wikipedia
Electropermanent magnet, Wikipedia
Hope for a new permanent magnet that’s cheap and sustainable, Science Daily
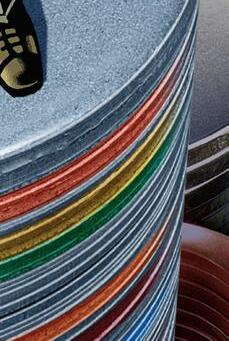
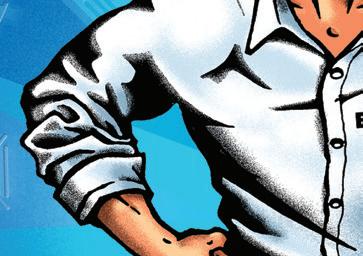
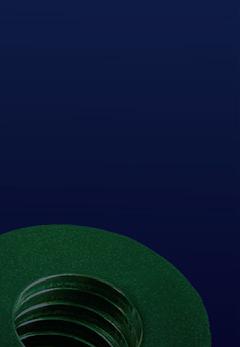
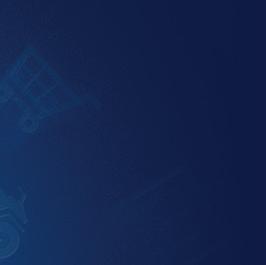
Permanentmagnetic electro holding magnets, Kendrion
Types of Magnets, Javatpoint