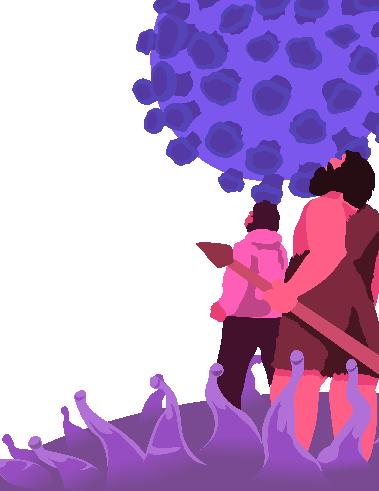
18 minute read
ANCESTRAL IMMUNITY: THE ROLE OF AN ANCESTRAL GENE IN THE FIGHT AGAINST
Ancient Immunity: The Role of an Ancestral Gene in the Fight Against COVID-19
NATALIE DZIKOWSKI `22
Advertisement
Viral infections have posed a challenge to human survival since the Homo sapiens species first evolved. In fact, researchers hypothesize that viruses have been around for billions of years, long before their future human hosts (1). Long before modern medical practices became widespread, the molecules and cells of the human immune system rose to the challenge of fighting viral infections. One of those molecules was OAS1, an enzyme involved in a pathway that inhibits viral replication (2). There is support for the hypothesis that certain variants of this enzyme had been introduced into the Homo sapiens species through mating events with Neanderthals thousands of years ago (3). It has been conserved in humans and primates this whole time, humbly executing its vital role in immunity (4). OAS1 has been investigated for its interactions with many different RNA viruses. Recently, it has made a comeback in scientific literature regarding its role in the COVID-19 immune response. OAS1 is an enzyme, or a protein that helps catalyze chemical reactions, and it is encoded by a gene on chromosome 12 (4). This enzyme has not acted alone; it actually belongs to a whole family of 2’-5’ oligoadenylate synthetases, or OASs. OASs are able to recognize when RNA viruses, such as coronaviruses, trespass into a cell. These viruses are categorized by the type of genetic material they carry, called ribonucleic acid or RNA. It is this genetic molecule, specifically double-stranded RNA, that OAS1 can recognize and bind to when a virus enters a host cell. Once OAS1 is alerted to this threat, it communicates with its sidekick: another enzyme called RNase L. OAS1 quickly catalyzes a reaction that generates 2’-5’ oligoadenylate, a long molecule made up of modified adenosine nucleotides (2). This molecule, in turn, binds to RNase L, inducing a change in its overall structure. This shape change enables it to cut up the double-stranded RNA into small pieces,
Just as p46 may rendering it unusable by the virus. Ultimately, RNase L prevents the virus from replicating have helped our within its host and attacking more host cells (2). In essence, RNase L is the fighter, while ancestors hundreds OAS1 arms it with what it needs to fight. Together, they have executed an extremely imof thousands of portant, yet behind-the-scenes molecular mechanism in the body’s response to viral infections. years ago, it may In fact, this immunoprotective response may have benefitted our ancestors 100,000 be aiding certain years ago, when Homo neanderthalensis — the Neanderthal — and Homo sapiens coexpopulations in isted (3). Neanderthals and Homo sapiens are classified as different species, and their fighting COVID-19 genomes have slightly different versions of the same genes, called variants. Variants in genes infection today. that code for proteins, like OAS1, can actually result in major changes in structure and function of that protein. Depending on the significance of the protein’s function, this may ultimately lead to variations in broader physiological responses. When Homo neanderthalensis and Homo sapiens first diverged into different species, the majority of Neanderthal genetic
variants had been quickly removed from the Homo sapiens genome, and the two species only had a small proportion of variants in common. In fact, the proportion of variants that were still shared by the human and Neanderthal genomes further dropped from 10% to about 3% (3). However, about 100,000 years ago, two major interbreeding events occurred between the species, reintroducing some Neanderthal genetic variants into human DNA. Increased proliferation of RNA viruses may have actually been a driving force for these mating events. Comparative DNA sequencing studies have identified that Neanderthal gene variants on chromosome 12 coding for virus-fighting enzymes were among the few variants that underwent positive selection, remaining in the modern human genome (3). Among these was a Neanderthal version of the gene coding for the OAS1 enzyme, called the p46 variant. It was found to have undergone prominent positive selection, particularly in Eurasian populations (6). Researchers from the University of Montreal studied specific sites of genetic variation, called single nucleotide polymorphisms (SNPs), at
the p46 gene locus. The results of their DNA sequencing studies showed that the SNPs associated with Neanderthal p46 were expressed at frequencies 20% greater than expected in modern, Eurasian human populations (6). Insight into the function of the p46 variant of the OAS1 enzyme compared to other forms may offer more clarity on why this variant has remained in the Homo sapiens genome. This version of OAS1 has higher levels of enzymatic activity due to its ability to synthesize more complex and higher order versions of the 2’-5’ oligoadenylate molecule that activates RNase L (10). Homo sapiens who inherited this form of the enzyme from Neanderthals could have gained a survival advantage when faced with the threat of RNA viruses (6). The process by which reintroduction of this gene variant provided a survival benefit to humans is known as adaptive introgression (6). Just as p46 may have helped our ancestors hundreds of thousands of years ago, it may be aiding certain populations in fighting COVID-19 infection today. A recently published Mendelian randomization study conducted by researchers at McGill University tested whether the level of the p46 variant correlated with COVID-19 outcomes. They analyzed the genome data and circulating enzyme levels of 14,134 COVID-19 patients of European ancestry (7). Their results showed that higher circulating levels of the p46 variant during a non-infectious state were linked to a protective effect against severe COVID-19 outcomes such as death, hospitalization, and the need for mechanical ventilation (7). In fact, each increase in the standard deviation of circulating p46 levels correlated to a 50% reduction in severe COVID-19 outcomes (7). This magnitude of difference shown by the Neanderthal variant has placed OAS1 in the spotlight as a new target for COVID-19 therapies. OAS1 can serve as a valuable biomarker that can be measured to predict outcomes of both viral and autoimmune diseases (2). COVID-19 is not the only virus whose outcomes are impacted by OAS1 variants. In the past, OAS1 has also been investigated in connection to hepatitis B and West Nile virus. It turns out that carrying other versions of the OAS1 gene, like the p48 and p52 variants, is actually associated with negative outcomes for these particular viruses. These variants encode less strong enzymatic activity, unlike p46 (8). For example, genome analyses showed that the alleles encoding the p48 or p52 form of the OAS1 protein were expressed in higher levels in both symptomatic and asymptomatic carriers of West Nile virus, compared to control groups (8). Other genotype analyses found that the presence of these variants is higher in those who develop the autoimmune disorder Sjögren’s syndrome after infection with hepatitis B virus (9). To uncover more about the intricacies of OAS1 polymorphisms, researchers have turned to analyzing the DNA of old-world primates. Overall, it was found that many sites of the OAS1 gene have been conserved, even among different species of primates. In 2016, researchers from the City University of New York found that across 22 different species of oldworld primates, 31 to 32 amino acid sites were conserved, 50% of which are located in the exact spot on OAS1 that is used to bind to viral RNA (4). Since this spot is critical for OAS1’s recognition of viruses, the presence of these conserved sites may have allowed old-world primates to successfully survive the challenges posed by RNA viruses, while other organisms lacking these conserved sequences did not. However, the RNA-binding domain of OAS1 still has highly detailed and unique variation. SNPs and sites of high variation have been commonly observed when sequencing the OAS1 RNA-binding domain in primates. The 54th amino acid in the OAS1 enzyme has been identified as its most highly variable site among primates, and it is directly involved in the enzyme’s recognition of viral RNA (5). How exactly this variation impacts the function of OAS1 is not currently understood in depth, but connecting genome-wide association studies, protein sequencing, and clinical studies can help us understand what aspect of the OAS1 enzyme structure leads to better or worse disease outcomes in humans. Genetic analyses of common ancestors and close evolutionary relatives of humans give us more than percentage similarities of DNA and sequences of adaptively introgressed gene variants. They tell stories about the past challenges that human beings faced, and leave us with questions on how to face similar ones today. The COVID-19 pandemic of the 21st century has been one of the most unpredictable threats to human health, revealing new differences in immune responses and severity of outcomes in different individuals. Understanding the molecular mechanisms behind human adaptations to RNA viruses can teach us a lot about these differences and help lead us to the development of new therapeutic approaches. Hidden in our ancient, evolutionary history are answers to challenges we currently face.
References
1. W. Diehl, et al., Tracking interspecies transmission and long-term evolution of an ancient retrovirus using the genomes of modern mammals. eLife 5, 1-25 (2016). doi: 10.7554/eLife.12704. 2. U. Choi, et al., Oligoadenylate synthase-like (OASL) proteins: dual functions and associations with diseases. Experimental & Molecular Medicine 47, 1-6 (2015). https://doi.org/10.1038/ emm.2014.110. 3. D. Enard and D. Petrov, Evidence that RNA viruses drove adaptive introgression between Neanderthals and modern humans. Cell 175, 360-371 (2018). https://doi.org/10.1016/j. cell.2018.08.034. 4. I. Fish and S. Boissinot, Functional evolution of the OAS1 viral sensor: insights from old world primates. Infection, Genetics and Evolution: Journal of Molecular Epidemiology and Evolutionary Genetics in Infectious Diseases 44, 341-350 (2016). https://doi.org/10.1016/j.meegid.2016.07.005. 5. I. Fish and S. Boissinot, Contrasted patterns of variation and evolutionary convergence at the antiviral OAS1 gene in old world primates. Immunogenetics 67, 487-499, (2015). doi: 10.1007/ s00251-015-0855-0. 6. A. Sams, et al., Adaptively introgressed Neandertal haplotype at the OAS locus functionally impacts innate immune responses in humans. Genome Biol 17, 1-15 (2016). https://doi.org/10.1186/ s13059-016-1098-6. 7. S. Zhou, et al., A Neanderthal OAS1 isoform protects individuals of European ancestry against COVID-19 susceptibility and severity. Nature Medicine 1-24 (2021). https://doi.org/10.1038/ s41591-021-01281-1. 8. J. Lim, et al., Genetic variation in OAS1 is a risk factor for initial infection with West Nile virus in man. PLoS Pathogens 5, 1-12 (2009). doi:10.1371/journal.ppat.1000321. 9. X. Liu, et al., A functional variant in the OAS1 gene is associated with Sjögren’s syndrome complicated with HBV infection. Scientific Reports 7, 1-9 (2017). https://doi.org/10.1038/s41598017-17931-9. 10. H. Di, H. Elbahesh, and M. Brinton, Characteristics of human OAS1 isoform proteins. Viruses 12, 1-16 (2020). doi: 10.3390/v12020152. Images drawn by Isra Ahmed ‘23
The Mysteries of How Non-Neural Components of the Brain Play a Role in Memory Formation
JASMINE KUMAR `22
There are many mysteries surrounding memory formation and consolidation. However, it is well known in the scientific community that neural components of the brain play a very important role in memory formation. More specifically, neural components such as signal transmitting synapses, neuronal branches called axons that transmit signals, and neuronal dendrites that receive signals work together to form memories (1). Neural components form memories in response to a stimulus that releases neurochemicals or neurotransmitters at the synaptic membrane, which increases the synaptic strength of neurons to communicate the memory trace. If the stimulus is strong enough, gene transcription and protein formation will result in the creation of new synapses to support the formation of a memory (2).
INHIBITORY
PREVENTS
NEUROTRANSMITTERS
PRODUCES SYNAPSE
FORMATION
INCREASES
S SURROUN D
PNN GABAERGIC INTERNEURON Although many studies regarding memory demonstrate how neural components of the brain play a major role in formation, recent studies have demonstrated how non-neural components can also play an essential role. For instance, perineural nets (PNNs) are non-neural components of the brain that have been found to assist in the formation of memories. PNNs consist of a lattice-like structure of protein and carbohydrate aggregates that surround the cell body and proximal dendrites of subpopulations of neurons in the central nervous system (CNS) that were discovered by Camillo Golgi in the 1800s (1). Tommasso Pizzoruso and James Fawcett first discovered that PNNs impacted brain plasticity two decades ago, when Fawcett discovered a bacterial enzyme, chondroitinase ABC (chABC), that destroyed PNNs, and combined his findings with Pizzoruso’s. Thus, a sudden revival within the scientific community took place in studying non-neural components such as the PNN after many centuries of MEMORY disregarding them (3). Studies have demonstrated that PNNs can form memories by stunting neural plasticity. Plasticity is the ability of CONSOLIDATION neurons to reorganize or reassemble synaptic connectivity in response to experiences (4). The heightened plasticity period, or critical period (CP), refers to periods in one’s lifetime in which both nature and nurture had molded experiences for an individual that increased their cognitive function, and especially occur during windows of early life (5). Neurons often reach this critical period during childhood and adolescence because the brain tends to have more plasticity and thus is more sensitive to gaining cognitive function and learning new skills as a result of life experiences (5). Janet Werker and Takao Hensch of the Canadian Institute for Advanced Research demonstrated that when certain pathways in the brain are at this CP, the perineuronal net acts as a “physical brake” to prevent synaptic growth (1). One of the many puzzling findings regarding PNNs is that they can form long term memories by preventing further synaptic growth, whereas
neural components aid in memory formation by promoting further synaptic growth instead. Thus opposite to neural components, PNNs play a role in memory formation by restricting brain plasticity and synaptic growth. Studies have determined that the physiological basis for PNNs inhibitory effect is related to their formation around a specific class of neurons. PNNs tend to surround a class of neurons, known as GABAergic interneurons, that produce a calcium-binding protein called parvalbumin (PV). The PV+ GABAergic interneuron then releases inhibitory neurotransmitters that prevent other neurons from firing (3). Thus, the physiological explanation regarding how PNNs disrupt plasticity involves PNNs surrounding these PV+ GABAergic interneurons, as the CP closes, chABC PREVENTS resulting in PV’s inhibitory effects through the creation of a lattice like structure that prevents the formation of new synapses (4). SYNAPSE ALLOWS In a study conducted by Elise Thompson at the University of Oslo, PNNs were removed from rats, leading to the inability to recall a remote fear memory (6). The rats were exposed to a conditioned stimulus of a white FORMATION light, and an unconditioned stimulus of a foot shock, which stimulated the formation of a fear memory. Following four weeks of training to pair the unconditioned and conditioned stimuli, the PNN in the lateral secondary visual cortex (V2L) was degraded using injections of chABC. Results demonstrated that the degradation of the PNN disrupted the recall of the remote unconditioned stimulus, or fear memory. The same experiment was repeated to test recent memory recall, where chABC was injected into V2L one day after training and the animals were allowed to recover for six days before testing again. In this case, results indicated that the degradation of PNN using chABC did not influence the recall of recent fear memory. Thus, the results indicate that PNNs are involved in remote memory recall, but not in recent memory recall, leading researchers to conclude that PNNs play an especially important role in long term memory formation (6). Oscillatory activity in the brain was measured to determine if a stimulus is firing, resulting in greater brain activity, or if it is inhibited, resulting in smaller activity. Synchronized oscillatory activity is a known physiological correlate of memory retrieval and indicates communication between V2L and basolateral amygdala (BLA). Within Thompson’s study, the firing of PV+ neurons was associated with synchronized oscillation activity between the V2L and BLA regions of the brain. Synchronized oscillation activity indicated an increase in PV+ firing, blockage of synaptic growth, and restriction of plasticity due to the PNN’s forming a memory. Synchronized oscillatory neural activity between V2L and BLA was collected for both the remote control group and the remote experimental fear memory recall group. Results demonstrated that the control group had synchronized oscillatory activity between V2L and BLA, with a peak at 7Hz, and thus could recall the fear memory remotely. However, the experimental group that was injected with chABC and tested for recall of remote fear memory could not recall the remote memory since there was no synchronization in oscillatory activity that is associated with a decline in PV+ firing. Lastly, the rats tested for recent memory retrieval also demonstrated synchronized oscillatory activity during the conditioned stimulus presentation (6). Thus, this study supported the physiological explanation that the maturation of PV+
Figure 2
The influence of PCC absence on remote fear memory recall is seen when chABC is injected during the conditioned place preference (CPP) procedure. GABAergic interneurons in combination with PNN formation around them towards the end of the CP results in the blockage of further synaptic growth. Research conducted by Megan Slaker and colleagues demonstrates that the function of PNNs in memory formation may be utilized in the development of novel therapeutic remedies for treating drug addiction. Slacker and her colleagues used a conditioned place preference (CPP) procedure to create cocaine addiction related CPP memories within rats that allowed them to form a memory of a specific location in which cocaine Future research on PNNs’ cravings occur. More specifically, rats underwent a CPP procedure within impact on memories re- different plexiglass compartments, in which there was one group conditioned mains necessary and has to prefer a cocaine paired compartment and another group conditioned to prefer a the potential to lead to de- saline paired compartment. After the CPP procedure, the PNNs from their medial velopments in novel drug prefrontal cortex were removed utilizing chABC. Results demonstrated that the addiction therapies, mem- removal of PNNs from the prelimbic region of male adult rats impairs the ory restoration therapies formation and reconsolidation of the cocaine induced CPP memory (7). These for Alzheimer’s and other results highlight that research regarding the role of PNNs within memory forms of tauopathy, and formation and even reconsolidation can be utilized to develop novel therapeutic more. remedies for drug addiction in the future. Memory reconsolidation, however, is not the same as memory formation due to differences in the physiological and biological mechanisms between reconsolidating a memory and forming a memory. In fact, memory consolidation is a part of forming a memory,
and memory reconsolidation just refers to the reactivation of a consolidated memory. However, this reactivation brought about by reconsolidation can change or even delete the initial memory (8).
Another research study led by Sujeong Yang and his colleagues affiliated with the University of Cambridge demonstrated PNNs’ role in memory formation and took advantage of their role to treat a neurodegenerative disease known as tauopathy in mice. Alzheimer’s disease is the most widespread form of tauopathy that is known (9). More specifically, tauopathy results from intracellular deposits of the tau protein. Under pathological conditions, the tau protein can undergo post translational modifications, like being phosphorylated or acetylated, which can damage neurons (10). A transgenic mouse model was utilized to induce tauopathy within these mice by introducing mutant P301S tau in their neurons. Additionally, another transgenic mouse model was created by introducing an adeno-associated virus that expressed P301S tau in the perirhinal cortex, a region associated with object recognition (OR) memory. There was a 15% loss of neurons in the first mouse model compared to its control group, and a 26% loss of neurons in the second mouse model compared to its control group. Although this neuronal loss is considered to be quite low in both models, it was still statistically significant compared to their controls. Despite this low neuronal loss observed in both mouse models, there was a major loss of OR memory observed in both models. A week later, chABC was used to reactivate plasticity by reducing the PNNs in the perirhinal cortex, and this injection of chABC restored OR memory in both models (9). As demonstrated in Slacker and Thompson’s studies, a loss in PNNs resulted in impairment in memory formation through the inability of memory recall because PNNs were no longer there to stunt synaptic transmission and plasticity, which promotes memory formation. However, in Yang’s study a loss in PNNs, or the reactivation of plasticity and restoration of synaptic growth and transmission, had the opposite effect on memory formation where a loss in PNNs was associated with OR memory recall. Yang hypothesized that this loss of PNNs allowed for synaptic growth and plasticity to be restored and that may have improved memory formation (9). These contradicting findings in Yang’s, Slaker’s, and Thompson’s studies indicate that there are many mysteries surrounding how exactly PNNs impact memory formation. It is important to consider that Slaker and Thompson focused on psychological memory, whereas Yang focused on memory related to neurodegenerative diseases, which could possibly influence these contradicting findings. Future studies comparing the effects of PNN removal in various regions of the brain on memory formation could help further elucidate the explanation for these contradictions. Overall, future research on PNNs’ impact on memories remains necessary and has the potential to lead to developments in novel drug addiction therapies, memory restoration therapies for Alzheimer’s, and other forms of tauopathy.
1. S.Ackerman, The nets around the neurons. American Scientist, (2021). 2. G. Miller, How our brains make memories. https://www.smithsonianmag.com/science-nature/ how-our-brains-make-memories-14466850/, (2010). 3. H.Shen, Core concept: perineuronal nets gain prominence for their role in learning, memory, and plasticity. PNAS 115, 9813-9815 (2018). doi:10.1073/pnas.1815273115. 4. J.Duncan, et al., The potential of memory enhancement through modulation of perineuronal nets. British Pharmacological Society 176, 3611-3621 (2019). doi: 10.1111/bph.14672. 5. R. Reh, et al., Critical period regulation across multiple timescales. PNAS 117, 1-6 (2020). doi: 10.1073/pnas.1820836117. 6. E.Thompson, et al., Removal of perineuronal nets disrupts recall of a remote fear memory. PNAS 115, 607-612 (2018). doi:10.1073/pnas.1713530115. 7. M. Slaker, et al., Removal of perineuronal nets in the medial prefrontal cortex impairs the acquisition and reconsolidation of a cocaine-induced conditioned place preference memory. The Journal of Neuroscience 35, 4190-4202 (2015). doi: https://dx.doi.org/10.1523%2FJNEUROSCI.3592-14.2015. 8. L. Nadel, et al., Memory formation, consolidation, and transformation. Neuroscience and Biobehavioral Reviews 36, 1640-1645 (2012). doi: 10.1016/j.neubiorev.2012.03.001. 9. S. Yang, et al., Perineuronal net digestion with chondroitinase restores memory in mice with tau pathology. Experimental Neurology 265, 48-58 (2015). doi: 10.1016/j.expneurol.2014.11.013. 10. G. Hoglinger, G. Respondek, and G. Kovacs, New classification of tauopathies . Revue Neurologique 174, 664-668 (2018). doi: 10.1016/j.neurol.2018.07.001.
Images drawn by Isra Ahmed ‘23