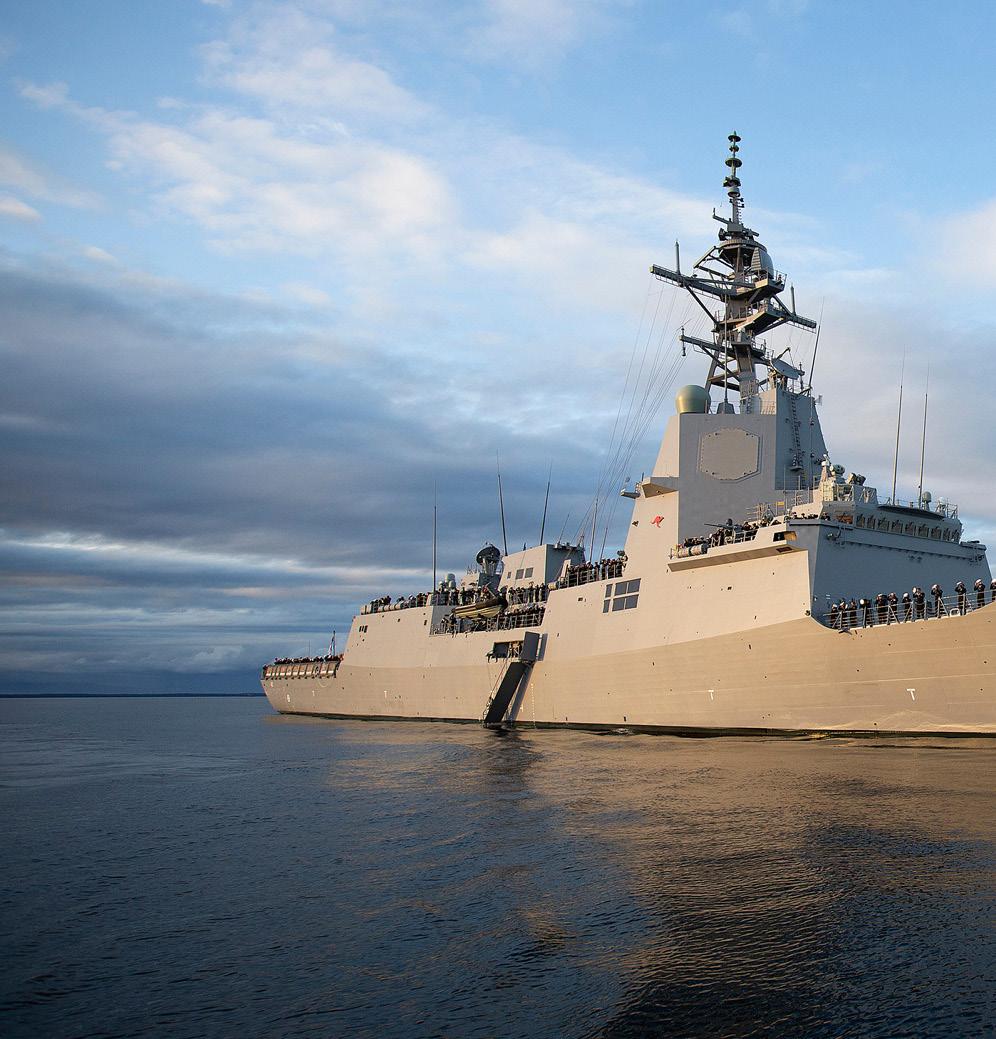
15 minute read
OFFBOARD ONBOARD Cooperative targeting
from ADBR May-June 2020
by adbr5
OFFBOARD ONBOARD
Emerging long-range, high-speed threats are placing a premium on networking to enable disparate sensors and weapons to detect, track and engage.
Advertisement
In the ongoing global defence buzzword bingo tournament, few phrases score better than ‘collaborative targeting’. Augmenting endless industry and program PowerPoint presentations, this missive is usually flanked by pictograms where outsized platforms stalk the airspace, coastline and territory of an unidentifiable nation linked together with brightly-coloured arrows.
But search for a workable definition of the phrase and one is disappointed. Even the bible of military phraseology – the US Department of Defense’s Dictionary of Military Terms – contains no definition. Instead, one is driven to compose one’s own interpretation, which for the purpose of this article, will define collaborative targeting as the use of offboard and onboard sensors, communications systems, kinetic or electronic effectors to engage targets originating from over-the-horizon ranges.
ENGAGEMENT
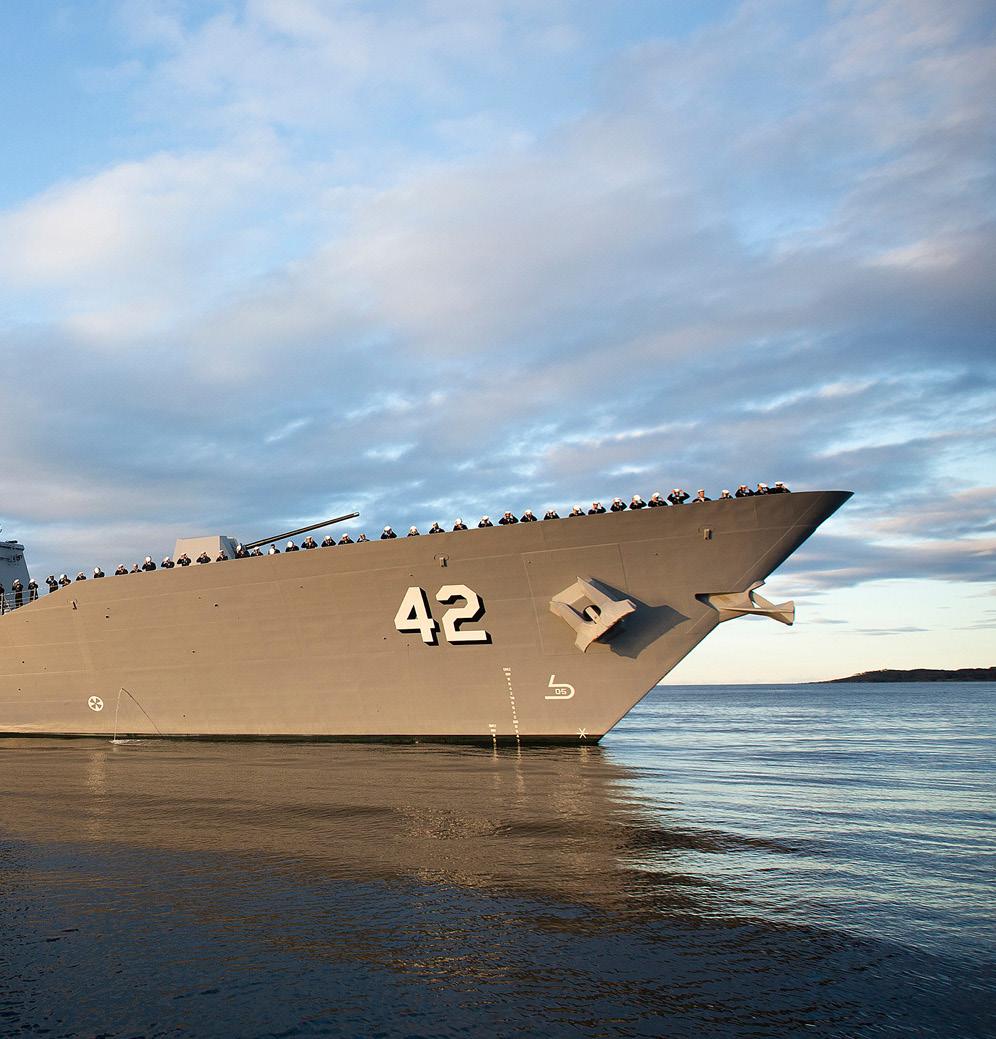
BY DR THOMAS WITHINGTON
The RAN’s ‘Hobart’ class employs the AN/USG-7B variant of the CEC hardware allowing these ships to participate in the US Navy’s Cooperative Engagement Capability. RAN IN THE BEGINNING Collaborative targeting is not new. Indirect fire is arguably its ancestor, and the desire to blindly engage an enemy while remaining unseen is as old as warfare itself. Milton Wylie Humphreys took an important step towards the formalisation of indirect fire during the American Civil War of 1861 to 1865.
Humphreys enlisted in the Confederate Army in March 1862, initially serving with Bryan’s Battery of the 13th Virginia Light Artillery. It was during engagements with the Union Army in West Virginia that Humphreys had the opportunity to test the theories of indirect fire which he had been formulating. During one attack on a Federal fort he calculated the elevation required for his guns to shoot over pine trees shielding his position from visual detection based on his knowledge of the target’s range from his guns, and the range of his own weapons.
This was an exercise in trigonometry. Humphreys was able to visualise his engagement of the fort as an obtuse triangle. The triangle’s horizontal side resting on the ground was the range from the gun to the fort, the hypotenuse was the trajectory of the shell as it left the gun.
The magic number for Humphreys was the third side of the triangle from where the shell met its maximum range, lost energy and would drop onto the target: Assuming that the fort was a range of 4km from Humphreys’ guns, and that his cannons had a maximum range of 5km, he would need to ensure that his cannons had an elevation of circa 38 degrees to give the shells a chance of falling onto the fort. To provide further assistance, Humphreys positioned an artillery observer on a nearby hill who could see the target and advise on adjustments to the gun’s positions to improve the accuracy of the fire.
The historical record says that Union troops experienced relatively light losses of two killed, nine missing and seven wounded. The main damage, however, may have been psychological; union troops could not determine where the fire was coming from. Humphreys had effectively devised indirect fire in its modern form, and even used the term to describe his action. Yet he was modest, saying “I claim no credit for the invention, the thing is so obvious. In fact, if I invented it, I did not do it at Fayetteville, but in my daydreams when I was about eight years old.”
THE MISSILE AGE Collaborative targeting takes the principles pioneered by Humphreys and others, such as the Russian Army’s Lieutenant Colonel KG Guk who, in 1882, took the concept a step further when he published Field Artillery Fire from Covered Positions.
The principle has a similar approach: to engage targets which may be out of detection and tracking range either visually, optronically or electromagnetically, of the platform performing the kinetic engagement. Collaborative targeting has three components; the sensor detecting the target, the platform or effector engaging the target, and a battle management/command and control component to coordinate the engagement and the communications necessary to knit these elements together.
While artillery was the main driver for the development of indirect fire techniques, it is the threat posed by ballistic and hypersonic missiles which is arguably spurring the development of collaborative targeting approaches, and nowhere ‘Humphreys had effectively devised is this arguably more apparent than the naval domain. indirect fire in its modern form’
Ballistic and hypersonic missiles pose unique challenges as they can travel long distances at high speeds. Ballistic missile speeds vary according to the phase of their flight. They are travelling at their slowest during the boost phase immediately after launch when they are flying upwards through the atmosphere. They will accelerate as they reach their mid-course phase as the missile reaches apogee, and then begins its fall towards earth. Finally, the terminal phase is when the warhead has detached from the rest of the missile and is descending rapidly towards its target.
A Short-Range Ballistic Missile (SRBM) which will typically have a range of under 540nm/1,000km and may reach average speeds of 2,915kts. Medium-Range Ballistic Missiles (MRBM) can reach distances from 540nm to 1,619nm/3,000km and speeds of 4,859kts, and Intermediate Range Ballistic Missiles (IRBMs) can hit speeds of 9,719kts across ranges of 1,619nm to 2,970nm/5,500km.
By far the fastest are Intercontinental Ballistic Hypersonic missiles are Missiles (ICBMs) with ranges above 2,970nm which can reach velocities of 12,959kts. emerging as major threats, particularly in the maritime domain. The ability to network Regarding ICBMs, a Russian RS-24 Yars/ Topol-MR (NATO reporting name SS-27 Mod.2/ multiple platforms, sensors and weapons to combat them is essential. US DOD
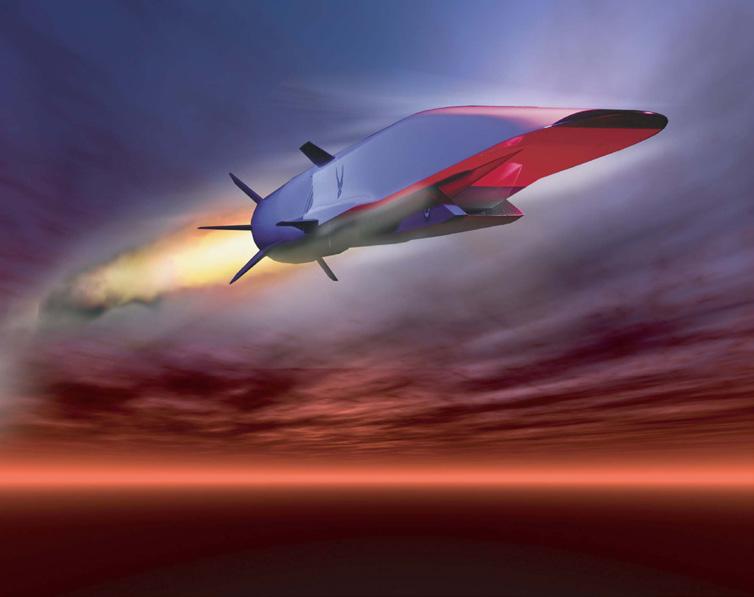
Russia’s 9K720 Iskander (NATO reporting name SS26 Stone) can cover long distances in a comparatively short time; precisely the sort of threats that collaborative targeting techniques could be optimised for. VITALY KUZMIN
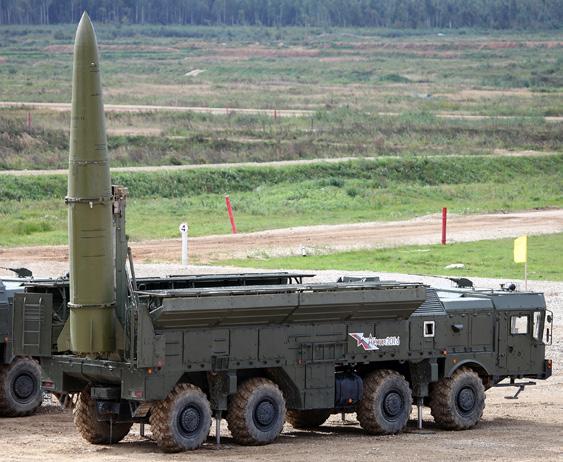
SS-29) missile fired from Teykovo in western Russia, would take a mere six minutes to travel the 1,442nm/2,671km to London.
The situation is similarly vexing regarding hypersonic threats. Hypersonic missiles can reach speeds over 3,333kts. While not quite matching the velocities of their ballistic missile counterparts, they can nonetheless, achieve impressive ranges. Russia’s NPO Mashinostroyeniya 3M22 Zircon (NATO reporting name SS-N-33) reportedly can hit speeds of 5,953kts across distances of up to 270nm/500km. If such reports are true, this would mean that the 3M22 could hit a target at its maximum range in just two minutes and 43 seconds.
These timings provide a guideline as to how quickly one must navigate Colonel John Boyd’s famous OODA Loop (Observe, Orientate, Decide, Act) to detect a ballistic or hypersonic missile and engage it successfully. As one can see, in such scenarios time is of the essence, but is not the only complicating factor.
To successfully intercept such a target, rapid detection and tracking is essential. This translates
Missile
SRBM
MRBM
IRBM Maximum range
540nm
1,619nm
2,970nm
Speed
2,915kts
4,859kts
9,719kts
Flight time for maximum range
11 minutes
20 minutes
18 minutes
Hypothetical flight times of different ballistic missile types based on their maximum range and average speed. into the ability to detect the target as soon after launch as possible, and here things get complicated. Ballistic missiles are typically detected using two mechanisms – satellite-based infrared sensors which spot the hot exhaust plume of the missile as it launches and begins its flight, and ground-based air surveillance radars or their ship-based counterparts.
OTH radars can ‘see’ distances of thousands of nautical miles and are able to look deep into hostile territory to spot a missile launch. This is achieved by using High Frequency transmissions (3MHz to 30MHz) which cannot penetrate the ionosphere, an ionised layer of the atmosphere between 60km and 1,000km above the Earth’s surface. Instead they are bounced back to the ground beyond the curvature of the Earth, hitting flying objects like ballistic missiles as they go. The transmissions bounce back to the radar as echoes, registered as targets and tracked.
However, OTH radars such as the Australia’s Jindalee Operational Radar Network (JORN), which transmits on frequencies of 5MHz to 30MHz to provide instrumented ranges of between 540nm and 1,619nm, are large contraptions which must be housed at a fixed site. For example, JORN uses four transmitters located in Queensland, the Northern Territory, and Western Australia. This means that fixed OTH radars can only look at a specific area, typically where a threat is expected to come from – in JORN’s case the northern and north-western approaches to Australia.
Warships offer a perfect platform for a deployable radar which can detect ballistic missile launches as and when they occur near their point of origin. Ideally, the best time to intercept a ballistic missile is when it is at its slowest and lowest during the boost phase. Lockheed Martin’s AN/SPY-1 series of S-band (2.3GHz to 2.5GHz/2.7GHz to 3.7GHz) naval surveillance radars offer instrumented ranges in excess of 100nm/185km, while Thales’ SMART-L L-band (1.215GHz to 1.4GHz) radar takes this detection range to 1,079nm/2,000km for ballistic missiles.
SCENARIOS Collaborative targeting not only assists with ballistic missile interceptions, it is also a valid technique for engaging conventional air-breathing targets.
Consider this scenario: A Royal Australian Navy Hobart class destroyer is underway in international waters off the coast of the Democratic People’s Republic of Korea (DPRK). Suddenly, her AN/SPY1D radar detects several aircraft which have taken off from known Korean People’s Army Air Force (KPAAF) airbases. Anti-Air Warfare (AAW) officers onboard watch their radar screens.
It soon becomes apparent that a large armada of aircraft is assembling over the DPRK and is heading south-west into the Yellow Sea. The AAW officers are now closely watching the aircraft which have switched
off their transponders and have turned on a south/ south-west heading towards Taiwan. To make matters worse some of the aircraft are attempting crude, yet largely ineffective, electronic warfare (EW).
For all intents and purposes the intentions of the KPAAF aircraft appear hostile, but as they are flying in international airspace and, with the exception of deactivating their transponders and performing some half-hearted EW, have yet to perform a hostile act. It is impossible to say at this stage whether the KPAAF’s actions are aggressive or merely an exercise, which prevents the destroyer from being authorised to take any offensive action against the aircraft.
To the south a pair of US Navy Arleigh Burke Flight-III destroyers is underway in Taiwanese territorial waters. The ships have just been performing exercises with their Republic of China Navy counterparts, despite predictable opposition from the People’s Republic of China (PRC). They have continued to patrol in Taiwanese waters with the blessing of the Taiwanese government, while demonstrating US commitment to the bilateral Taiwan Relations Act.
Both the US destroyers are equipped with Raytheon’s AN/SPY-6(V)1 S-band/X-band (8.5GHz to 10.68GHz) naval surveillance radar. The instrumented range for the AN/SPY-6(V)1 has not been published, yet some reasonable calculations in the public domain have speculated that it could detect and track a ballistic missile target at distances of almost 300nm/550km.
For now the KPAAF aircraft remain outside the gaze of these radars. Nonetheless, the US ships receives notification from the Hobart class that the aircraft are heading in their direction. This gives the crew valuable additional moments to prime their radars to detect the aircraft and ready the ship’s Raytheon RIM-66 SM2 and RIM-162 ESSM series SAMs to intercept the jets.
CEC NETWORK This fictious, though plausible, scenario typifies the philosophy behind collaborative targeting. It is this philosophy which is at the heart of the US Navy’s Cooperative Engagement Capability (CEC). The CEC is a communications network which allows aircraft and warships to share their radar data with one another in real time. This improves the detection of air and missile targets, and the coordination of the response to such threats.
Navies can already share track information regarding targets between warships and aircraft using protocols such as the Link-11 (2MHz to 29.9MHz, 225MHz to 399.975MHz) and Link16 (960MHz-1.215GHz) tactical datalinks used throughout NATO and allied forces.
While the exchange of track and other tactical data is intrinsic to deepening theatre-wide situational awareness and improving command
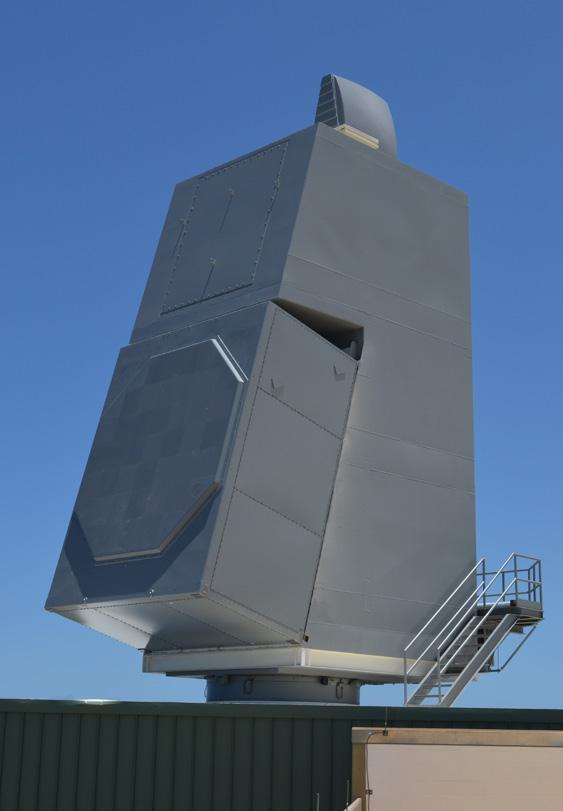
and control, CEC takes this a step further by An example of the AN/ enabling the radar picture to be shared between platforms, providing an even deeper level of SPY-6 is seen here undergoing tests in Hawaii. The radar represents a comprehension. The architecture of the CEC would merit an notable enhancement in US Navy anti-ballistic missile capabilities. RAYTHEON article by itself, although it is possible to summarise how the capability works. Raytheon is leading the initiative which will see an array of surface vessels, aircraft, and land-based radars belonging to the USN and USMC equipped with the AN/USG-2/3/4 CEC Common Equipment Set (CES). The AN/ USG-3 will equip the US Navy’s Northrop Grumman E-2D Hawkeye AEW&C aircraft, accompanying its Lockheed Martin AN/APY-9 Ultra High Frequency (UHF: 420MHz to 450MHz/890MHz to 942MHz) radar letting the E-2C share its air and maritime radar picture in real time.
Likewise, surface vessels will use the AN/ USG-2 to convert and share their radar imagery, for example allowing a Ticonderoga class cruiser to see the radar picture generated by the Raytheon AN/SPY-3 X-band multifunction ‘The RAN...to date is the only force beyond the radar furnishing the USN’s new Gerald Ford class aircraft carriers. USN to have received the CEC capability’
Part of Australia’s JORN radar is shown here. This complex of several large arrays can look thousands of miles beyond Australian territory but is neither mobile nor transportable. BAE SYSTEMS Even land-based radars such as the USMC’s Northrop Grumman AN/TPS-80 G/ATOR (Ground/ Air Oriented Task Radar) S-band ground-based air surveillance radar will be linked into the CEC network using the AN/USG-4 kit.
Capabilities used by other services can also plug into the CEC. The US Army’s Raytheon Joint Land Attack Cruise Missile Defence Elevated Netted Sensor System – better known as JLENS – is an aerostat-based Very High Frequency (133MHz to 144MHz/216MHz to 225MHz) airborne surveillance radar and X-band fire control radar. It is capable of detecting surface-to-surface cruise missiles, lowflying aircraft, and surface vessels at ranges of up to 300nm/550km, and can connect to the network using the AN/USG-5 system.
These CESs possess the necessary hardware and software to form the CEC network in the form of the Cooperative Engagement Processor (CEP) which turns the platforms’ analogue radar data into secure traffic, which is then sent to the equipment’s Data Distribution System (DDS). While there appears to be no specific details in the public domain, it is reasonable to assume that this radar imagery is then shared with other platforms across secure V/UHF (30MHz to 3GHz) wideband line-ofsight datalinks.
The DDS also receives radar data from other CEC-equipped platforms in the network, converts this information from digital to analogue formats which can be viewed by the crew in the operations room. Such is the quality and timeliness of the radar imagery that can be shared across the CEC, that the US Navy says it will be sufficient to enable the offboard targeting of weapons against OTH threats.
The RAN has performed a series of tests to evaluate the performance of the CEC architecture outfitting the Hobart class vessels and their USN counterparts and, to date, is the only force beyond the USN to have received the CEC capability.
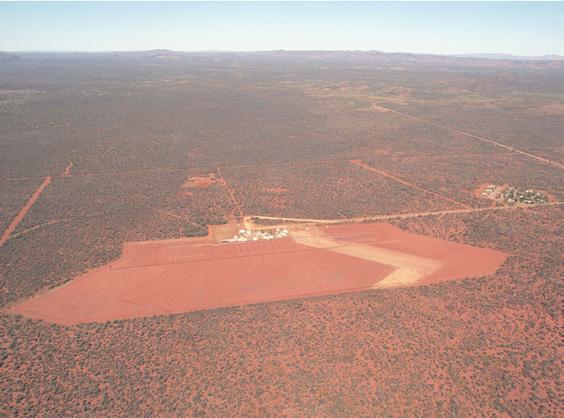
During 2019 both HMAS Hobart and HMAS Brisbane participated in live fire surface-to-air missile tests involving US Navy warships off the US west coast, demonstrating the abilities of these vessels to send and receive radar imagery over the CEC.
During a test in October 2019 HMAS Brisbane performed a live SAM test using data sent remotely over the CEC, while in 2018 HMAS Hobart demonstrated her ability to send and receive radar imagery between her and the USS John Finn using the CEC during exercises in the US Pacific Test Range off Hawaii. The Hobart class uses the AN/USG-7B variant of the AN/USG-2 terminal earmarked for non-US naval vessels.
Other platforms in the Australian armed forces earmarked to receive CEC terminals include the RAAF’s Boeing E-7A Wedgetail AEW&C aircraft, plus the RAN’s planned nine Hunter class frigates.
NEXT STEPS The RAN and US Navy are not the only two forces looking to exploit the advantages collaborative targeting brings. The French Navy is moving ahead with its Veille Coopérative Navale (VCN/Naval Cooperative Surveillance) project.
Much like CEC, VCN will let aircraft and vessels in a task group share their radar pictures for the engagement of OTH targets using offboard sensors and effectors. Radar imagery will be carried across the French Navy’s RIFAN-2 (Réseau IP de la Force Aero Navale/Naval Aviation and Internet Protocol Network) V/UHF communications.
Trials of the VCN involving Horizon and Aquitaine class frigates and the aircraft carrier Charles de Gaulle have already been performed. The French Navy expects the VCN to be declared fully operational onboard these ships by 2022, with the capability rolled out across the rest of the fleet thereafter.
Likewise, the Indian Navy is moving ahead with a collaborative targeting initiative and, in 2019 performed tests off India’s west coast during which Israel Aerospace Industries’ (IAI) Barak-8 active radar homing SAMs were launched using radar data shared between the navy’s Kolkata class destroyers INS Chenai and INS Kochi, with one ship passing radar imagery from their IAI EL/M-2248 S-band naval surveillance radar to the other.
Basketball legend Michael Jordan once said that “talent wins games, but teamwork and intelligence wins championships.” Jordan’s maxim is as applicable to combat as it is to the court. Individual platforms, sensors and weapons will always face limits in their ability to engage high-speed threats with OTH ranges. Bringing these platforms, sensors and weapons together immediately makes them stronger than the sum of their parts. Initiatives such as the CEC, and its counterparts, mark an important step in this direction.