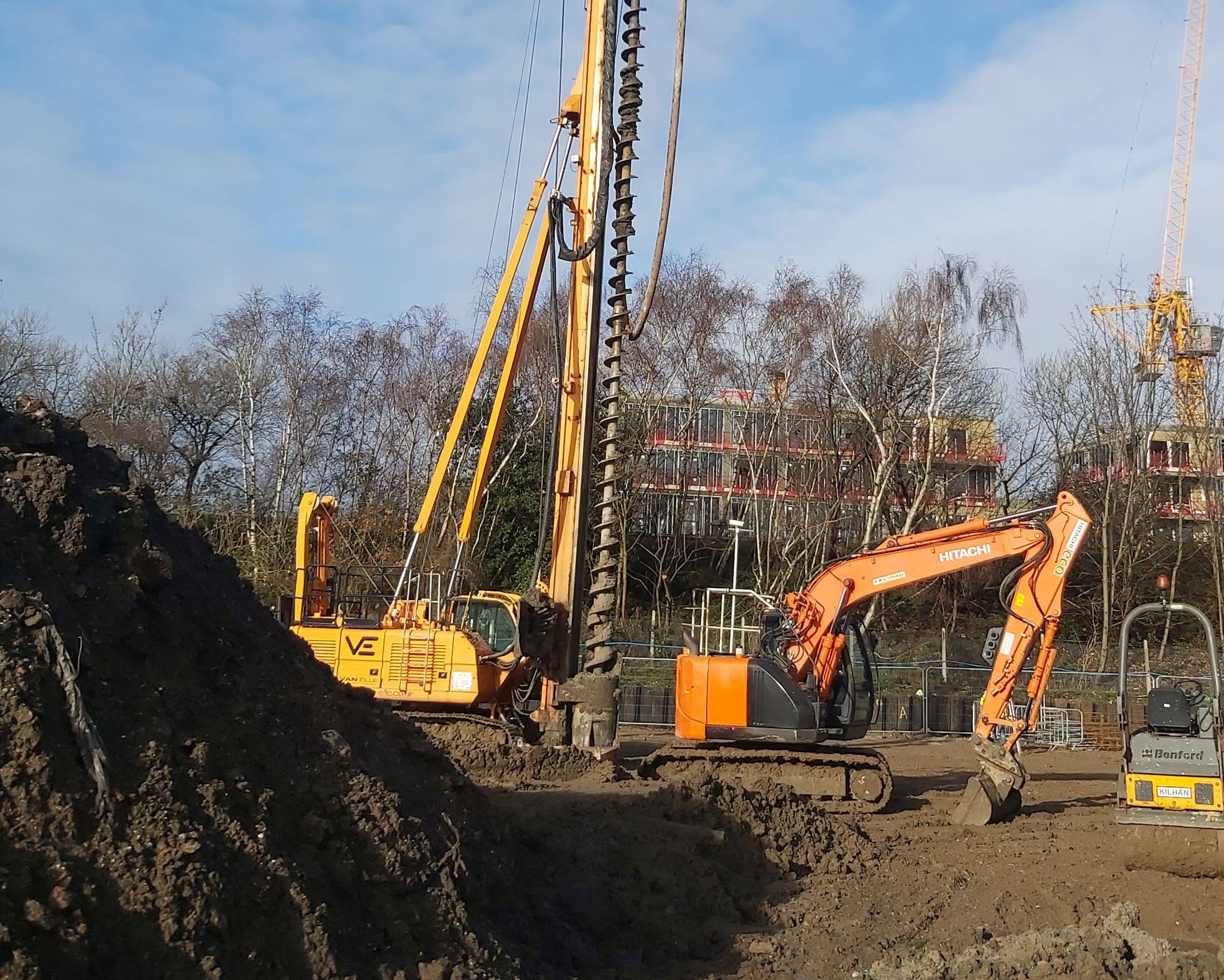
22 minute read
Assessment and Mitigation of Turbidity Risks from Piling
In recent years geo-environmental practitioners have experienced an increasing drive from regulators and water companies to assess risks to groundwater abstractions from turbidity that can be created by piling. There is currently no authoritative UK guidance on how to assess this risk.
Piling operations present a number of potential risks to environmental receptors if not correctly managed. These can include vibration and ground movement hazards, noise and creation of new pathways for contamination. Geo-environmental specialists are familiar with assessing risks from piling related to contamination, with reference to the Environment Agency’s 2001 guidance (EA, 2001), however, this does not cover turbidity. The Environment Agency has recently commissioned CL:AIRE to update the guidance and it is understood that the revised version will refer to turbidity, but that the release date is unlikely to be before the end of 2023. Planning consents for developments in sensitive areas such as the Source Protection Zone 1 (SPZ1) of a public water supply borehole often include conditions to assess and mitigate risks to the abstraction, and can specifically require turbidity to be assessed.
Why is Turbidity Assessment Required?
Abstractors of groundwater are required by the Drinking Water Inspectorate to regularly test groundwater for turbidity. The turbidity results are used as a marker for risks from pathogens such as Cryptosporidium and E. coli, which the turbidity test does not differentiate from mineral particles. Therefore, if increased turbidity is detected the operator has to shut down the abstraction until mitigation has been implemented (Burris et al, 2020). This has significant implications for supply of water to local consumers and to the cost of water treatment. Additionally, increased turbidity can compromise the disinfection process, and where the abstracted water is treated using membrane filters then the filters can become fouled by the turbidity, resulting in replacement costs running to potentially millions of pounds. Operators of a site at which piling resulted in the shutdown of an abstraction could face significant financial and reputational liabilities.
The turbidity of water presented for disinfection must be less than 1.0 nephelometric turbidity unit (NTU), and in areas where background turbidity is elevated then water companies may apply their own more stringent criteria, which can be as low as 0.2 NTU. These are lower than the UK Drinking Water Standard of 4 NTU when supplied at consumer’s taps (DWI, 2016). For context, the image below shows water with 20 to 800 NTU. The abstracted water target is clear to the naked eye and a turbidity sensor is required to detected turbidity < ~50 NTU.
The low target values that must be achieved by the abstractor, therefore, present significant challenge to the risk assessor.
A public water supply groundwater abstraction in Hertfordshire has been affected by persistent turbidity issues in the Chalk aquifer. Intrusive foundation works were undertaken concurrently on three development sites within SPZ1 of the abstraction. Specifically, continuous flight auger piling foundations into saturated Chalk occurred at two separate sites 80 m and 95 m from the abstraction. At another site less than 50 m from the abstraction, vibro ground improvement was applied to superficial deposits directly overlying saturated Chalk. Telemetred continuous turbidity monitoring was in place only for the piling foundations at 80 m the distance site and did not indicate a direct link with the turbidity issues. The other sites either had no monitoring or inadequate monitoring of turbidity, therefore, it was difficult to definitively confirm if either, or a combination, induced turbidity detected at the abstraction.
How to Assess Risks?
Review by others has not identified an authoritative methodology for quantifying risks (Burris et al, 2020), however, a qualitative approach can be employed. By development of a robust conceptual site model (CSM) similar to those used for contaminated land risk assessment, the potential risks can be qualitatively assessed. The principles of source, pathway and receptor creating a potential pollutant linkage are similar to those set out in the Environment Agency’s Land Contamination Risk Management guidance (EA, 2021). For the piling CSM the greatest emphasis is on the pathways and the source. The development of a scale cross-section is strongly recommended to both inform the assessment and to communicate it to regulators.
Where qualitative assessment identifies potential risks, semiquantitative assessment can be undertaken to better understand risks and inform mitigation measures. In higher risk scenarios the CSM could be further developed with site-specific fracture details.
The primary source of turbidity during piling is mechanical action against the aquifer producing a microscopic rock ‘flour’ in suspension in groundwater, with different piling methods likely to result in different degrees of turbidity. Loss of cement fines before cement has cured is also a concern. The turbidity created will also be a function of the strata in which the piles are installed. No studies were identified that quantify the turbidity created by piling. However, qualitative assessment can quickly identify methods that are likely to create more turbidity. Continuous Flight Auger (CFA) and other rotary methods are likely to generate turbidity, particularly when operating in rock or fine-grained strata, due to the mechanical action of the rotating parts abrading the rock or soil. For context, measurement of turbidity during drilling of 194 mm diameter boreholes in Chalk using a tri-cone rock roller reported turbidity in thousands of NTU (maximum of 4,240 NTU) while rotary cored boreholes generated up to 452 NTU (Burris et al, 2020), although it is uncertain whether either would be representative of piling turbidity. Conversely, driven piles are expected to produce less turbidity.
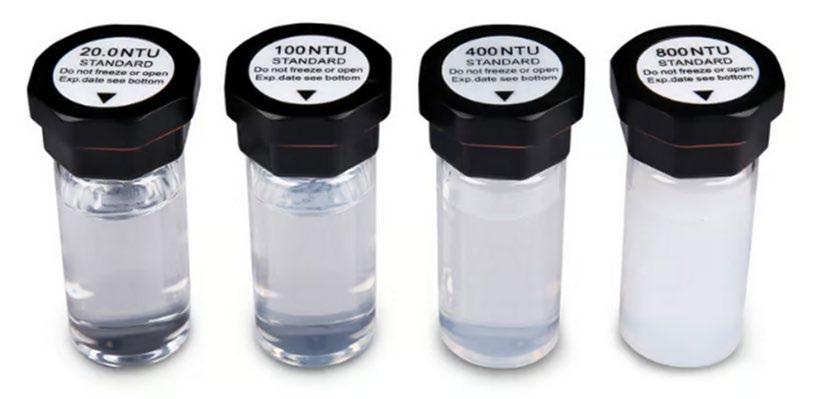
Particle size of the aquifer will be important in determining extent of turbidity migration, with finer particles migrating further in an aquifer since they can be held in suspension at lower velocities and migrate through smaller pore sizes. Particle size will be largely a function of the geological strata. In a sandstone, particles formed should mainly be sand-sized since the bonds between grains will generally be weaker than the bonds within grains. Analysis of settled turbidity produced by tunnel boring machines in Chalk reported 80% of particles to be < 10.5 µm and 20% < 0.1 µm (Burris et al, 2020), which was attributed to the size of intact coccoliths in the Chalk (approximately 10 µm) and fragmentary material, respectively.
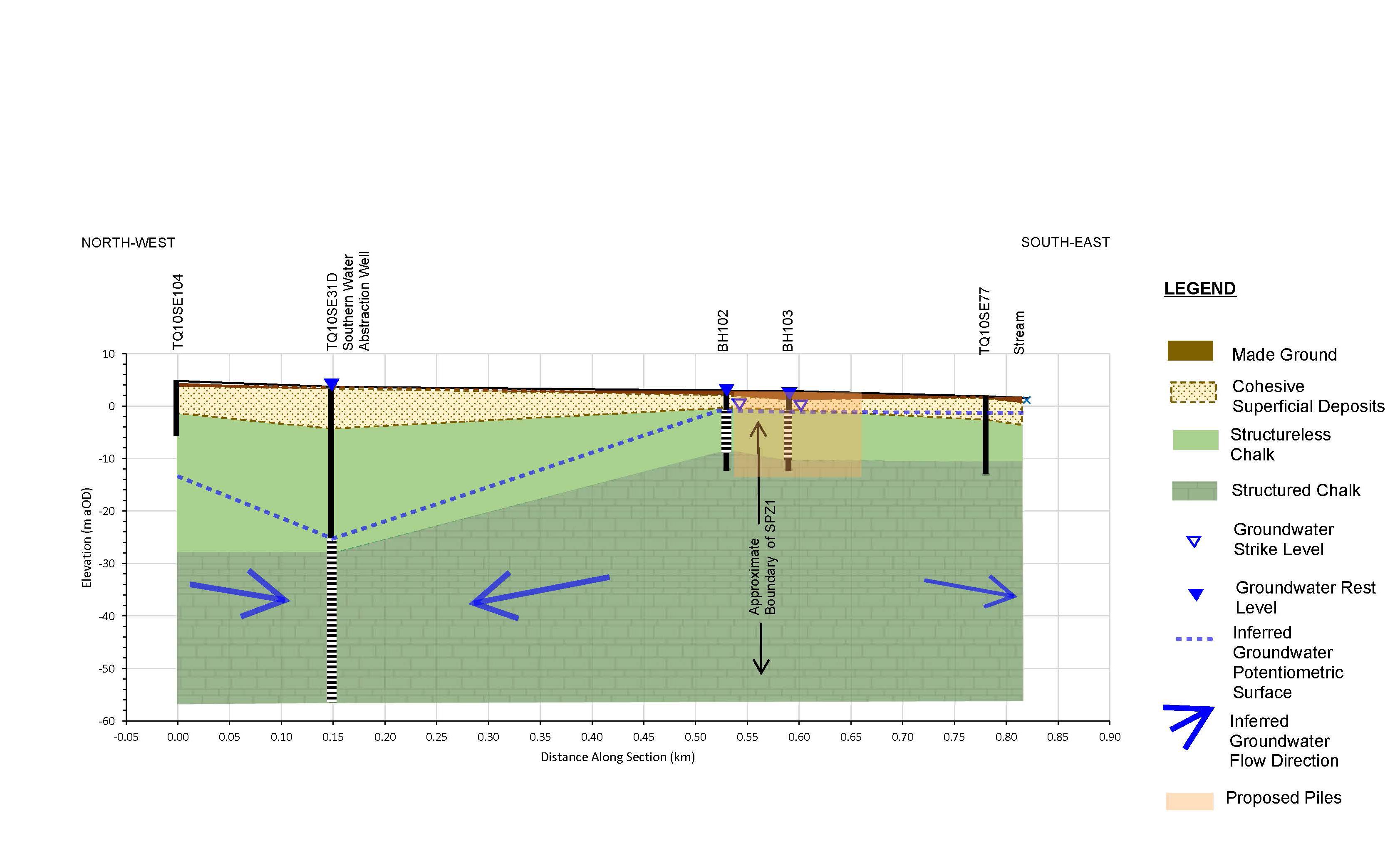
For turbidity to migrate beyond the source area then the groundwater velocity must be greater than the settlement velocity of the particles to keep particles in suspension. For intergranular flow the porewater velocity is unlikely to exceed settlement velocity, whereas in fractured rock, such as in the upper part of structured Chalk, the groundwater fracture velocity can exceed settlement velocity (Burris et al, 2020). In SPZ1 the groundwater velocity and gradient can exceed those under natural conditions, with both increasing nearer to the abstraction.
The lateral and vertical location of the source relative to the receptor will also be important in determining the risk. Piles installed in saturated strata to a similar depth as the abstraction intake will be at greater risk than piles that are much shallower than the intake, and risks increase with lateral proximity to the abstraction.
The scale of the project will affect the source magnitude, with both the number and depth of piles, and the duration of piling affecting the release rate of particles.
Other sources of turbidity include natural background of mineral particles in the aquifer, precipitation of solutes such as manganese and microbial contamination by bacteria and protozoa. The natural turbidity can also be affected by weather events such as intense rainfall and changes in groundwater level. Operation of the abstraction will also affect the turbidity of abstracted water. Stop/re-start cycles or changes in abstraction rate are major factors.
Pathways
This is likely to be the most critical part of the turbidity assessment, since in most cases it will not be possible to change the receptor, and there will be other constraints on the choice of piling method such as ground strength, cost and contamination migration. For a pathway to be present then the source zone must be connected to the receptor by strata that have pore sizes greater than the particles produced and sufficiently high groundwater velocity. The focus on velocity is a significant variation from typical solute transport CSMs.
The most likely scenario for this are karstic features or well-connected fractures in rock, with Chalk aquifers being at particular risk. It has been shown that in fractured, structured Chalk, groundwater velocity in fractures can exceed 2 km/day indicating potential for rapid transport of turbidity from site to the abstraction.
Where piles do not penetrate the abstracted strata and are separated from it by a finegrained stratum such as clay then there is unlikely to be a complete pathway, provided that the fine-grained material is intact beneath the entire piled zone and for sufficient distance down-hydraulic gradient to protect the underlying aquifer. Whilst there is no defined minimum thickness for such a stratum to prevent migration of turbidity, confidence that the stratum will be continuous and of suitable material will increase with increasing thickness. Where an assessment is reliant on such a protective stratum then it should be supported by the proven thickness on site as well as desk-study information including offsite boreholes where available and review of other references such as BGS memoirs.
Attenuation and removal of turbidity caused by suspended sediment will be mainly by settlement of particles due to low groundwater velocity. Other mechanisms are dispersion within the aquifer and dilution at the receptor.
Receptors
In most cases the receptor will be a potable groundwater abstraction, which could be operated either for public supply or by a private operator. Groundwater fed surface waters may also be considered if in close proximity to the foundation works.
Risk Assessment
Once a potential pollutant linkage has been identified, then qualitative assessment can be undertaken using the approach for land quality (CIRIA, 2001). Where risks are classified as greater than Low then further assessment or mitigation will be required. Fate and transport models for dissolved phase contamination are not suitable for assessing turbidity migration, and review by others has not identified a practicable method for modelling migration of particles in fracture flow systems (Burris et al, 2020), therefore, traditional quantitative risk assessment is not appropriate. Where quantitative methodologies have been proposed they are not known to have been recognised by regulators and the cost of collecting supporting data will be prohibitive for most sites. Semi-quantitative assessment based on dilution at the receptor may be appropriate.
A cost benefit exercise will usually be required to determine whether it is more cost effective to modify the foundation solution to reduce risks or to undertake other mitigation during piling.
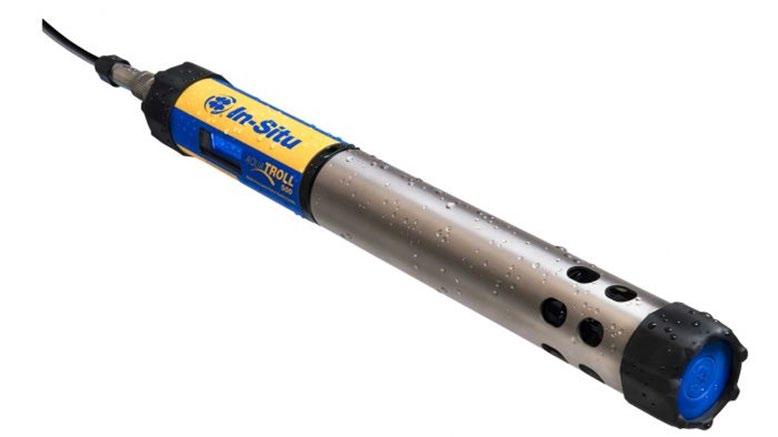
MITIGATION Foundation Design/ Re-design
Where turbidity risks cannot be addressed by risk assessment then foundation design changes may provide a lower cost, reduced timescales and more certain solution than other mitigation approaches. By altering the number, depth and diameter of piles it may be possible to terminate piles in strata overlying the aquifer and/or above the water table.
Monitoring
If the foundation solution cannot be changed to reduce risks then the most common mitigation measure is to undertake groundwater monitoring for turbidity during piling. Monitoring adjacent to the piled area allows any turbidity increase to be detected at the earliest opportunity. Monitoring can also exclude the site as a source if turbidity at the abstraction increases from another cause. Baseline and post-completion monitoring will also be required. Sentinel monitoring boreholes must be suitably located down-hydraulic gradient of the piled area, installed to similar depth as the pile bases and fitted with a filter pack representative of the aquifer material. An upgradient borehole is required to assess changes in groundwater flow direction and changes in background turbidity from natural causes such as heavy rainfall. The frequency and duration of each monitoring period will be site specific and should be agreed with the stakeholders at the earliest opportunity.
The site monitoring data should be complemented with turbidity data from the receptor borehole to show any seasonal trends or other events that affect turbidity. These data can also be used to inform the design of the monitoring programme, which will also need to consider lag-times and potential cumulative effects.
Where piles are installed in lower permeability strata then monitoring at the end of each day may be sufficient, whereas for piles installed in fractured rock with a short travel time to the receptor then real-time monitoring with telemetry and automatic alarms may be required. Real-time monitoring also offers the option to reduce piling rate to reduce turbidity.
For larger projects consideration can be given to scheduling piling to commence near a monitoring well so that worst-case data can be collected at the earliest opportunity.
Turbidity targets will be site-specific and will need to be agreed with stakeholders. The targets are often a defined increase relative to baseline conditions. When setting targets it is important to recognise the detection limits of the proposed monitoring instruments to ensure that the target can be detected.
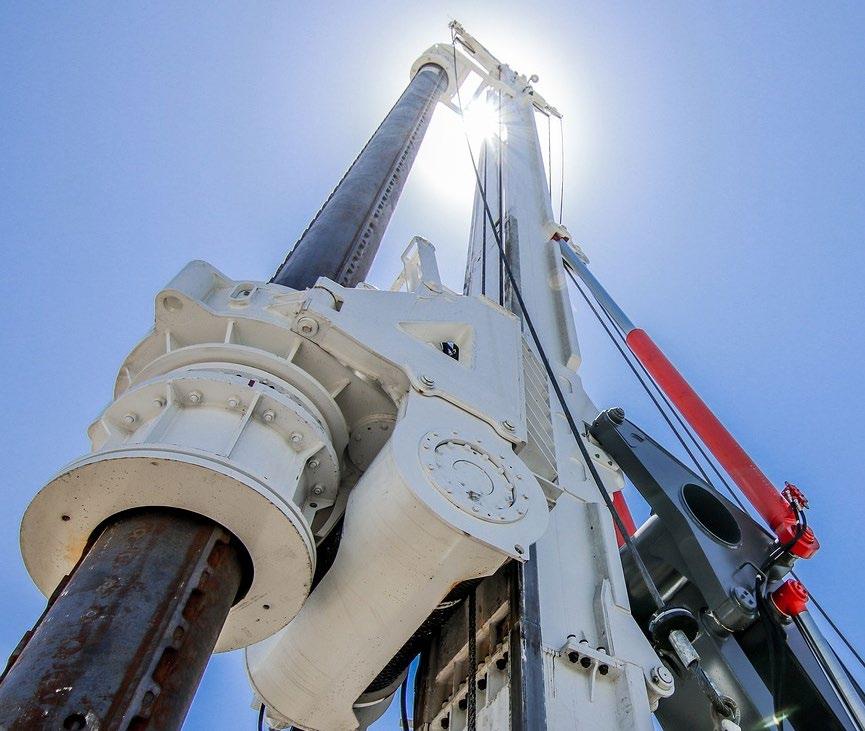
Other Mitigation
Alternatives to monitoring that have been implemented including funding or indemnification for the abstractor to undertake additional treatment of abstracted water before disinfection, or abstracting turbid groundwater adjacent to the source and treating it before discharge to ground. However, these are likely to be prohibitively costly and time consuming to agree with other stakeholders and implement, even if agreement can be reached.
Conclusions
Assessment of turbidity risks from piling can be undertaken by qualitative assessment of source-pathway-receptor linkages based on a robust understanding of ground conditions. In many cases this will be sufficient to demonstrate that risk is acceptable without further works. Where the qualitative assessment identifies potentially unacceptable risks then the risk can be controlled by implementation of mitigation measures.
The authors thank Philip Burris for providing technical review.
References
Burris et al, 2020. Tunnelling, Chalk and turbidity: conceptual model of risk to groundwater public water supplies. P. Burris, C. D. Speed, A. E. Saich, S. Hughes, S. Cole and M. Banks. Quarterly Journal of Engineering Geology and Hydrogeology
CIRIA, 2001. CIRIA Report C552 ‘Contaminated Land Risk Assessment: A Guide to Good Practice’. CIRIA 2001.
DWI, 2016. The Water Supply (Water Quality) Regulations 2016, Schedule 1. The Drinking Water Inspectorate. 2016.
EA, 2001. Piling and Penetrative Ground Improvement Methods on Land Affected by contamination: Guidance on Pollution Prevention. Report NC/99/73. Environment Agency, May 2001.
EA, 2021. Land contamination risk management. Published online 8th October 2020, updated 19th April 2021.
UK WIR, 2012. Turbidity in Groundwater Understanding Cause, Effect and Mitigation Measures. Report 12/DW/1/4/5. UK Water Industry Research. 2012.
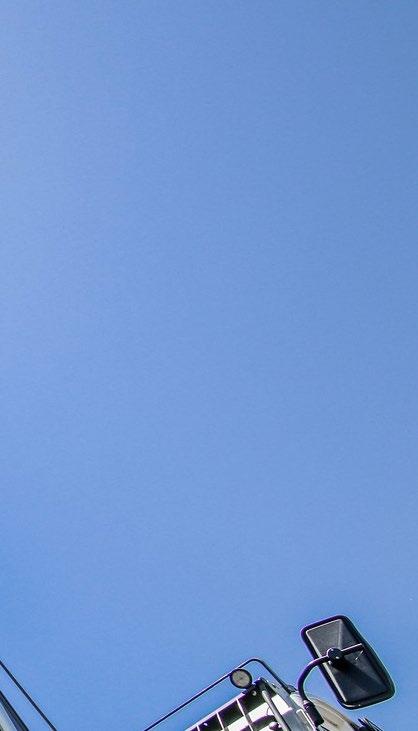
Article contributed by Markus Hjort1, Eleni Vaiopoulou1, Richard Gill1,3, Pablo Campo4, Célia Lourenço4, Chris Walton4 , Tamazon Cowley4, and Frederic Coulon4
1 Concawe (Scientific Division of European Fuels Manufacturers Association), Brussels, Belgium
2 ExxonMobil (Esso Petroleum Company Limited), Avonmouth Fuels Terminal, St. Andrews Road, Avonmouth, Bristol, BS11 9BN, United Kingdom
3 Shell Global Solutions International B.V., Carel van Bylandtlaan 30, 2596 HR, The Hague, Netherlands
4 Cranfield University, School of Water, Energy and Environment, Cranfield, MK43
0AL, UK
Petroleum hydrocarbons are common soil contaminants that pose a risk to human and environmental health.
Different analytical methods are used to determine their presence in soil, including non-specific screening techniques and labbased fingerprint techniques. While the latter provides high accuracy, they can be timeconsuming and expensive.
Over the past decade, the emergence of various field analytical techniques, such as test kits and portable handheld devices, have enabled real-time petroleum hydrocarbons detection and measurement on-site, which has the potential to drastically reduce cost and time of analysis compared with traditional technologies and without sacrificing ‘Quality Management’ objectives. However, their performance for different soil types, contamination levels, and fuel types, as well as their ability to speciate and quantify different hydrocarbon groups for risk assessment, and their suitability for remediation monitoring and validation, have not been fully studied. It is also important to understand the type and quality of data that will be generated by field analytical technologies and interpretation of the data generated should be carefully evaluated before conclusions are drawn. Depending on which analytical technology is used, it is possible to achieve qualitative, semi-quantitative and quantitative results. In some cases, the accuracy of field analytical technology is approaching that achievable previously only from laboratory analysis. Yet, laboratory analysis may still be required to attain legally recognised measurements.
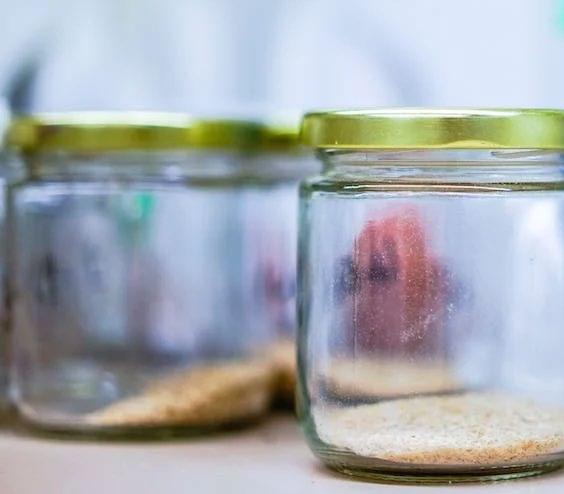
The main goal of this study was, therefore, to assess and raise awareness about the feasibility of using field-based techniques for determining TPH concentrations in soil, and whether they can replace lab processing. The study reviewed various techniques, ranging
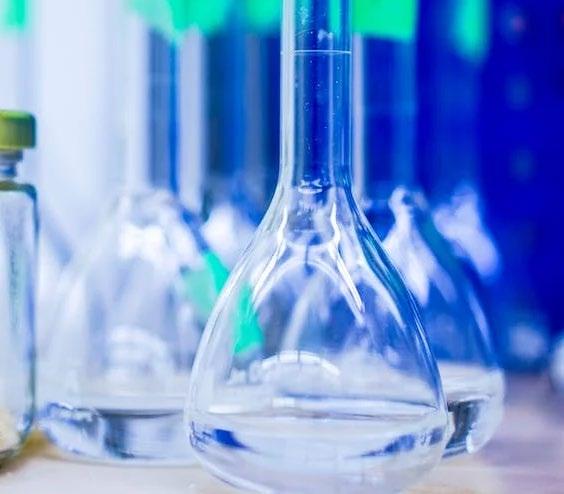
Legend key Table 1: Qual: Qualitative; Semi-Quant: Semi Quantitative Quant: Quantitative; VOC: Volatiles Organic Compounds - include a range of seleted low molecular weight aliphatic and aromatic hydrocarbons up to EC12; BTEX: benzene, toluene, ethylbenzene and the three xylene isomers: a variant on VOC analysis which specifically targets BTEX; SVOC: Semi-volatiles Organic Compounds including aliphatic and aromatic hydrocarbons in the range of C12-C40; PAH: Polycyclic aromatic hydrocarbons: a variant of SVOC analysis targeted to the aromatic hydrocarbons group. This is usually the 16 PAHs listed in the US Environmental Protection Agency.; N.A. Not applicable.
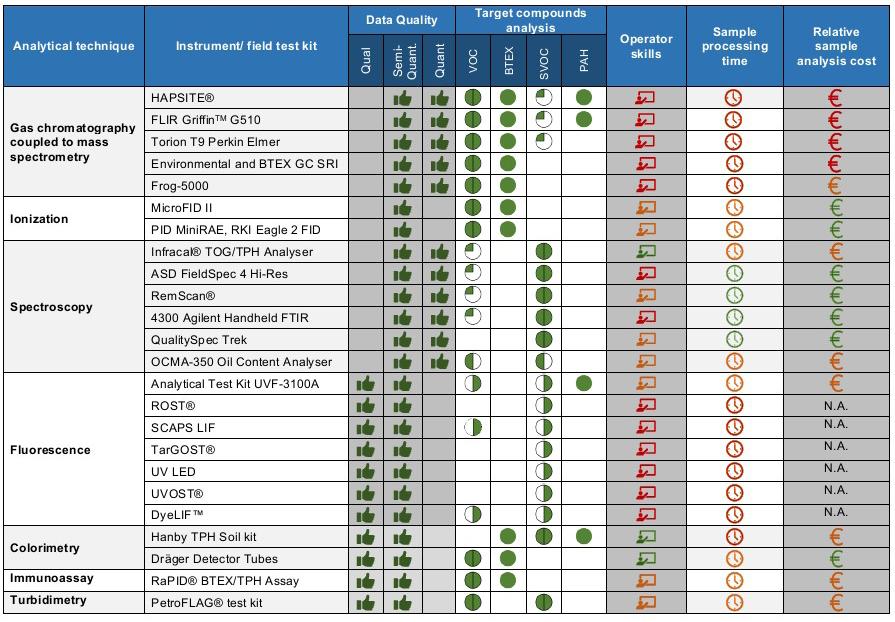
Target analytes: Both aliphatic and aromatic hydrocarbons of the group; Greater response towards aliphatic hydrocarbons. Greater response towards aromatic hydrocarbons. Covering only selected compounds ranges within the hydrocarbons group (e.g. spectroscopy only VOC between EC10-EC12; gas chromatography covering selected SVOC up to EC21)
Operator skill: Low ; Moderate High;
Sampling processing time: immediate (few sec); intermediate (within few minutes); long (several minutes);
Relative cost: relatively low; relatively moderate; relatively high from high-end gas chromatographs and handheld infrared spectrometers to low-end oil pans and chemical kits. To ease comparison, the field analytical kits and devices were classified according to detection methods, target analytes detected and data quality levels (qualitative, semiquantitative and quantitative) (Table 1, left). The basic principle along with the advantages and limitations of each field analytical technique, quality control requirements, operator skill level, and analysis cost are summarised and the synthesis can be accessed for free on the Concawe website at https://www.concawe.eu/wp-content/ uploads/Rpt_21-3.pdf.
In general, the field analytical technologies for detecting petroleum hydrocarbons in soil are highly developed and well established. In terms of risk assessment, only GC-MS can accurately differentiate between aliphatic and aromatic petroleum fractions. However, this technique involves soil sample extraction and demands a high level of expertise, which may not be suitable for all projects. On the other hand, colorimetric, immunoassay, and turbidimetry test kits are cost-effective and rapid options for monitoring the reduction of petroleum hydrocarbons over time and guiding remediation strategies, but they lack specificity and do not provide information on individual analytes. Field spectrometry technologies offer real-time measurement of petroleum hydrocarbons in soil with minimal sample handling but require soil drying and cannot discriminate between aliphatic and aromatic fractions. Fluorescence technologies are used for in-situ site investigation with high spatial resolution but provide relative data and require skilled personnel. Both spectrometry and fluorescence systems can be useful in adaptive sampling designs to detect and predict contamination levels during the Phase 2 Investigation.
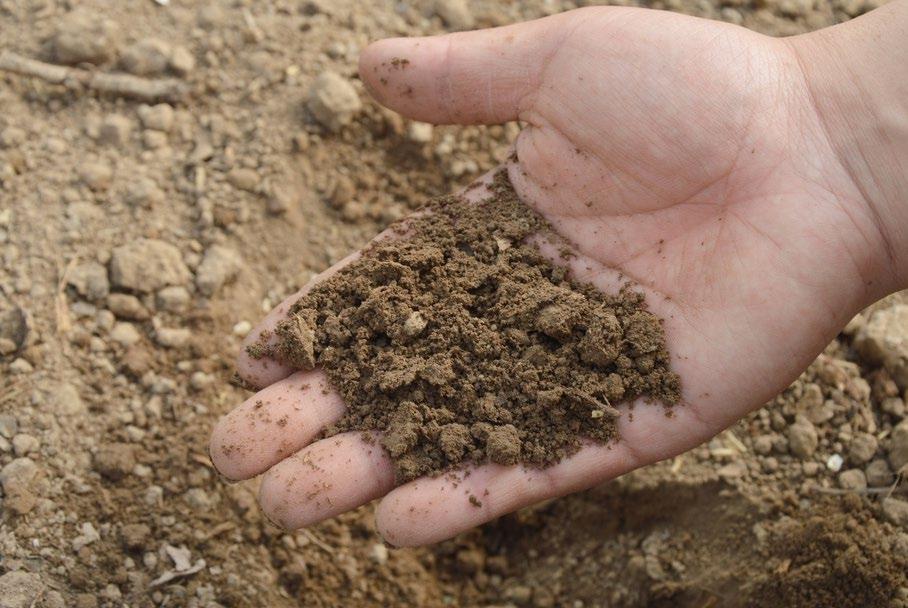
It is important to note that no single field analytical technology can quantify the entire range of petroleum hydrocarbons in soil, and therefore a combination of technologies may be necessary for greater accuracy in prediction.
A representative subset of seven field-based technologies was then selected and tested with impacted soil samples, which were compared to results from accredited laboratory analysis
[Table 2]. The subset included 3 portable solvent-based technologies (one portable GCMS (FT1), one portable nondispersive infrared (NDIR) spectrophotometer (FT2), one portable ultraviolet fluorescence (UVF) spectrometer (FT3)), and 4 handheld solvent free technologies (one handheld visible and nearinfrared reflectance (vis-NIR) spectrometer (FT4), two handheld Fourier-transform infrared (FTIR) spectrometers (FT5 and 6), and one handheld photoionization detector (PID; FT7)). Three soils (sandy loam, silty clay loam, and clay loam) were spiked with gasoline or diesel fuel on weight/weight basis to achieve 100, 1000 and 10,000 mg/kg spike levels.
All samples were analysed for Total Petroleum Hydrocarbons (TPH), Volatile Organic Compounds (VOCs), Gasoline Range organic (GRO) and Diesel Range organic (DRO) and speciated hydrocarbon compounds when the chosen technology allowed to do so. Gasoline spiked soils were not analysed with FTIR and vis-NIR spectroscopy as the use of methanol as preservative interferes with the analysis.
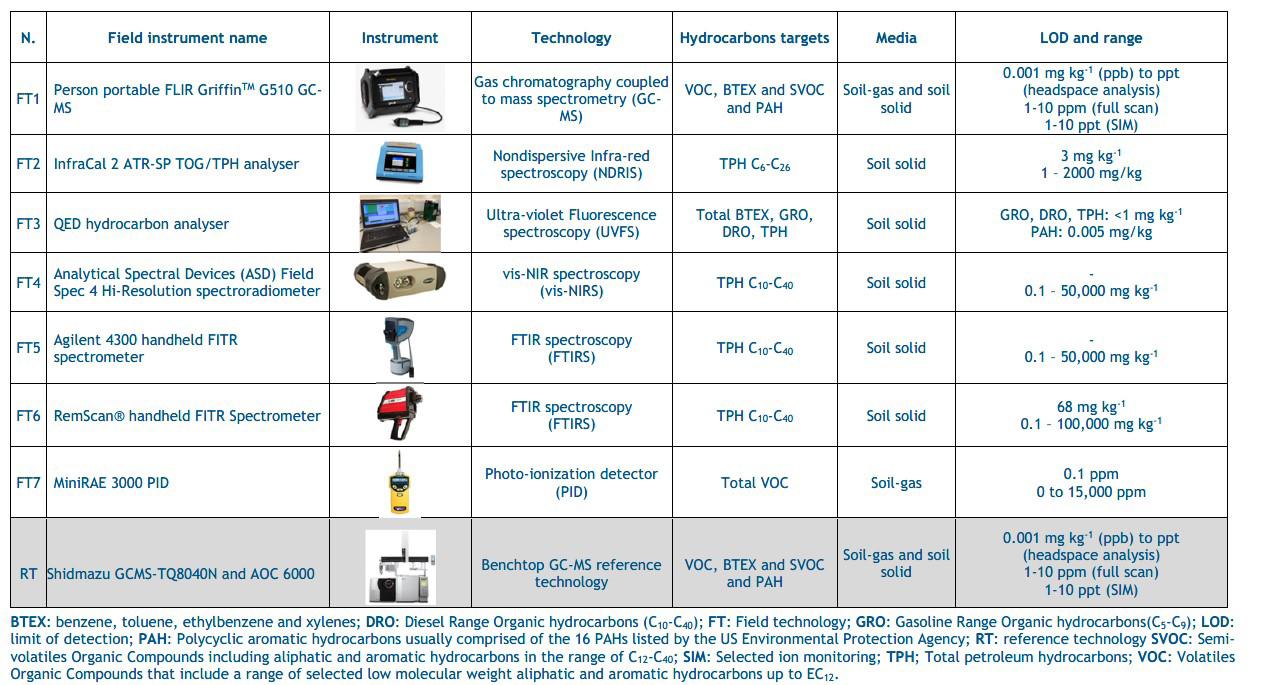
It has also been reported that non-preserved samples contaminated with gasoline are subject to volatilisation losses that occur during the analytical process, which result in poor performance for such technique. The intra and inter spikes consistency were evaluated by determining (1) precision from triplicates expressed as the percentage of relative standard deviation (%RSD) and (2) bias, which is the difference expressed as a percentage between the mean of the replicate measurements and the spiked theoretical concentration level. Similarly, performance comparison of the field technologies against a benchtop GC-MS technology was carried out by determining the difference (%) between the mean measurements determined by the benchtop GC-MS and the field technologies evaluated. Additionally, performance characteristics of the GC-MS were determined by analysing the certified reference material RTC-SQC026 in triplicate.
All solvent-based field technologies performed well for TPH determination in different soil types, while solvent-free non-invasive technologies showed higher variability and lower accuracy. Infrared technologies are influenced by soil characteristics, particularly for low-level spikes (<1000 mg/kg) and certain soil types. The portable GC-MS performed well and closely to the benchtop GC-MS. While the headspace analysis of the portable GC-MS was easy to use and allowed to save time compared to the benchtop GC-MS, extra analysis time was required for the soil extraction and analysis due to manual injection. The non-destructive and solvent free FourierTransform IR (FTIR) and visible and nearinfrared reflectance IR (vis-NIR) spectroscopic technologies performed well with diesel and were demonstrated to be versatile, fast, and easy to use methods, but the accuracy was lower than for other technologies when total petroleum hydrocarbons (TPH) levels were <1000 mg kg-1. The procedures for soil calibration and validation may further limit the FTIR and vis-NIR applicability for diverse soil type and fuel type. In comparison, the nondispersive IR (NDIR) and UVF spectroscopy technologies showed better performance, typically ±15% precision and ±30% bias for quantifying TPH in soil, which meet regulatory requirements. The UVF technology also provided additional quantitative information into hydrocarbons groups which can inform swiftly remediation monitoring and validation. Analysis of soil-gas samples by photoionization detector (PID) showed that PID underestimated concentrations compared to both portable and benchtop GC-MS which was expected as PID only provides an indirect and approximate indication of concentration of volatile compounds (VOC) in soil. Nevertheless, the PID remains a valuable instrument for site risk screening of soil-gas vapours considering its low cost and ease to use. Complete report is freely available on Concawe website at https:// www.concawe.eu/wp-content/uploads/ Rpt.22-12.pdf
The authors are grateful to all members of the Concawe Soil and Groundwater Taskforce (STF-33) which include a wide team of collaborators and advisors across Europe for their useful discussions and contribution during the study progress and revision of the reports.
Findings of SiLC DoWCoP industry survey: Regulatory challenges for regeneration of historical landfills and reuse of stockpiles and mineral waste
Article contributed by Clive Williams (Senior Associate Contaminated Land, Mott MacDonald), Louise Beale (Technical Director, SLR Consulting), Sarah Owen (Senior Associate, Waterman), Sarah Bullock (Associate Director, Atkins) and Catherine Copping (Technical Director, Stantec)
Given on-going interest and concerns about regulatory constraints to the regeneration of former landfill sites, Specialist in Land Condition (SiLC) has been examining recent regulatory approaches and use of the CL:AIRE Definition of Waste Code of Practice (DoWCoP) in England and Wales.
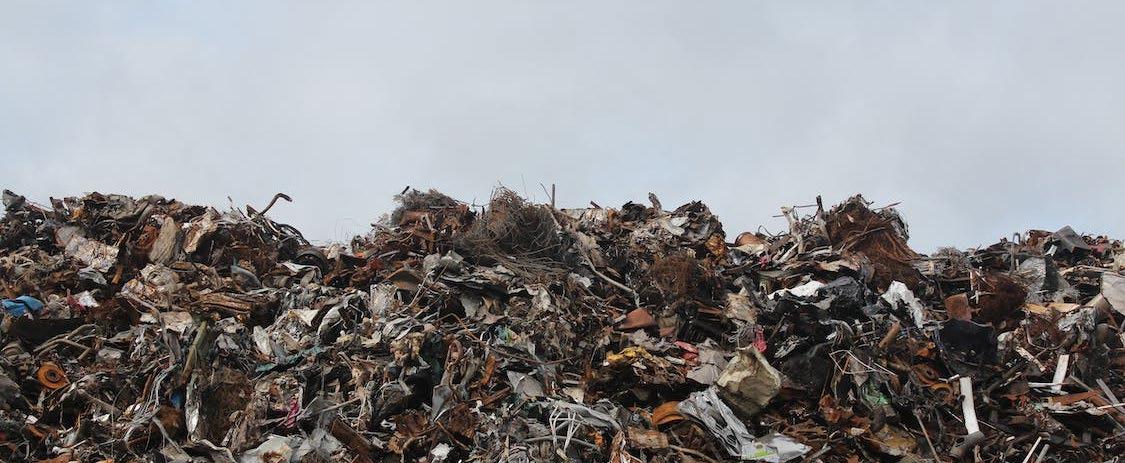
SiLC held a webinar in June 2022 entitled ‘Regeneration of historical landfill sites –views from multistakeholder perspectives’. This included looking at the history and development of the DoWCoP and the key decisions that underpinned this; presentation of case studies which illustrated how DoWCoP could effectively be used for regeneration of historical landfills while securing environmental betterment and protection of health; and case studies that clearly showed significant concerns with undertaking land regeneration under the permitting regime. Alongside this webinar, SiLC undertook an online survey in August and September 2022 requesting views on the use (and misuse) of the DoWCoP and experiences of the difference between project and programme delivery via DoWCoP and waste recovery permitting. There was a large response to this with some 212 responses received from the consulting, contracting and regulatory brownfield development community. The key take-away messages included a strong desire to maintain the DoWCoP as a robust and effective tool to manage brownfield development (including historical landfills) but also highlighted some issues. For example, the survey responses referred to inappropriate use of the DoWCoP for dealing with mineral wastes, such as colliery spoil.
The DoWCoP was published in 2008 and is currently in its 2nd edition, with a 3rd
Historic landfills
Old stockpiles
Mineral waste or colliery spoil 39% (82 respondents) 65% (137 respondents) 24% (50 respondents) edition keenly awaited for some time. On 12th July 2021 the Environment Agency (EA) confirmed to SiLC that “Our DoW CoP and Land Contamination leads are in the final stages of drafting internal guidance around these matters as we are aware of the industry's interest. The guidance will make it clear to our staff the appropriate permitting options for these activities and ensure we maintain consistency in our opinion. We will share this with you once it has been issued and welcome any further comments.” SiLC wrote to DEFRA and the EA on 21st April 2023 again requesting a review of EA guidance Downloads | Specialist in Land Condition Register Ltd (SiLC)
The current scope of the DoWCoP covers the reuse of soil, rock, dredged material, made ground and aggregates derived from construction and demolition waste as well as stockpiles containing these materials on the site of origin. The 2nd edition expanded the scope of the DoWCoP to include transferring natural soils and rock directly from a donor site to a development. It is however important to note that the DoWCoP specifically excludes application to soil contaminated with invasive weeds, infrastructure (pipes, tanks), construction wastes such as plasterboard, glass and wood, and mining wastes. Whilst not specifically excluding historical landfills from the DoWCoP, it is becoming increasingly the case that the Environment Agency will not support the use of the DoWCoP for any development work on such a site regardless of its licensing/ permitting history and date of deposition.
The figures in Table 1 demonstrate a lack of understanding and confusion within the industry of what the DoWCoP covers and points towards confusion within the regulatory bodies who must confirm no objections (or no response within 21 days) to the reuse of materials in advance of a declaration being made. One Local Authority respondent reported “we often have to respond to EA/QP issues (i.e. sites being subject to DoWCoP when planning conditions for site investigation have not been satisfied let alone agreed a Remediation Method Statement”.
Over the last three years half the respondents reported being told by the EA they could no longer use DoWCoP for projects they would previously have done so (Graph 1, overleaf) with the main reason being cited as development of historical landfills. One respondent indicated that personal discussions with EA Area Officers has clearly shown that Area Officers are also frustrated. They can often see environmental benefits and gains from a proposal that they are told is no longer in line with EA national thinking on the use of DoWCoP. One QP responded “It is not always going to be acceptable to reuse material from a historical landfill, or a stockpile, these are site specific decisions and often, will not be straight forward. Rather than a blanket, include or don't include in DoWCoP, each case should be considered in terms of its Conceptual
Site Model.”
While the position for historical landfills is understood not to have yet been formalised the following footer is now appearing on some emails from the EA permitting team “The Environment Agency considers that any material which consists of current or historically landfilled waste remains waste on excavation and therefore cannot be used under DoWCoP. Excavated landfilled waste materials require appropriate waste controls such as an environmental permit for storage, treatment and deposit.” Whilst this position is now being circulated, there is limited steer or guidance on specifics such as what constitutes a current or historically landfilled waste, which could again be subject to interpretation. In addition, consultants have recently reported changes in position within the EA on how sites are to be regulated mid-way through projects.
30% of respondents had obtained an environmental permit to permanently deposit waste on land as a recovery activity (also referred to as deposit for recovery or DfR for short) in the previous 18 months. The time required to obtain the permit varied with 8% (five) of permits issued in six months or less, average was between 18 months to 2 years, with some reported as five years. Two refusals were reported after 3 years of consultations.
Major benefits of using the DoWCoP compared to a DfR permit are considered to be the reduced time and costs taken to prepare the Material Management Plan and submit the declaration. Where DoWCoP has not been used to support development our survey respondents reported significant cost increases, project delays and worryingly a trend towards the requirement to landfill material offsite and importing virgin aggregates to make up levels (Graph 2). Almost 1 in 5 projects were abandoned because the increased costs and delays to programme. 80% of respondents consider the exclusion of landfills, mining wastes and stockpiles from DoWCoP would hinder brownfield development. Some commonly expressed views were that their exclusion seems arbitrary and would hinder sustainable materials management for no economic, social or environmental benefit. Respondents also acknowledged that there needs to be effective regulatory oversight, particularly with landfilled wastes. Excluding landfills is considered counterproductive to the drive for sustainability and adoption of circular economy principles, leading to superfluous use of virgin materials, which result in the additional use of earth’s resources, increased carbon expenditure from additional transport of materials, impact on the utilisation of key landfill space. Regeneration of landfill sites can have considerable positive impact on the social value of an area and short term potential negative impacts during redevelopment need to be managed.
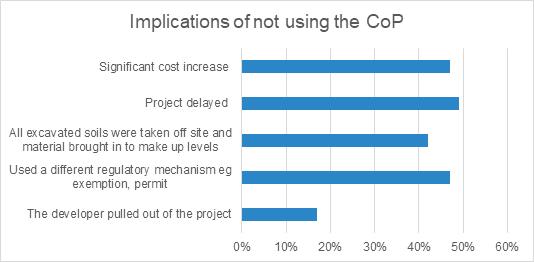
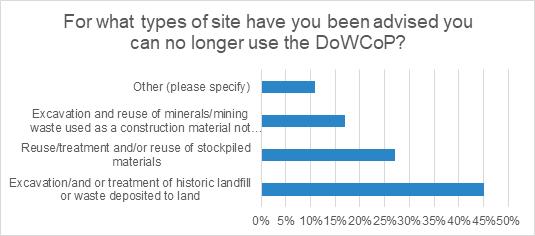
There is a significant view from respondents that the DoWCoP should be extended to cover development of landfills and materials from former mining activities including old stockpiles (Graph 3). The existing permitting regime has few advocates within the industry with some calls for a simpler (quicker) standard rules waste recovery permit.
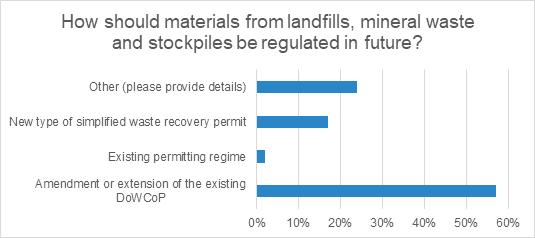
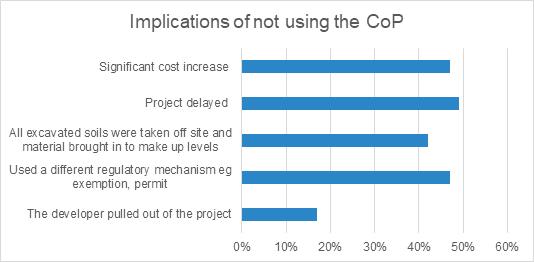
There were also calls to modify the definition of waste to exclude materials for which there is a defined use. The review of legislation since Brexit offers an opportunity to rethink how waste is classified and material is reused within the UK in accordance with circular economy, sustainable development and net zero principles.
Conclusions
There is an increasing trend from the EA to require an environmental permit (deposit for recovery) to manage the reuse of material on development sites (historic, current landfills, stockpiles, possible mining extractive waste etc). The EA does not have capacity within the permitting system to accommodate this increase. Permit decision times of greater than 6 months will not work in the construction sector. An increasing number of permits need to be bespoke because material for reuse and/ or the environmental setting does not fit the standard rule permits available.
The DoWCoP has proved its worth to the brownfield development industry since its publication in 2008. It offers a simple costeffective and crucially timely approach to managing the reuse of materials in construction. It also provides a pragmatic approach to the sustainable reuse of materials supporting the drive for circular economy, zero waste and enhancing social value all of which are key concerns in relation to brownfield regeneration. The constraint to regeneration posed by the need to use the permitting regime is at odds with government drivers including brownfield first, recovery from Covid, levelling up and build back better. However, use of the DoWCoP has been inappropriate at times and as an industry we need to tighten up our understanding and application of it to regeneration.