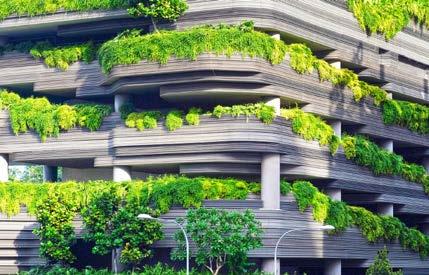
5 minute read
CARBON CONSTRUCTION The energy embodied within materials technology and products is a key factor in a building’s carbon footprint
CARBON CONSTRUCTION
A significant amount of energy is trapped within the materials used in the creation of our buildings. The energy embodied within materials, products and technologies is a key factor in the carbon footprint of buildings due to their direct link to sourcing of raw materials, manufacture procurement and construction.
Advertisement
WHAT IS EMBODIED CARBON?
Embodied carbon considers all the greenhouse gasses associated with the entire lifecycle of a material or product. It includes extraction, manufacture, transportation, installation, maintenance and endof-life carbon associated with each building material and/or component over the lifecycle of the building.
WHY DOES IT MATTER?
Historically, reducing the building sector’s carbon emissions has always targeted operational carbon. This has been appropriate in South Africa where our grid is so carbon intensive. But when grids become cleaner (as they have largely done in Europe), the focus of reducing emissions in the built sector moves to embodied carbon.
While operational carbon is produced during the day-to-day activities of running and using a building, embodied carbon results from producing, procuring and installing materials and components that make up a structure, as well as encompassing the end-of-life stages of a building (ie demolition, removal and repurposing of materials). For the industry to make headway with understanding the overall carbon impact of the built sector, whole life carbon must be assessed.
Due to the lifespan of buildings (typically 60 years), materials that are being specified into new buildings now need to shift towards lower embodied carbon materials to support the required shift towards a low carbon built sector.
HOW IS IT CALCUATED?
Embodied carbon is measured using a lifecycle analysis and is categorised into carbon associated with the various stages of a building.
Above: Embodied carbon (yellow) and operational carbon (blue) across the key lifecycle stages of a building. Below: Terminology used in this report cross-referenced to terms and lifecycle stages defined in EN15978.
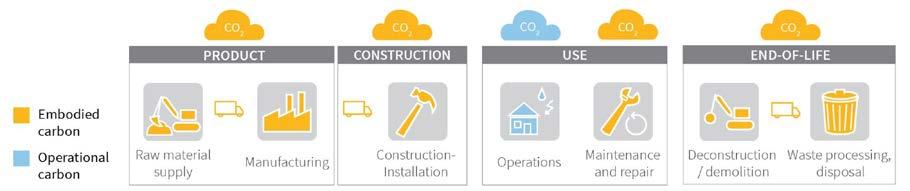
CLF-Policy-Primer-1
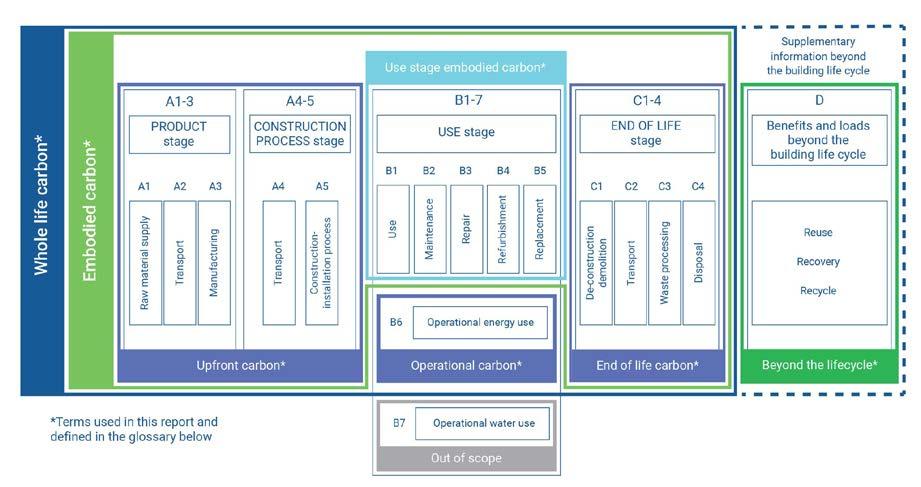
WGBC WGBC Bringing embodied carbon upfront
Upfront embodied carbon: this relates to embodied carbon from material selection to practical completion and includes A1-A3 as well as A4 and A5.
A1-A3: Manufacture, transport and installation of construction materials that relate to the extraction of raw materials and the manufacturing of materials to factory gate.
A1: Raw material extraction and processing, processing of secondary material input (eg recycling processes)
A2: Transport to the manufacturer
A3: Manufacturing to factory gate
Module A1, A2 and A3 may be declared as one aggregated module A1-A3. All stages include the provision of all materials, products and energy, as well as waste processing up to the end-of-waste state or disposal of final residues during the product stage. The assessment takes only the building and its parts into account, but typically not furniture or appliances.
A4: Transport to the building site
A5: Installation into the building
Stages A4 and A5 include all impacts and aspects related to any losses during this construction process stage (ie production, transport and waste processing and disposal of the lost products and materials). Stages A4 and A5 would typically be applied to reused materials. Embodied carbon over the lifecycle: embodied carbon associated with the selection and replacement of materials during the lifespan of the building and relates to B1-B5. End-of-life embodied carbon: embodied carbon associated with disassembly, transportation away from site and waste process of materials and relates to C1-C4. Whole life carbon: includes all the above stages of embodied carbon and addresses all stages of a structure’s life (cradle to grave). Beyond life embodied carbon: embodied carbon associated with the reuse, recycling and recovery of materials and relates to D, this is an additional section that can be included in whole life carbon assessment.
WHAT DO I NEED TO KNOW?
• Build and contribute to local data. Local data on carbon in materials is limited, but it is important in driving good life carbon assessments • Demand information from suppliers. One of the factors limiting the implementation of sustainability is the difficulty that professionals find in locating products with bona fide preferential environmental and health features. • Look for and specify low carbon materials. Get to know what is out there regarding low carbon materials, products and systems available. This information is accessible locally through several eco-standard product certifications. • Don’t forget about traditional materials.
Materials such as rammed earth, thatch and timber have a lower embodied carbon and although not always commercially viable can be used and adapted in a beautifully modern way. • Design for flexibility. In this context, it means designing for a variety of space uses in the hope that the building does not need to be refurbished too often. • Design for disassembly (DfD) and reuse. All sectors of the built environment need to design,
manufacturer and incorporate materials, products and technology in ways that facilitate DfD, or at the very least do not hinder it. Only in this way can we be assured that once we have created a resource, that it is not lost and can enter the cradle-to-cradle recycling loops and not need to be replaced with virgin resources at end of first or subsequent use. • Design for reuse. If it’s not reusable or recyclable to a high value, it’s not sustainable. This design approach drives design re-thinking throughout the project, requiring the design team to think “up” from the construction of building elements to the whole building. (Walker Morison, 2007) • Buy recycled. An important initiative to ensure that the carbon footprint of materials is minimised is to ensure that products being purchased and specified already contain recycled content. • Material minimisation. Advancements in technology such as 3D printing allow the development of materials that take advantage of their structural integrity while eliminating the mass of materials required. • Renewable inputs. The use of renewably sourced products has a major impact on reducing overall environmental and carbon impacts. Renewable materials can still be sourced in ecologically unsustainable ways, so care must be exercised that resources are sourced sustainably.
COMPLETING THE SOLUTION
The initiatives discussed above have a critically important role in promoting sustainability up to a point. If we reduce our demands on nature’s systems and living resources, there is no doubt that regeneration will occur to some extent.
The key question is: can nature replenish herself in the context of explosive consumption, ongoing population growth and the inexorable growth of cities where impacts are concentrated and populations insulated from their impacts?
If we are to solve the looming global warming crisis, we need all the tools in our armoury to be fully primed and must be ready to actively implement.
FURTHER READING
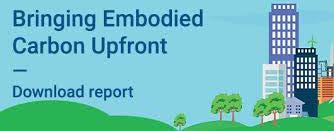
Above: The WorldGBC’s report on embodied carbon. Below: GBCSA’s guide to developing buildings in South Africa.
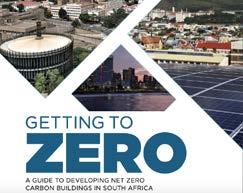
DOWNLOAD GUIDES BY CLICKING ON PICTURES