
15 minute read
Fumonisins impact in aquaculture: A real threat?
Rui A. Gonçalves, Lucta
When talking about mycotoxins' impact on aquaculture, the most typical response is “it depends”, which is always a “correct answer”, however, “most of the time” is also an unsatisfactory answer.
It is easy to understand that with the high number of produced species (466 species-groups, excluding algae and aquatic plants) in aquaculture, it would be extremely hard to fully understand mycotoxins’ impact in such a highly diverse industry. However, we need to be aware that the extrapolation and/or generalization of the already learned concepts for some species may be incorrect and dangerous.
Fumonisins: What are they?
Fumonisins are naturally occurring toxins produced by a number of Fusarium fungi species, notably F. verticillioides, F. proliferatum and F. nygamai. Several different types of fumonisins are known, but fumonisins B1, B2 and B3 (also named FB1, FB2 and FB3) are the major forms found in feed and food. You will note that most of the experiments performed in animal nutrition tend to use FB1 (purified form) or a combination of all three forms (especially when using natural contaminated ingredients).
Fumonisins are characterized by having a long-chain hydrocarbon unit (similar to that of sphingosine and sphinganine), which plays a role in their toxicity. Fumonisins inhibit the sphinganine (sphingosine) N-acyltransferase (ceramide synthase), a key enzyme in the lipid metabolism, resulting in a disruption of this pathway. This enzyme catalyzes the acylation
Figure 1. Fumonisins sensitive levels for freshwater species reported in the scientific literature.
and environmental-related (farm management, biosecurity, hygiene, temperature) will highly influence the sensitive levels. Therefore, it is not a surprise that sensitive levels may vary within the same species. Figure 1 shows the minimal sensitivity level for fumonisins reported in the scientific literature for African catfish (Clarias gariepinus), channel catfish (Ictalurus punctatus), African clariid catfish (Heterobranchus longifilis), common carp (Cyprinus carpio), Nile tilapia (Oreochromis niloticus) and rainbow trout (Oncorhynchus Figure 2. Fumonisins sensitive levels for marine species towards fumonisins reported in the scientific mykiss). It is important to literature. note that the EU guidance value for fumonisins (FB1 + of sphinganine in the biosynthesis of sphingolipids FB2) in complementary and complete feedstuffs and also the deacylation of dietary sphingosine and for fish is currently 10,000 µg kg-1 (EC, 2006). the sphingosine that is released by the degradation As shown in Figure 1, only two species (in this figure) of complex sphingolipids (ceramide, sphingomyelin have reported sensitive levels below 10 ppm (i.e., and glycosphingolipid). Therefore, sphingolipids are 10,000 µg kg-1) and two reports indicate negative key components for the membrane and lipoprotein effects over common carp at the 10 ppm. structure and will also play an essential role in cell Regarding the African catfish (Clarias gariepinus), regulations and communications (second messenger Gbore et al. (2010), reported that the exposure to for growth factors). contaminated diets containing 5,000 µg kg-1 or more of FB1, for six weeks, induced a negative What do we know about FUM in aquaculture? physiological response in fingerlings (17.35 ± 1.26 g). In reality, very little is known about the potential Besides negative effects over growth performance, effect of fumonisins on aquatic species. The little FB1 significantly influenced hematocrit, erythrocytes, information available may seem, sometimes, highly hemoglobin, mean corpuscular volume (MCV), variable, even within the same species. However, we mean corpuscular hemoglobin (MCH) and the serum cannot forget that when comparing sensitive levels protein constituents (total protein, albumin and in aquaculture, we may, in many of the cases, be globulin) values. comparing species sensitive levels between a wide In common carp, Pepeljnjak et al. (2003), evaluated range of species with very different ecological and the toxicosis potential of FB1 (500 and 5,000 µg kg-1) biological characteristics. Furthermore, even in young carps (Cyprinus carpio L.; 120–140 g). comparing within the same species, it is well At both FB1 tested levels, Pepeljnjak et al. (2003) described that factors as toxin-related (level and observed loss of body weight and higher incidence duration of intake), animal-related (sex, age, of bacterial dermatological lesions erythrodermatitis general health, immune status, nutritional standing) cyprini (Aeromonas salmonicida subsp. nova) in the
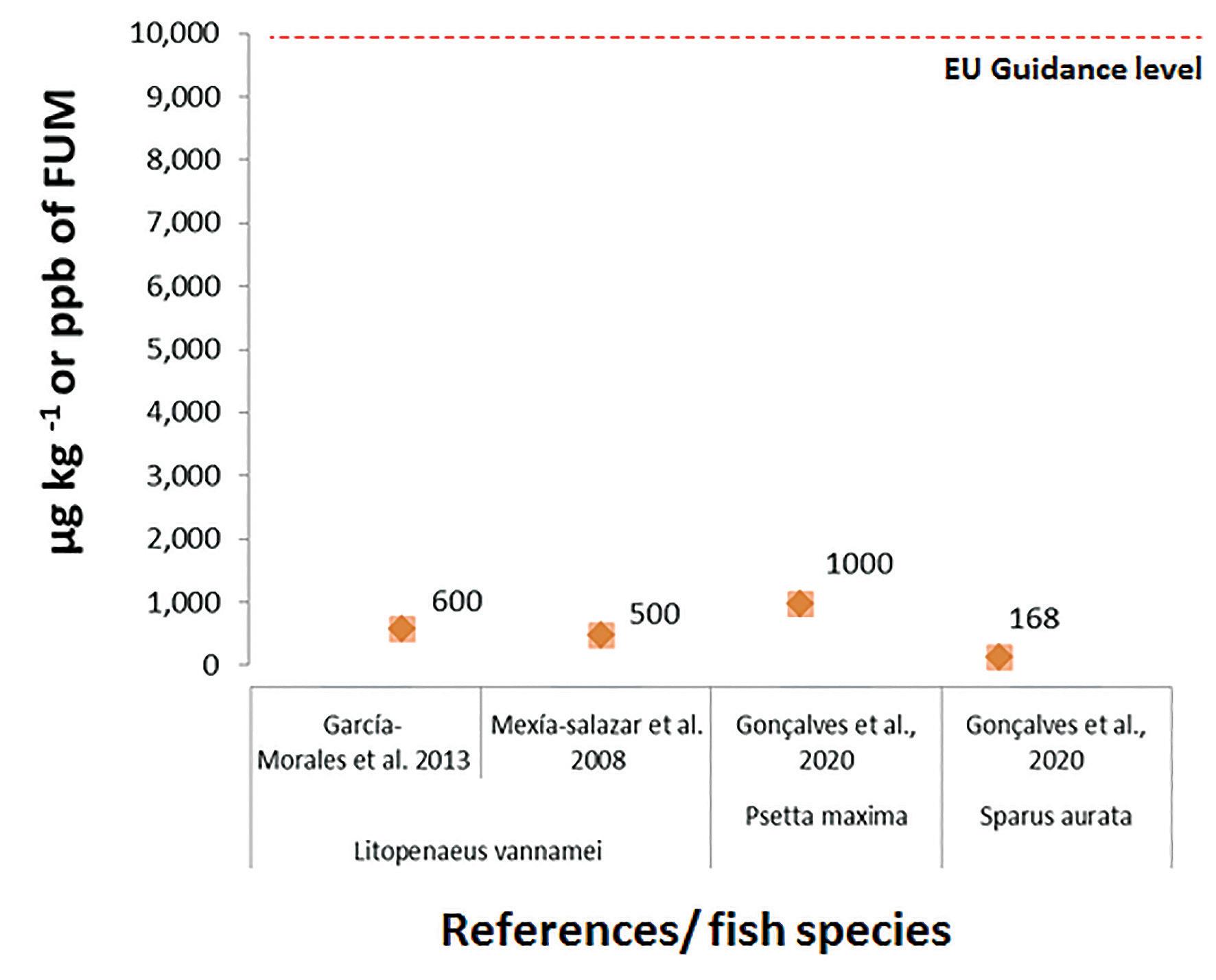
group treated with the higher FB1 dose. Several hematological parameters (erythrocyte count, platelet count) and serum chemical concentrations (creatinine, total bilirubin) and activities (aspartate aminotransferase, AST and alanine aminotransferase, ALT) were altered. The author concluded that while not lethal, contamination levels of 500 and 5,000 µg kg-1 FB1 kg-1 body weight could result in adverse physiological effects.
In other studies (Petrinec et al., 2004; Kovacic et al., 2009) confirmed that 10,000 µg kg-1 FB1 could affect negatively common carp.
The common aspect between all species represented in Figure 1 is their common environment ecosystem, i.e., they are all freshwater species. However, even considering that freshwater species are the most studied group regarding the impact of fumonisins, we are still far from having a full picture of fumonisins negative effects on this group of species. For example, to the present knowledge nothing is known about the potential effect in other important freshwater species, e.g., grass carp (Ctenopharyngodon idellus), the #1 produced species in the world, 10.5% share of all produced species, or silver carp (Hypophthalmichthys molitrix). From the top 5 most-produced species in the world, only common carp and Nile tilapia were studied for the possible impact of fumonisins.
Effect of FUM in marine species Shrimp
Fumonisin B1 has not been extensively studied as a shrimp feed contaminant. However, the few studies available suggest that Litopenaeus vannamei is highly sensitive to FB1 (Fig. 2).
In 2008, Mexía-Salazar et al. (2008), reported that Litopenaeus vannamei fed FB1 as low as 500 µg kg-1 led to marked histological changes in the hepatopancreas as well as tissue necrosis. This author also found for the first time that both electrophoretic patterns and thermodynamic properties of myosin extracted from shrimp exposed to FB1 were modified, being this an indication that shrimp meat quality was negatively affected.
Later in 2013, Garcıa-Morales et al. (2013) re-confirmed Mexía-Salazar et al. (2008) findings for similar contamination levels and further explored the impact of FB1 in muscle. This study showed that white shrimp fed 600 µg kg-1 FB1 had its growth significantly affected. Interestingly, this author also observed that soluble muscle protein concentration decreased, and changes in myosin thermodynamic properties were observed in shrimp after 30 days of exposure to FB1. Moreover, marked histological changes in the tissue of shrimp fed a diet containing FB1 at 2,000 µg kg-1 were also observed. These findings were further reinforced by showing that FB1 fed animals had a greater decrease in shear forces after 12 days of ice storage, therefore, affecting their shelf life and product quality.
To the present knowledge, no information is available about the impact of fumonisins in black tiger shrimp (Penaeus monodon) or any other commercially relevant crustacean species.
Fish
Regarding marine fish, almost nothing is known about the possible impact of fumonisins in this group of species. Only recently, two studies were published, exploring the impact of this mycotoxin in seabream (Sparus aurata) and turbot (Psetta maxima) (Fig. 2). The gilthead seabream is one of the most important fish species farmed in the Mediterranean. Being a carnivorous fish, traditionally fed high levels of fishmeal and fish oil, its trend to fed with cost-effective plant meals to maintain profitability and sustainability is almost unavoidable. Recently, Gonçalves et al. (2020) reported that fumonisin levels as low as 168 µg kg-1 feed had a significant impact on final body weight, feed conversion ratio and protein efficiency ratio. The authors observed that fumonisins also reduced fat and energy retention and disrupted the respiratory burst in circulating leukocytes. At slighter higher levels of fumonisin (i.e., 333 µg kg-1), these detrimental effects were intensified, clearly demonstrating a dose-response relationship.
In other important European carnivorous species, turbot, it was observed that dietary concentrations of 1,000 to 5,000 µg kg-1 of fumonisins in feed, lead to a significant damaging effect on the overall growth performance, nutrient retention, organ morphology and immune status of turbot. Fumonisins tested levels caused a reduction of the brush border villi length in the distal intestine and a reduction of hepatic lipid inclusion (Gonçalves et al., 2020).
Conclusions
While some freshwater species, namely, channel catfish (Ictalurus punctatus), clariid catfish (Heterobranchus longifilis), Nile tilapia (Oreochromis niloticus) and rainbow trout (Oncorhynchus mykiss) seem to be quite tolerant to fumonisins, others like common carp (Cyprinus carpio) and African catfish (Clarias gariepinus) are apparently more sensitive to FUM.
Therefore, not ALL freshwater species may be tolerant to fumonisins.
It is important to understand that extrapolation of findings to other species may be dangerous, as we observed within the group of “catfish” sensitive levels between channel catfish and African catfish was impressively different.
Very little is known regarding marine aquaculture species, including Pacific white leg shrimp in this group. So far, all data report consistently indicates that marine species might be highly sensitive to fumonisins.
Aquaculture is a very diverse industry and will be hard to create guidance values for safe mycotoxin levels in complete feeds that would safeguard all species. Very restrictive guidance values may be practically challenging with possible important economic negative consequences. However, it is important to further understand the impact of fumonisins in commercially important marine species, especially the species using raw materials with a high risk of fumonisins occurrence. Furthermore, for both fumonisins sensitive and nonsensitive species, it would be very important to study the possibility of carry-over effects.
References available on request.
More information: Rui A. Gonçalves
Aquaculture Business Developer Lucta, S.A., Spain E: ruialexandre.goncalves@lucta.com
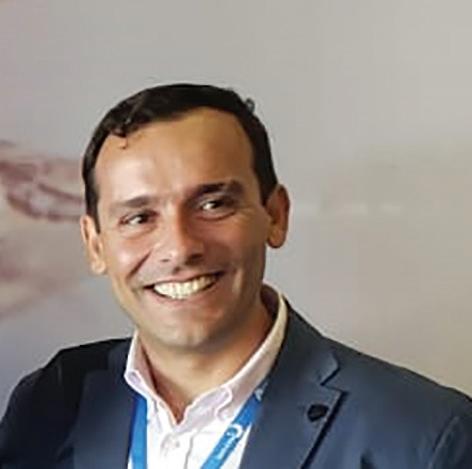
CLEAN FEED. CLEAN WATER.
Wenger Extrusion Solutions for RAS Feed Production
Wenger innovative extrusion solutions deliver clean, durable, nutritional feeds specifi cally designed for the most e cient RAS operations. Feeds produced on Wenger systems maintain their integrity better and longer, for clean and clear water. So you feed the fi sh, not the fi lter. Learn more about the Wenger RAS advantage. Email us at aquafeed@wenger.com today.

PHONE: 785.284.2133 |
EMAIL: AQUAFEED@WENGER.COM | WENGER.COM USA | BELGIUM | TAIWAN | BRASIL | CHINA
Nutritional fish & shrimp pathology - I
Albert G. Tacon, Ph.D.
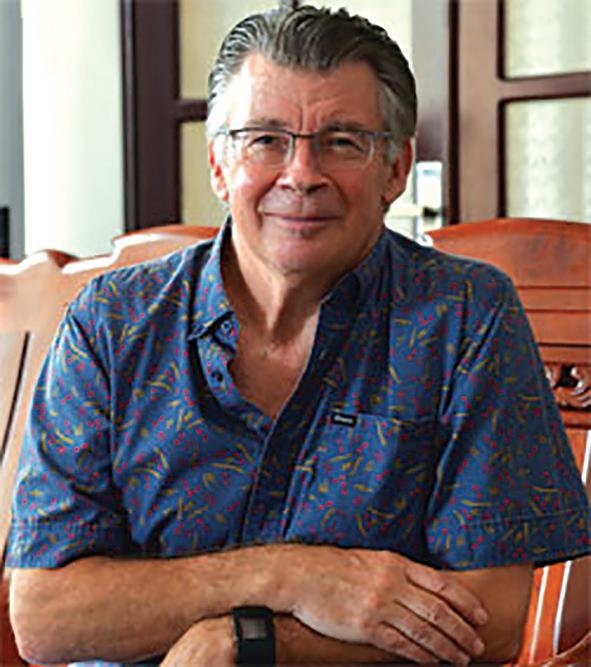
Dr. Albert Tacon is a Technical Editor at Aquafeed.com and an independent aquaculture feed consultant. E: agjtacon@aquahana.com
Disorders in protein & amino acid nutrition
This column is the first of a six-part series dealing with a forthcoming publication by the author dealing with the major reported nutritional disorders in farmed fish and shrimp and represents an update to a previous review published by FAO in 19921 .
Nutrition is the study of all those biological processes of growth, reproduction and maintenance which depend upon the intake and digestion of food. It follows, therefore, that any negative effects on any of these biological processes can result in nutritional disorders, tissue pathologies and can negatively affect fish and shrimp health.
A simplified diagrammatic representation of the possible environmental interactions between nutrition and health in farmed fish and shrimp is shown in Figure 1.
Of particular note, is the negative effect of environmental stress and unfavorable culture conditions on feed intake and dietary nutrient requirements, and, in particular, the potential stressful effects of sub-optimal water quality conditions (temperature, oxygen, salinity, natural food availability, pollutants, algal blooms, weather, storm-water runoff, etc.) and sub-optimal rearing conditions (stocking density, water exchange, sediment build-up, human interactions, disease outbreaks, etc.) on the farmed species. Notwithstanding the above, it is generally believed that the majority of these environmental stress factors can be reduced and minimized by creating a more stable culture environment and management system for the farmed species.
Notwithstanding the above, for the purposes of this mini-series, the major reported nutritional disorders will be summarized and discussed under six main categories, namely 1) disorders in protein and amino acid nutrition, 2) disorders in lipid nutrition, 3) disorders in mineral nutrition, 4) disorders in vitamin nutrition, 5) anti-nutritional factors, and 6) feed contaminants and mycotoxins.
Disorders in protein & amino acid nutrition
Table 1 shows the reported deficiency signs for individual amino acids when present at sub-optimal levels (i.e. below known dietary requirement levels) within formulated diets for different farmed fish and shrimp species. Of particular significance from the data presented in Table 1 is the important metabolic role played by many amino acids on fish and shrimp health, immunity, gut health and disease resistance, and in particular, the functional amino acids arginine, histidine, isoleucine, methionine, phenylalanine, taurine, threonine, tryptophan and valine.
Although specific dietary amino acid deficiencies can be remedied through dietary supplementation with the corresponding limiting free amino acid, it is also apparent from the deficiency signs reported that many amino acids perform other important biological functions over and above to that of just serving as building blocks for proteins and tissue growth (Fig. 2). It follows, therefore, that dietary amino acid requirements for these specific amino acids may be higher when formulating feeds for optimum health and disease resistance, gut health and immunity, stress control and reproductive function.
1 Tacon, A.G.J. (1992). Nutritional fish pathology: morphological signs of nutrient deficiency and toxicity in farmed fish. FAO Technical Paper No. 330, FAO, Rome. 75 pp.
Table 1. Reported amino acid (AA) deficiency signs and health impacts in farmed fish and shrimp.
Major species - AA Reported AA deficiency signs & health impacts1
Arginine
Channel catfish (Ictalurus puctatus) Golden pompano (Trachinotus ovatus) Jian carp (Cyprinus carpio var. Jian) Largemouth bass (Micropterus salmoides) Nile tilapia (Oreochromis niloticus) Red drum (Sciaenops ocellatus) Red seabream (Pagrus major) Senegalese sole (Solea senegalensis) Striped bass (Morone chrysops × saxatilis) Sturgeon (Acipenser schrrenckii x baeri) Turbot (Scophthalmus maximus) Yellow catfish (Pelteobagrus fulvidraco) - Reduced disease resistance & immune response - Reduced disease resistance & immune response - Reduced disease resistance & impaired intestinal immune response - Reduced immune response - Reduced disease resistance & immune response - Reduced immune response & intestinal structure - Reduced immune response - Reduced disease resistance & immune response - Reduced immune response & intestinal functionality - Reduced stress control, disease resistance & immune response - Reduced digestive capacity & intestinal morphology - Reduced disease resistance & immune response
Histidine
Atlantic salmon (Salmo salar) Blunt snout bream (Megalobrama amblycephala) Gian tiger prawn (Penaeus monodon) Grass carp (Ctenopharyngodon Idella) Jian carp (Cyprinus carpio var. Jian) - Cataract - Reduced intestinal health & antioxidant capacity - Abnormal histological alterations in the hepatopancreas - Reduced gill structural integrity & antioxidant capacity - Reduced digestive & antioxidant capacity
Isoleucine
Jian carp (Cyprinus carpio var. Jian) Olive flounder (Paralichthys olivaceus)
Lysine
Atlantic salmon (Salmo salar) Black seabream (Sparus macrocephalus) Cobia (Rachycentron canadum) Milkfish (Chanos chanos) Rainbow trout (Oncornchus mykiss) Silver perch (Bidyanus bidyanus) Yellow Perch (Perca flavescens) Yellowtail (Seriola quinqueradiata) - Reduced intestinal immune response, tight junctions & microflora - Reduced innate immunity
- Loss of appetite - Reduced hemoglobin level - Reduced hematocrit - Reduced appetite - Caudal fin erosion & increased mortality in fry - Reduced hematocrit & hemoglobin concentration - Reduced sperm quality & motility - Reduced hematocrit
Methionine
Arctic charr (Salvelinus alpinus) Atlantic salmon (Salmo salar L.) European seabass (Dicentrarchus labrax) Jian carp (Cyprinus carpio var. Jian) Nile tilapia (Oreochromis niloticus) Yellow catfish (Pelteobagrus fulvidraco)
Phenylalanine
Grass carp (Ctenopharyngodon Idella) - Cataract - Loss of appetite - Reduced innate immune response - Reduced digestive capacity, microflora & immune response - Reduce immune response - Reduced disease resistance & immune response
- Reduced digestive & immune function & antioxidant capacity
Major species - AA
Taurine2
European seabass (Dicentrachus labrax) Hybrid snakehead (Channa maculatus x C. argus) Japanese flounder (Paralichthys olivaceus) Rabbitfish (Siganus canaliculatus) Red sea bream (Pagrus major) Tongue sole (Cynoglossus semilaevis) Totoaba (Totoaba macdonaldi) Turbot (Scophthalmus maximus) Yellow catfish (Pelteobagrus fulvidraco) Yellowtail (Seriola quinqueradiata)
Reported AA deficiency signs & health impacts1
- Reduced immune function - Reduced stress resistance - Reduced oxidative stress response - Reduced stress response - Green liver, increased scale loss - Reduced reproductive performance, antioxidant function & larval quality - Green liver, low visceral fat & fat digestibility - Reduced immune function - Reduced immune function, hemoglobin & hematocrit - Green liver
Threonine
Blunt snout bream (Megalobrama amblycephala) Grass carp (Ctenopharyngodon Idella) - Reduced immune response, intestinal function & hemoglobin - Reduced intestinal morphology & function
Tryptophan
Asian seabass (Lates calcarifer) Ayu (Plecoglossus altivelis) Atlantic cod (Gadus morhua) Brycon (Brycon amazonicus) Chum salmon (Oncorhynchus keta) Common carp (Cyprinus carpio) European seabass (Dicentrarchus labrax) Giant tiger prawn (Penaeus monodon) Grass carp (Ctenopharyngodon idellus) Grouper (Epinephelus coioides) Hybr. catfish (Pelteobagrus vachelli × L. longirostris) Jian carp (Cyprinus carpio var. Jian) Mrigal carp (Cirrhinus mrigala) Persian sturgeon (Acipenser persicus) Pikeperch (Sander lucioperca) Rainbow trout (Oncorhynchus mykiss) Totoaba (Totoaba macdonaldi) White shrimp (Litopenaeus vannamei) - Increased cannibalism in fry - Reduced maturation - Increased aggressive behavior & reduced stress response - Altered aggressive behavior - Cataract, scoliosis - Reduced stress response - Reduced immune response - Caudal fin erosion - Gill antioxidant system disruption - Increased cannibalism - Reduced appetite, digestive function & antioxidant status - Reduced digestive function - Reduced stress response - Reduced stress & immune response - Increased cannibalism in fry - Reduced disease resistance, stress & immune response - Reduced stress response - Reduced protein digestibility & protease activity
Valine
Grass carp (Ctenopharyngodon Idella) Jian carp (Cyprinus carpio var. Jian) Red seabream (Pagrus major) - Impaired gill structural integrity & gill antioxidant status - Reduced digestive function & microbiota - Reduced immune response
1 Major reported deficiency signs and health impacts in addition to reduced growth and feed efficiency. 2 Non-essential amino acid for most non-carnivorous fish species.
Figure 1. Environmental interactions between nutrition and health. Adapted after Waagbo, 1994.
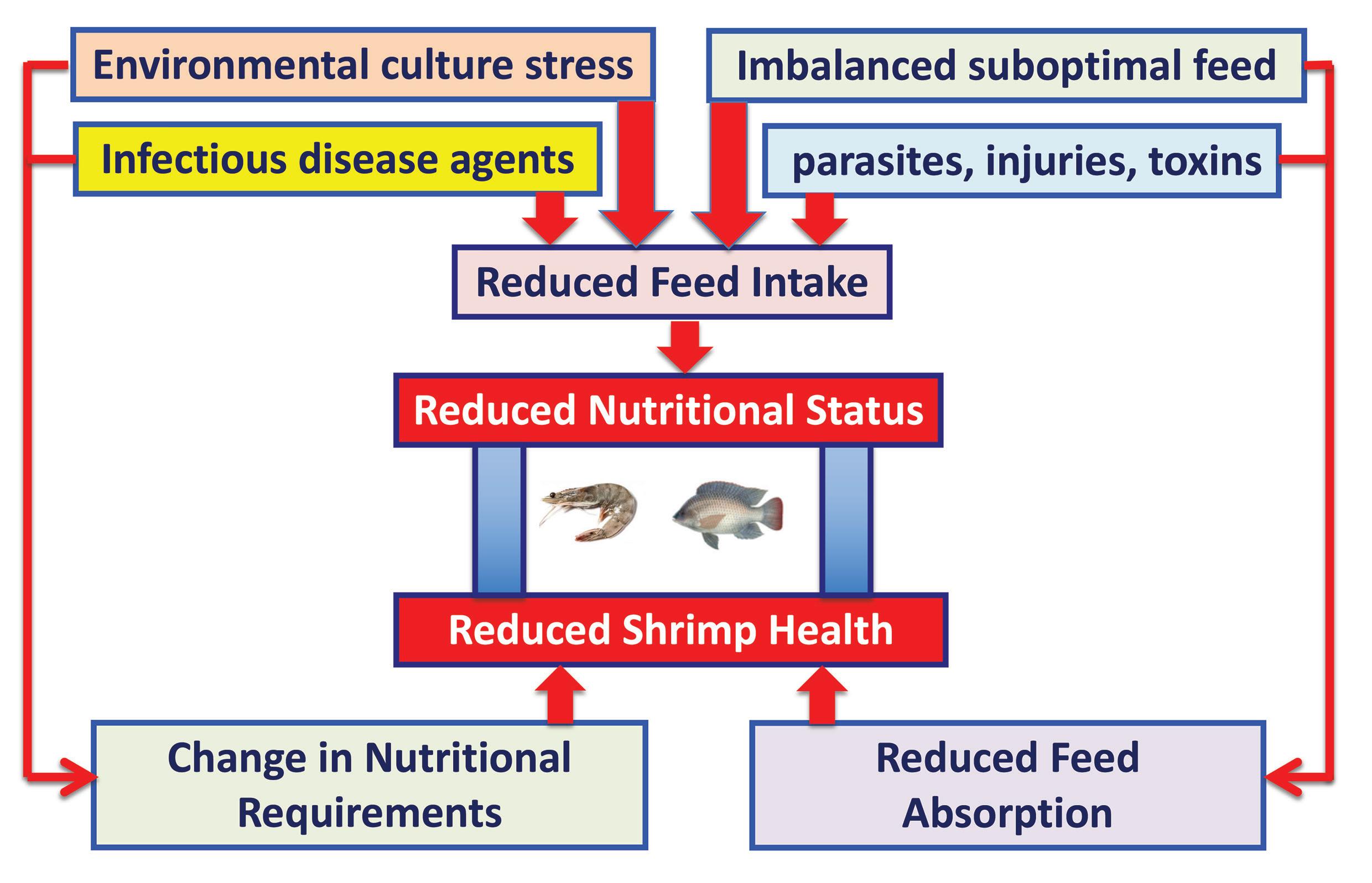
Figure 2. Different roles played by amino acids in the growth, development and health of fish. Adapted after Li et al., 2009.
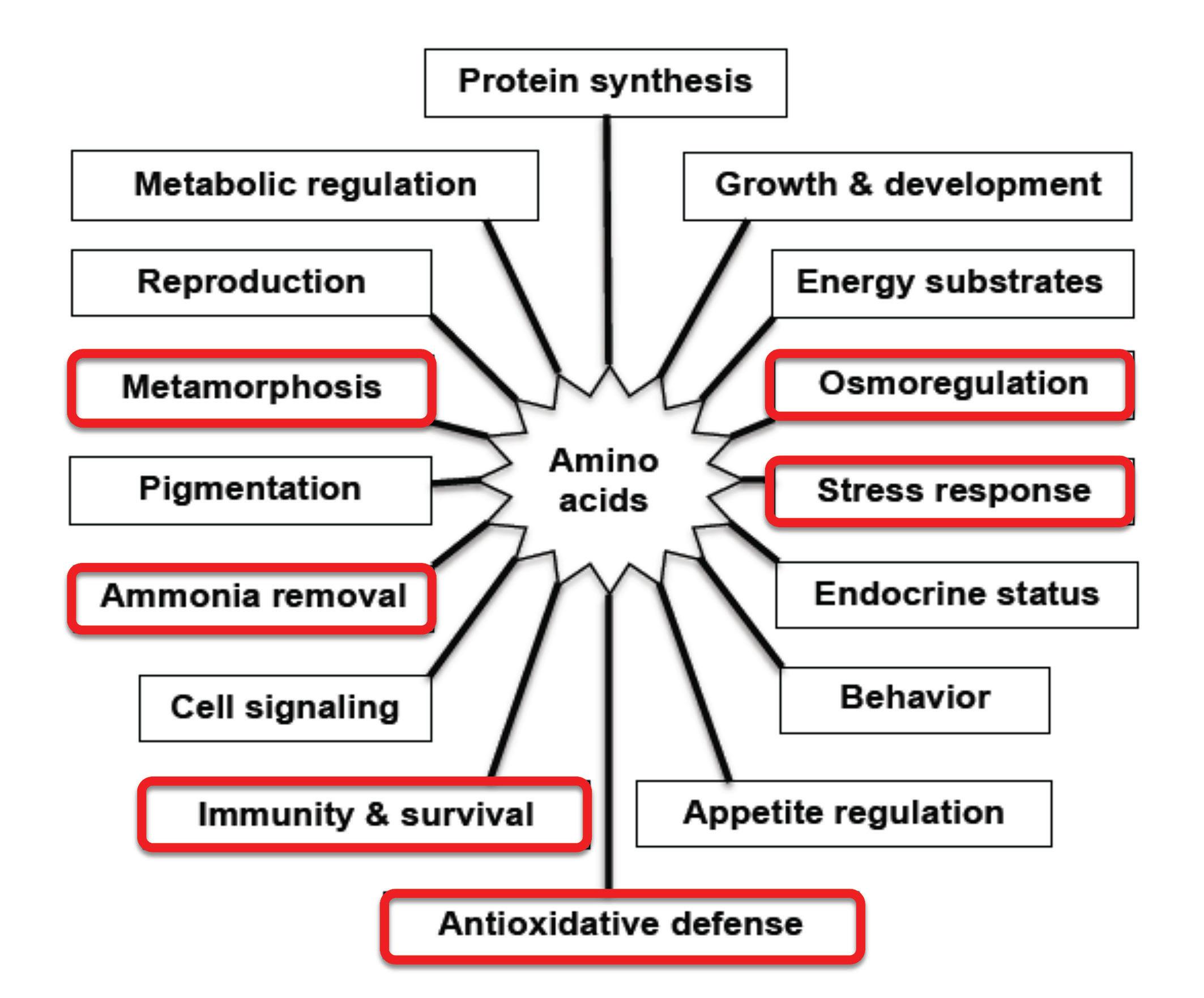