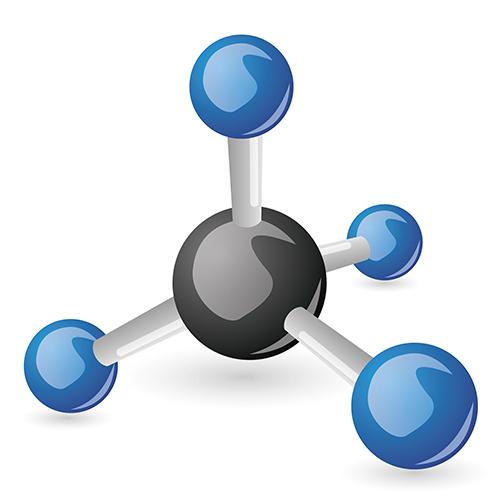
3 minute read
Shapes of Molecules
where an electron has zero probability of being within the orbital so a bonding orbital must have no nodes.
In addition, in dihydrogen, there is a reduction in the potential energy between the two nuclei by 270 kJ/mole or a decrease in the repulsion of the two nuclei by 270 kJ/mole when one electron is between the two nuclei. When two electrons are added into the bonding orbital, one would expect a reduction in the repulsion to be twice that or -540 kJ/mole. This isn’t the case, though, and it’s actually less than this—only about 452 KJ/mole. Why this is the case is because the two electrons repel each other and this repulsion is added to the equation. This is why it is easier than one would think to split dihydrogen or H2 into two atoms of hydrogen ion or H+.
Advertisement
Things get more complicated in larger molecules but, suffice it to say that with any molecular orbital, there is a favorable bonding orbital and an unfavorable anti-bonding orbital created. The bond will happen primarily with a bonding molecular orbital but will not happen with regard to the anti-bonding orbital. This predicts whether or not a bond between two atoms happens.
SHAPES OF MOLECULES
Lewis structures are helpful in determining the arrangement of electrons and bond pairs in that they predict whether a bond will occur. In reality, however, Lewis structures are by nature two dimensional while molecules themselves are three dimensional. Often, it is the three-dimensional shape of a molecule that best predicts the characteristics of the molecule. We’ve hinted at 3D shapes of molecules when we talked about polarity and the fact that water is a polar molecule because it is not a straight-lined molecule.
It is the VSEPR theory or valence shell electron pair revulsion theory that best predicts the shape and geometry of the molecule. The basis of this theory is that, in order to minimize electron-pair repulsion activity, these electron pairs around the central large atom need to get as far apart from one another as possible. Figure 25 describes this when looking at the methane molecule:
Figure 25.
Methane is considered to be tetrahedral with a bond angle of 109.5 degrees between any two hydrogen atoms with the carbon molecule in the middle.
Figure 26 depicts the ammonia molecule, which is different and is considered to be trigonal pyramidal:
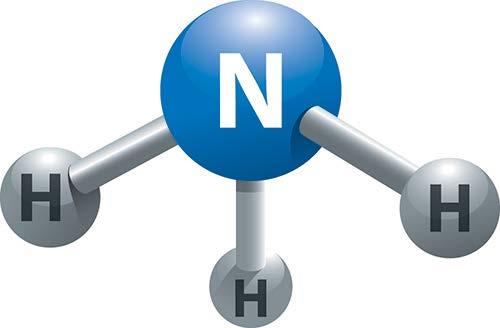
Figure 26.
In this case, there are four pairs of electrons around the central atom; however, one pair is not bonded with anything. The electrons will be 109.5 degrees apart from one another, giving a different shape to the molecule. With water, this is not a straight molecule either. It has a central atom and two lone pairs of electrons, making a bent molecule.
This makes the five possible shapes of small molecules to be linear, trigonal planar, bent, trigonal pyramidal, and tetrahedral.
We’ve talked a little bit about covalent bonds being polar or nonpolar. This can be described by looking at the ability of an atom to attract the shared electrons between the atoms, called “electronegativity”. When two atoms combine, their electronegativities with respect to one another can determine how much each molecule wants to attract that electron pair. If there is equal sharing because the two molecules are the same (as in dihydrogen or dioxygen), the respective electronegativities between the two molecules is the same so this is a nonpolar covalent bond. The extreme of that is the ionic bond.
In between the nonpolar covalent bond and the ionic bond is what’s called the polar covalent bond. This is a bond that is covalent but that involves two atoms with different electronegativities. There is complete sharing of the electrons but the attraction is not equal. There will not be a completely +1 charge and -1 charge; however, there will be what’s called a “fraction of a charge” or sigma positive and sigma negative charges. There is a dipole moment between the two molecules (with the arrow pointing to the more electronegative atom or element).
The difference in electronegativity must be between 1 and 1.7 in order to have a polar covalent bond. An electronegativity difference greater than this is an ionic bond. Polarity can determine the molecule’s boiling point, melting point, surface tension, and solubility. The Lewis structure will help determine this polarity versus non-polarity. Nonpolar molecules have no unshared pairs of electrons, while polar molecules are asymmetrical, having different electronegativities or lone pairs of electrons.
Polar covalent molecules are more likely to have the following:
• Higher melting points than nonpolar molecules
• Higher boiling points than nonpolar molecules
• Greater water solubility than nonpolar molecules
• Lower vapor pressures than nonpolar molecules