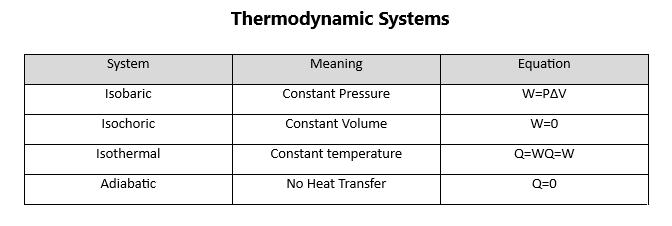
3 minute read
Second Law of Thermodynamics
by AudioLearn
Note that figure 83 is a perfect system in which every process is reversible when, in fact, many thermodynamic processes are not reversible and involve a loss of heat from the system. Later on, we will see a more realistic picture of what this looks like in a fourstroke engine.
In some work situations involving pressure and volume, there can be an adiabatic process, which is defined as a process that has no transfer of heat. This means that Q equals zero. These can only be done if there is a great deal of insulation in the system or if the process is so fast that heat transfer does not occur.
Advertisement
In fact, in an adiabatic process, the change in temperature must be negative because work will be done at the expense of internal energy. An example of an adiabatic process is the release of gas into the air by a pressurized cylinder. The gas will be colder than the gas in the cylinder and there will be less work done. Figure 84 describes these simple engines and the processes involved:
Figure 84.
SECOND LAW OF THERMODYNAMICS
While, in theory, work can be done that is reversible, this doesn’t take place in most thermodynamic processes. In theory, an irreversible process is one that will depend on the path taken. A cold object next to a hot object will never get colder because the direction in heat only goes in one direction—from hot to cold. Friction is irreversible as well because, when it stops an object, it does not cool the object so it moves again. Gases
expand in an area with the molecules evenly distributed. According to the first law of thermodynamics, reversible processes are not forbidden. This is the case, however, with the second law of thermodynamics.
According to the second law of thermodynamics, heat spontaneously moves from high temperatures to low temperatures but the reverse is never possible. Heat goes in a oneway direction from hot to cold and not the other way around. This is just the first expression of the second law of thermodynamics.
This takes us back to heat engines, which include jet engines, gasoline and diesel engines, and steam turbines, which use heat transfer to power them. In a heat engine, there will be heat transfer from a hot reservoir to a cold reservoir with work done as part of this. Figure 85 describes a heat engine and the work done in the system:
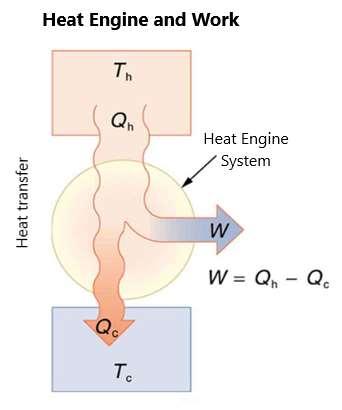
Figure 85.
What figure 85 shows is that heat transfer drives the system, with the work done being the heat transferred into the system subtracted by the heat leaving the system. The heat leaving the system in a heat engine will be positive because no heat engine is perfect and will have no heat output as part of their activity. This leads to the second expression of
thermodynamics: it is impossible in any system for heat transfer to work in a cyclic process whereby the system returns to its initial state.
What this means is that, in a piston system, where the piston goes up and down, or in a rotating turbine system, where there is a cyclic process, there cannot be a perfect conversion between heat transfer into a system and work done. It means that the process will never be 100 percent efficient. The efficiency of a system is the work output divided by the heat input or heat transfer into the system. Because work equals heat input minus heat output, the efficiency can be the described as 1 minus the heat output divided by the heat input. What this looks like is seen in figure 86:

Figure 86.
An efficiency of one or 100 percent is possible only if there is no heat transfer into the environment. All Q will be positive because heat goes in one direction. Ideally the efficiency will be 100 percent; however, this is not the case so that it will be less than 100 percent because there will be some heat output.