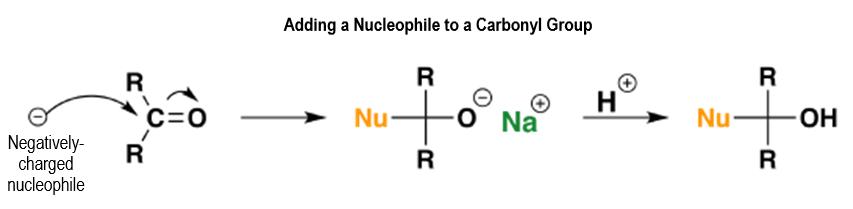
3 minute read
Reactivity of Aldehydes and Ketones
Figure 43.
The nucleophile will be a negatively charged ion or a molecule that has a lone electron pair like ammonia or NH3. As you can see, the first thing that happens in the reaction is that the double bond gets broken with an addition reaction happening after that. Aldehydes, in particular, are easily oxidizable because of the hydrogen attached to the carbon atom. This just doesn’t happen easily in ketones unless there are powerful oxidizing agents involved.
Advertisement
REACTIVITY OF ALDEHYDES AND KETONES
Small aldehydes, like methanal and ethanal will be gaseous or will boil close to room temperature. Larger molecules will have higher boiling points and will be liquid at room temperature. As the molecule gets bigger, there will be more electrons as part of it and there will be increased van der Waals attraction forces. This would be true even if it weren’t an aldehyde or ketone.
The addition of polarity means that there will be dipole-dipole attraction forces, leading to higher boiling points than the corresponding alkane. Consider the 3-carbon alkane, which has a boiling point of -42 degrees Celsius; this increases to 21 degrees Celsius in aldehydes because of polarity. Alcohols will have hydrogen bonding, further increasing the boiling point.
As mentioned, solubility in water with aldehydes and ketones depends mainly on chain length. The small aldehydes and ketones are fully miscible with water because, while they can’t hydrogen bond with themselves, they can hydrogen bond with water. This can be hydrogen bonding between the lone pairs of electrons on the carbonyl oxygen
molecule with hydrogen on the water molecule. All of this falls apart when the chain length gets larger and there is more nonpolarity in the molecule.
A ketone can be reduced through a process called dehydrogenation. Under certain “reduction conditions”, the RCOR molecule can be reduced to RCH2R plus H2O, completely reducing the carbonyl group to make an alkane or similar molecule plus water.
The Wolff-Kishner reduction reaction involves treating an aldehyde or ketone with hydrazine (N2H4), which causes a hydrazone derivative species that, when mixed with heat and a base will lead to a hydrocarbon. This can lead to the making of a hydrocarbon from an aldehyde or a ketone. It essentially hydrogenates the ketone to make a hydrocarbon. Another reduction reaction is the Clemmensen reduction reaction. This involves the use of zinc and an acid like HCl, which again takes a ketone or aldehyde and removes the carbonyl oxygen.
The carbon atom of the carbonyl group has a relatively high oxidation rate already. It can, however, be further oxidized by converting the RCHO molecule to an RCOOH molecule, which is a carboxylic acid. There are several reagents, such as bromine and potassium permanganate, that will help oxidize the aldehyde. Even exposing the aldehyde to air will slowly oxidize it. Saturated ketones, on the other hand, will be generally inert to the oxidation process.
The alpha carbon, which is the carbon atom next to the carbonyl group, is particularly susceptible to substitution in substitution reactions. They can be mixed with Chlorine or Bromine gas along with an acid or base catalyst in order to halogenate the alpha carbon of the ketone or aldehyde. In ketones, the alpha carbon can be on both sides of the carbonyl carbon—both of which are technically reactive. The substitutions involve the loss of a hydrogen atom and the replacement of it with a halogen.
Water can be added to the carbonyl carbon, leading to a geminal-diol, which is relatively unstable. The main exception to this is formaldehyde, which forms a stable geminaldiol. A similar reaction involves the activity of alcohols to aldehydes and ketones to make an unstable hemiacetal. Figure 44 shows the formation of a hemiacetal and an acetal when mixing a ketone with an alcohol: