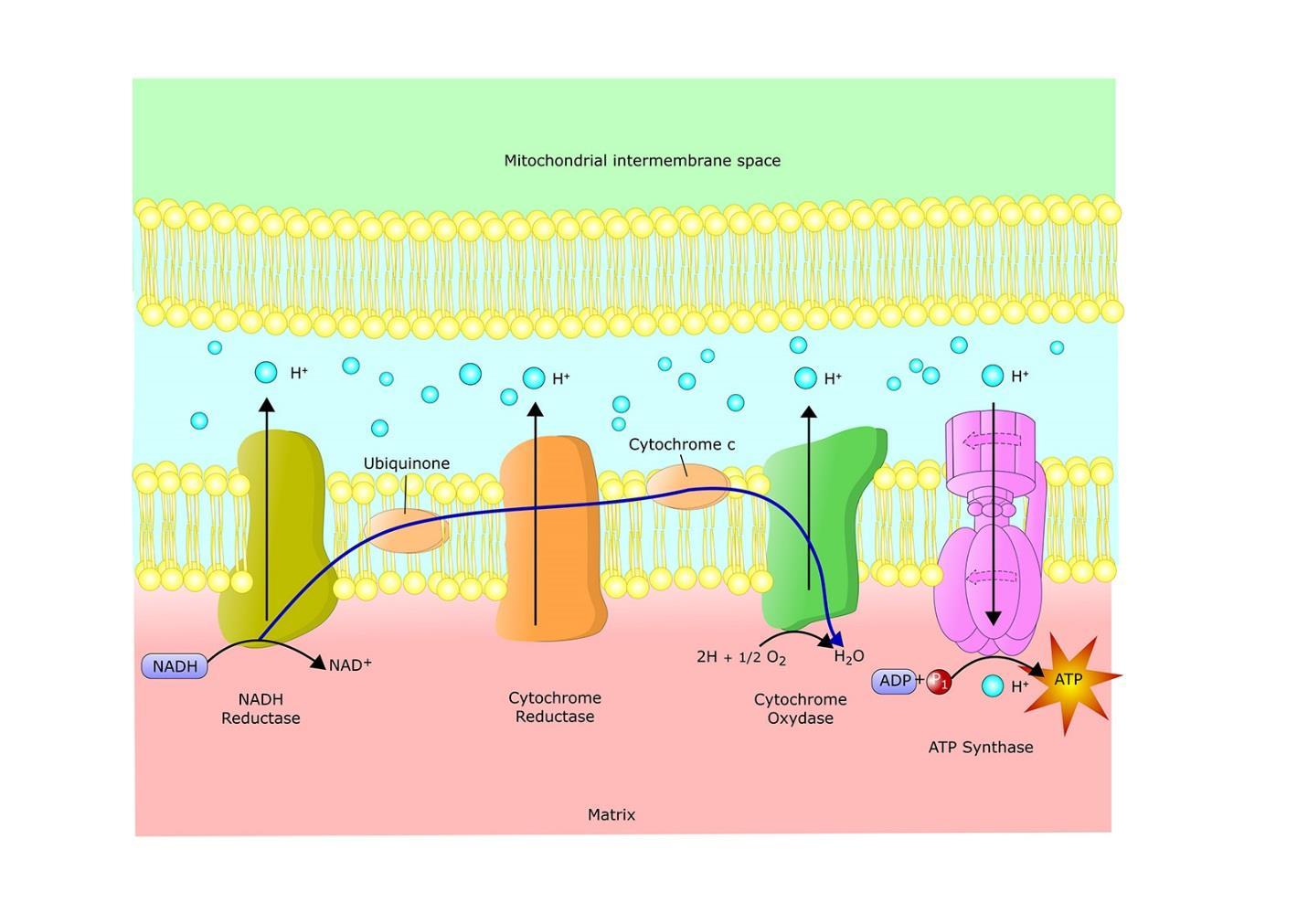
3 minute read
Mitochondrial Respiration
levels are high and ATP levels are low, the enzyme works. If ADP levels are low, the enzyme is not active.
This glucose-1,6-bisphosphate molecule is highly unstable, so that fructose bisphosphate aldose easily cleaves it into two three-carbon molecules. One is called dihydroxyacetonephosphate, while the other is glycraldehyde-3-phosphate. In the next step, an isomerase (which is a rearrangement enzyme) turns the first molecule into glyceralde-3phosphate, ending the first phase of glycolysis.
Advertisement
In the second half of glycolysis, two small three-carbon sugar molecules start the process and energy is created in order to pay back the two ATP molecules used. This leads to a profit of 2 more ATP molecules plus NADH, which has even more energy than ATP.
Glyceraldehyde-3-phosphate gets oxidized, giving up two electrons to make NADH from NAD+. A second phosphorus molecule gets added to the glyceraldehyde-3-phosphate but this does not require ATP. This is a rate limiting step because it depends on the availability of NAD+.
In the seventh step, phosphoglycerate kinase donates the extra phosphate in order to form ATP from ADP. This is referred to as substrate-level phosphorylation. The end result is 3-phosphoglycerate and an ATP molecule. Then the phosphate group gets moved around to make 2-phosphoglycerate. Enolase is the ninth step. It is a dehydration reaction that forms PEP, also called phosphoenolpyruvate plus water. Finally, PEP is acted on by pyruvate kinase to make another molecule of ATP plus the end-product of glycolysis, which is pyruvate. The pyruvate kinase step is another ratelimiting step.
MITOCHONDRIAL RESPIRATION
In glycolysis, ATP is made through substrate-level phosphorylation. As you can see, however, it doesn’t make much ATP and leaves behind pyruvate as an end-product. Most ATP is made in the mitochondria using oxidative phosphorylation. There is a series of reactions that occur after that, through the Krebs cycle, which has oxygen or a non-oxygen inorganic final electron acceptor, depending on the organism. There is a
transfer of electrons that takes place on the inner part of the cell membrane of prokaryotic cells or in the inner membrane of the mitochondria in eukaryotic cells. This leads to an electron gradient that drives the making of ATP.
The final component of mitochondrial respiration is the electron transport system. There is a series of membrane-associated protein complexes and mobile electron carriers that take FADH2 and NADH in a type of bucket brigade that performs redox reactions to create energy. There are four types of electron carriers: flavoproteins, cytochromes, quinones, and iron-sulfur proteins. The electron transport chain is described in figure 42:
Figure 42.
Oxygen is the final electron acceptor in this electron transport chain in aerobic respiration. It involves cytochrome oxidase, which is the last electron carrier. Each type of bacterium has a different cytochrome oxidase, which helps to define them by
molecular biologists. This process is not possible if cytochrome oxidase is not present, if the cell cannot tolerate the oxygen-free radicals made in this process, or if oxygen is not available.
Remember that it does not have to be oxygen as the final electron acceptor. De-nitrifiers are soil bacteria that use nitrate and nitrite as final electron acceptors, making nitrogen gas. Some aerobic bacteria, like E. coli, have the ability to change the acceptor to nitrate instead of oxygen when oxygen is depleted (making nitrite).
The microbial organisms that have anaerobic respiration still have a Krebs cycle so they use the energy held within the NADH and FADH2 molecules. They have a unique electron transport system that generates smaller electrochemical gradients. This means that less ATP is made in the process.
In the electron transport system, some energy is used to pump hydrogen ions across some type of membrane. In prokaryotic cells, hydrogen leaves the cytoplasmic membrane. In eukaryotic cells, it is pumped from the inner mitochondrial matrix into the intermembrane space. Hydrogen ions is positively charged so that there is a difference in electric charge across the membrane. This is called the proton motive force. This also creates a pH gradient. It can do more than just produce energy. It can transport nutrients and can rotate flagella.
This is all tied to ATP synthase, which is how ATP gets made. The hydrogen ions leak back into the cytoplasm in prokaryotes and into the mitochondrial matrix in eukaryotes, changing the configuration of ATP synthase so that ATP can be made from ADP and phosphate. Figure 43 describes how ATP synthase works: