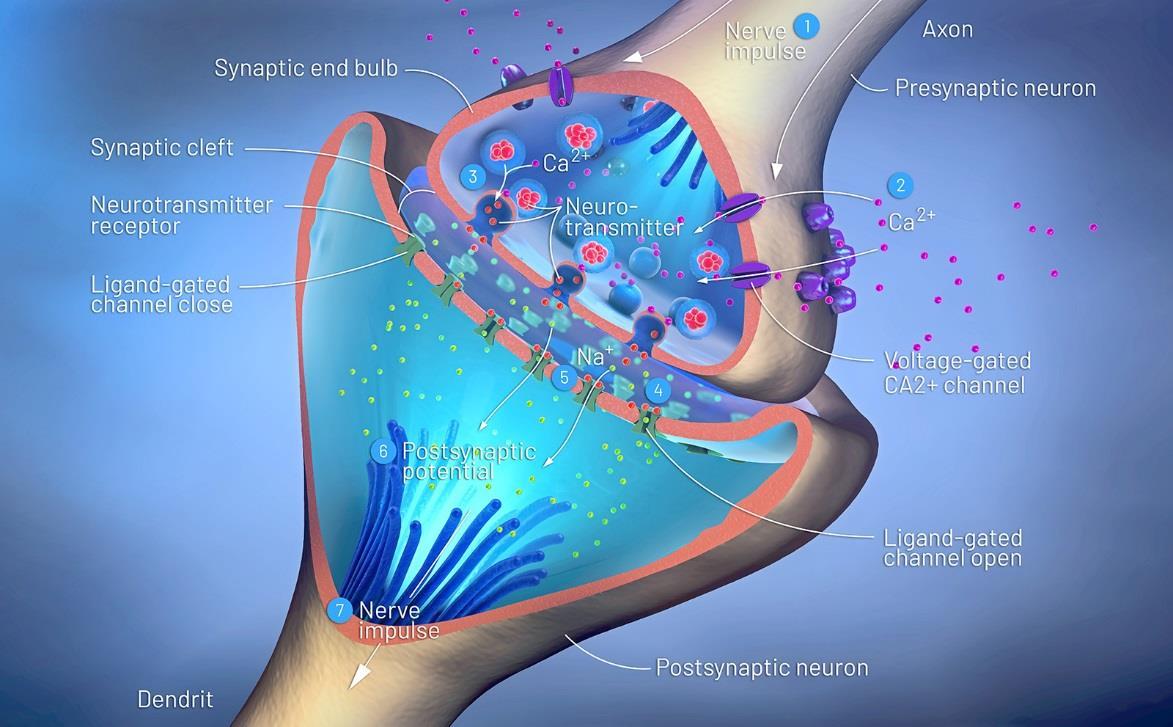
4 minute read
Secretory Pathways in Nerve Cells
As you can see, the SNARE proteins have twisting ability so they can fuse the vesicle to the target membrane. The tethering proteins help them to get in contact with each other. RAB-GTP is one of these tethering proteins. There is an additional SNARE protein involved in order to allow for twisting of these proteins together.
Interestingly, the tetanus toxin protein called tetanospasmin cleaves a specific SNARE protein so that neurotransmitters cannot fuse with the cell membrane. The same is true of botulinum toxin, which prevents neurotransmitter release. These toxins do basically the same thing but have opposite effects on the muscle cell. One blocks inhibitory neurotransmitters, while others block excitatory neurotransmitters.
Advertisement
SECRETORY PATHWAYS IN NERVE CELLS
There is a regulated release of neurotransmitters through exocytosis in the nerve cell. The process by which it does this is similar to all vesicular trafficking. Neurotransmitters are released from small vesicles that take part in many rounds of recycling and fusion at the presynaptic terminals. These are not made in the Golgi apparatus but are made in presynaptic endosomal compartments. The vesicles are filled after being formed, which is different than normal vesicles.
Loaded neurotransmitter vesicles are sequestered in a reserve pool in the cytoplasm or cluster at “active zones” near the presynaptic membrane. When calcium levels rise enough in the cell, the vesicles will discharge into the synaptic cleft. In fact, this is the major trigger for neurotransmitter vesicle release.
Synaptic vesicles need to find the right acceptor membrane in order to discharge. This relies on SNARE proteins that are specific to the vesicle and synaptic cleft, similar to other vesicles. The SNARE proteins are called synaptobrevin, syntaxin, and SNAP-25. There is also a v-SNARE and a t-SNARE protein involved as in other vesicular attachment. VAMP is another protein linked to this type of vesicular transport and attachment.
At rest, neurotransmitters inside vesicles are stored either in the active zone near the synaptic cleft, where the actual neurotransmitters are released. Most neurotransmitters
are held a distance away, held by calcium-sensitive VAMPS (or vesical membrane proteins). They are bound to the cytoskeleton until they can be released.
When calcium rushes into the cell as part of the action potential of the nerve, this triggers the release of the neurotransmitter from the vesicle because the VAMPs are calcium-sensitive. Ultimately, the neurotransmitter leaves the cell to go into the synaptic cleft between nerve cells.
The vesicles can leave once calcium is present because the VAMPs let go of them. Once in the active zone, the vesicles are docked, ready for release. Fusion then happens with the presynaptic membrane getting a small pore that connects to the lumen of the neurotransmitter vesicle. The pore gets larger and then the vesicle membrane collapses, letting neurotransmitters out into the synaptic cleft. This is how exocytosis happens in the nerve cell.
After this happens, the vesicle forms a pit and pinches off to make a new, vacant vesicle. The vesicle is then recycled, where it is filled again with neurotransmitter. It can also get sent back to the cell body of the nerve cell, where it gets broken down to be made into a new vesicle. Figure 50 shows the synaptic cleft and what happens to the neurotransmitters:
Figure 50.
There are two types of neurotransmitter vesicles. One is a small type (about 50 nanometers in diameter) and another is a large type (that is up to 200 nanometers in diameter. Neurotransmitter release happens at the active zones. Only a small number are stored at the active zones. Synapsin I and actin hold the rest of the neurotransmitters further away from the active zones. Synapsin I binds to actin in order to hold the vesicle in place.
Calcium is crucial to the release of neurotransmitters. There are voltage-dependent calcium channels that open when there is electrical activity in the nerve cell. Calcium rushes in and the process begins to take place. Calcium influx is about ten times greater in the active zone compared to other places in the neuron. During an action potential, the calcium concentration increases by 1000-fold in just a few milliseconds.
Synapsin I immobilizes the vesicles until it is time for release of the vesicles into the active zone. It is dephosphorylated at rest and becomes phosphorylated when activated. ATP does the phosphorylation process.
There is an enzyme called calcium-calmodulin-dependent protein kinase that does the phosphorylation of the synapsin I. It works when calcium is present. When calcium is bound, the kinase is activated and synapsin I gets phosphorylated. Vesicles get released by the cytoskeleton to go to the active zone.
VAMPs include synapsin I and synapsin II, although there are others. Two proteins called Rab3a and Rab3b bind GTP and are believed to guide the vesicles to the active zones, where docking can occur. Synaptobrevin is another VAMP that helps recognize the nerve cell plasma membrane.
Two other important proteins linked to this process are called synaptotagmin and synaptophysin. Synaptotagmin binds to phospholipids when calcium is around. Synaptophysin helps to form the pore in the presynaptic membrane. SNAP-25 is a protein that attaches to fatty acids, acting as a SNARE protein when the vesicle docks. Physophilin is a protein on the membrane that also participates in making a fusion pore with the vesicle. Both synaptophysin and physophilin are necessary—they bind together to allow the pore to open.