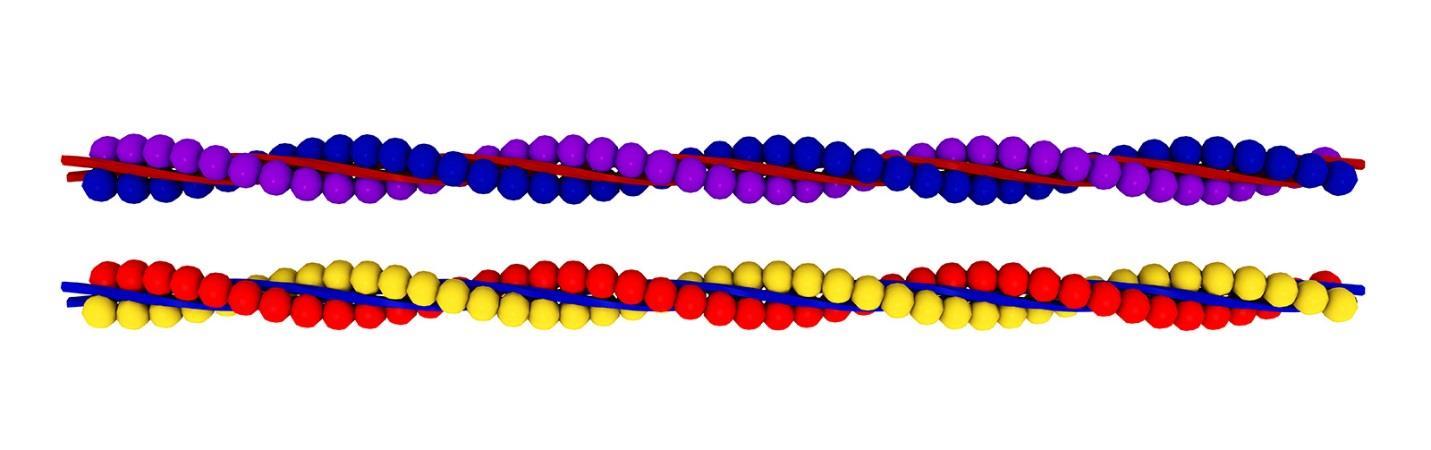
5 minute read
Microfilaments
Intermediate filaments are between eight and twelve nanometers in diameter. They are made from proteins like keratin, lamin, desmin, and vimentin. Lamins are found in the nucleus but not in the cytoplasm, helping to support the nuclear envelope. These are the structures that give the cell its basic shape.
Microtubules are the biggest and thickest of the fibers of the cytoskeleton, being about 23 nanometers thick. These are hollow tubes that consist of both alpha and beta tubulin. It is the microtubules that make the flagella of the cell and the cilia. The centrosome is an organelle that has microtubules sticking out of it. The centrosome is a microtubule organizing center. It separates the sister chromatids during the process of cell division. They also participate in transporting molecules inside the cell. They help to form cell walls in plant cells.
Advertisement
In general, the cytoskeleton functions in several ways. Cells get their shape—even those without cell walls. Cells can move because of the cytoskeleton. Cilia and flagella could not exist and participate in movement without the cytoskeleton. Cells are organized because of the organelle placement by the cytoskeleton. Endocytosis, for example, happens because of the pull of microfilaments that take in the vesicle. Chromosomes move because of the cytoskeleton.
MICROFILAMENTS
Microfilaments are also called actin filaments because they are mostly made of actin. These filaments extend throughout the cell so as to provide structure for the cell and to keep organelles in place. They participate in organelle movement, cell division, cell movement, and muscle contraction. Figure 54 shows what microfilaments look like:
Figure 54.
As you can see by the figure in figure 54, the structure is made from wound-up filaments of actin protein fibers. Actin-G is globular and actin-F is filamentous. These are positively-charged polar substances on one end and negatively charged on the other end. The positively-charged end grows faster than the negatively-charged end. The diameter is about 6 to 7 nanometers.
The structure starts when three actin-G proteins bind together forming a trimer. More actins add to the positively-charged end. There are autoclampin proteins that help these proteins self-assemble. Eventually a spiral forms, which is made of two strands of actin filaments.
A major function of actin filaments is to help contract muscles. They are in a high concentration in muscle cells, forming myofibrils. It is in muscle cells that they are particularly called actin filaments. As you will see, actin interacts with myosin in order to contract a muscle cell. It takes both actin and myosin in order to do this. They cannot do this separately.
As already mentioned, cells move because of microfilaments. This occurs in all cells of the body and is functional in single-celled organisms, such as amoeba. The complex of actomyosin also does this type of movement. Myosin is the motor that drives cellular movement. Microfilaments can also attach to an organelle and will contract so that the organelle can be pulled to another site in the cell.
Mitosis cannot happen without microfilaments. They aid in the pinching off of two cells in the process of cytokinesis that makes two separate daughter cells. In cytokinesis,
there is a ring of actin that cinches off the cell in the middle so that physical separation of the cells can take place. After the shortening and cinching off of the two cells, the actin myofilaments break down into separate actin molecules.
Actin and Myosin
As mentioned, myosin is the motor that drives the contraction of the actin filaments. It uses ATP energy in order to allow the process to energetically happen. Also mentioned is the fact that this actomyosin complex happens in non-muscle cells as well. Figure 55 shows the actin and myosin complex in muscle cells:
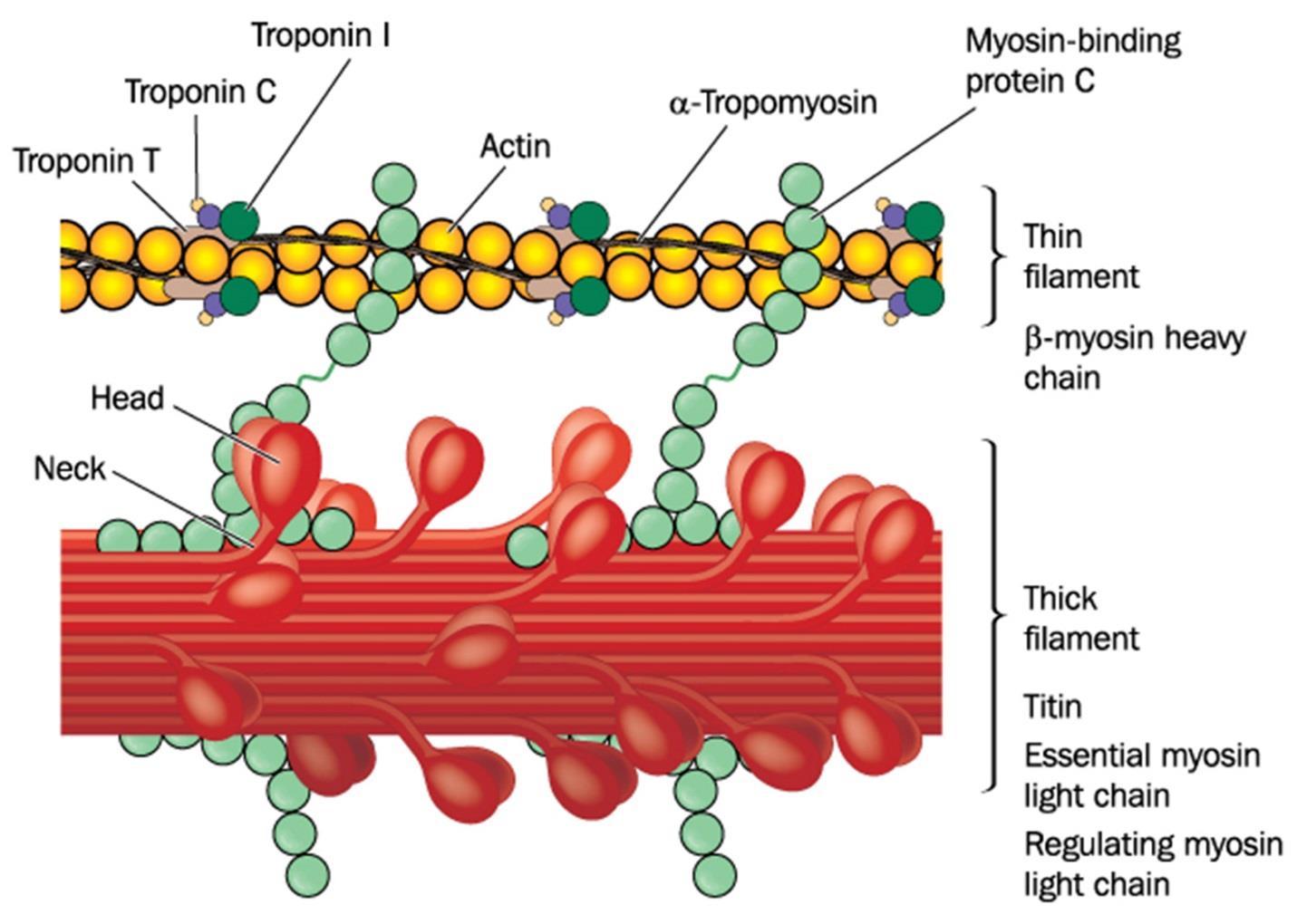
Figure 55.
In humans and other vertebrates, there are muscle cells that can be smooth muscle, heart muscle, and skeletal muscle. Skeletal muscle is the only muscle that is voluntary. Skeletal and cardiac muscle have well-organized actinomycin complexes, while smooth muscle is less well-organized.
Each muscle fiber is a single cell about fifty micrometers in diameter and sometimes several centimeters in total length. The cytoplasm is made from myofibrils, which are thick filaments of myosin and thin filaments of actin. Each myofibril is made up of a contractile unit called a sarcomere.
A sarcomere is just 2.3 micrometers in length, having several regions. The ends of a sarcomere are referred to as Z-discs. There are dark bands visible, called A bands, along with light bands, called I bands. I bands have only actin filaments, while A bands have myosin filaments and actin filaments together. In the H zone, there are only myosin components. The positively-charged ends of the actin attach to the Z disc. There are cross-linked proteins on the ends of the sarcomere that are called alpha-actinin. Myosin filaments are anchored in the middle of the sarcomere at the M line. Figure 56 shows a sarcomere:
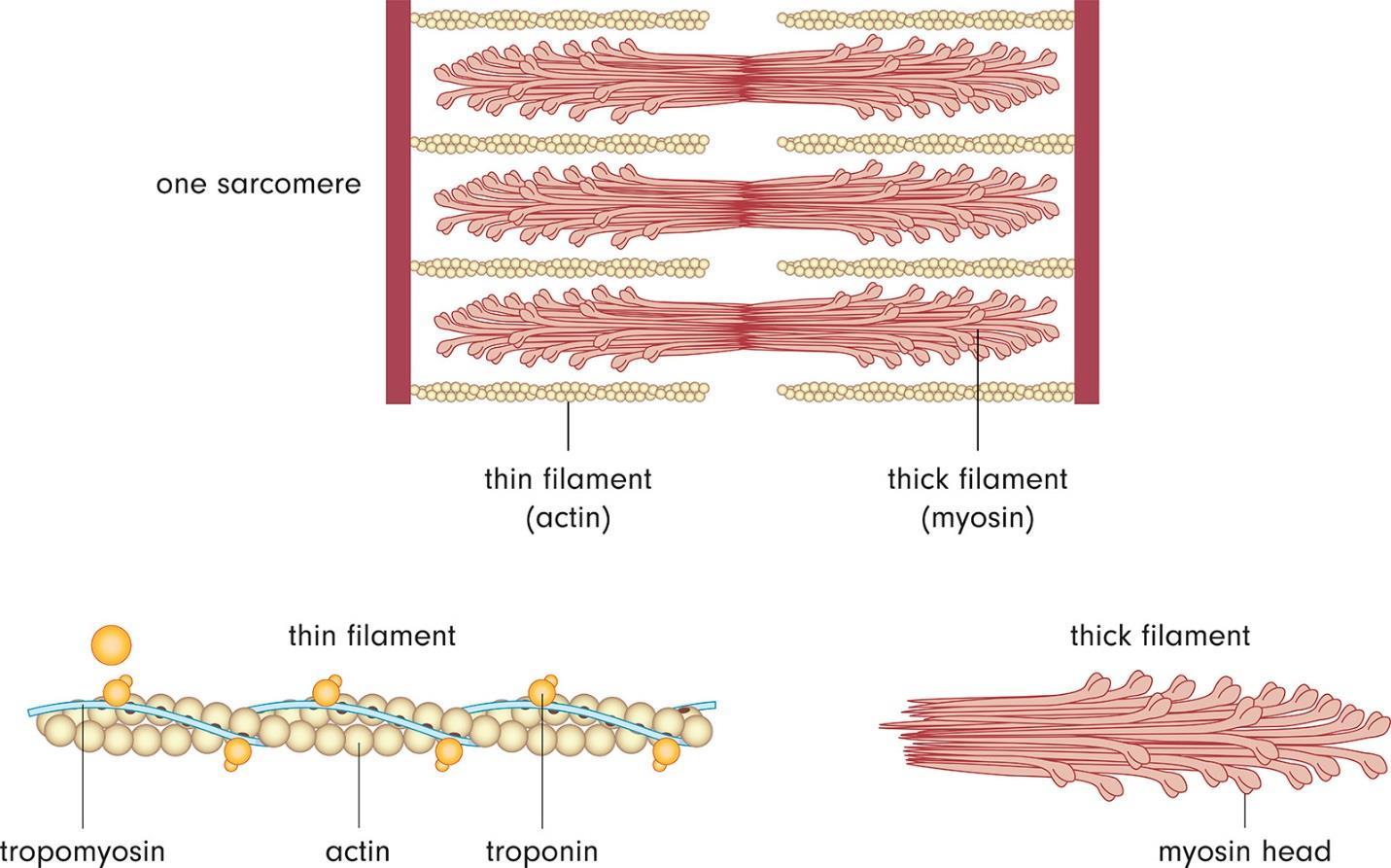
Figure 56.
The proteins titin and nebulin add to the structure and stability of the sarcomere. The titin acts like a spring that keeps the myosin filaments centered. Nebulin is associated with actin and regulates the length of the actin filaments as they grow in the sarcomere.
Muscles are able to contract through the sliding filament model. The sarcomere actually shortens so the Z-discs come closer together. The A band does not change but the H zone and I bands nearly disappear because all of these sections have come together to make the A band.
Myosin II is the type of myosin present in muscle cells. It has two heavy chains and two light chains. It has a globular head region and a narrower twisted molecule as the tail of the muscle. Figure 57 shows the shape of the myosin protein structure:
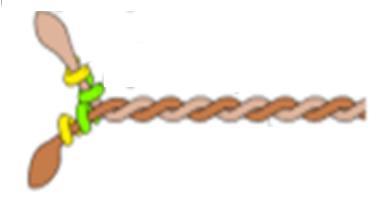
Figure 57.
The myosin fragments are arranged in a staggered fashion with the polarity of the myosin fragments being reversed at the M line, making the interaction between the actin and myosin symmetric throughout the muscle. The actin slides using the myosin motor toward the M line.
Myosin binds to actin as well as to ATP, which is hydrolyzed to provide energy for the filament driving. There is a change in shape of the myosin head that cocks and re-cocks in order to move the myosin head down the actin filament. There is a cycle that starts with myosin bound tightly to actin. ATP binding dissociates this complex. ATP is hydrolyzed so that the myosin head can attach to another myosin segment. ATP hydrolysis provides the power stroke to slide the myosin past the actin.
Calcium is also very important in this process. It is released from the sarcoplasmic reticulum in the muscle fiber when the muscle cell is activated. It interacts with