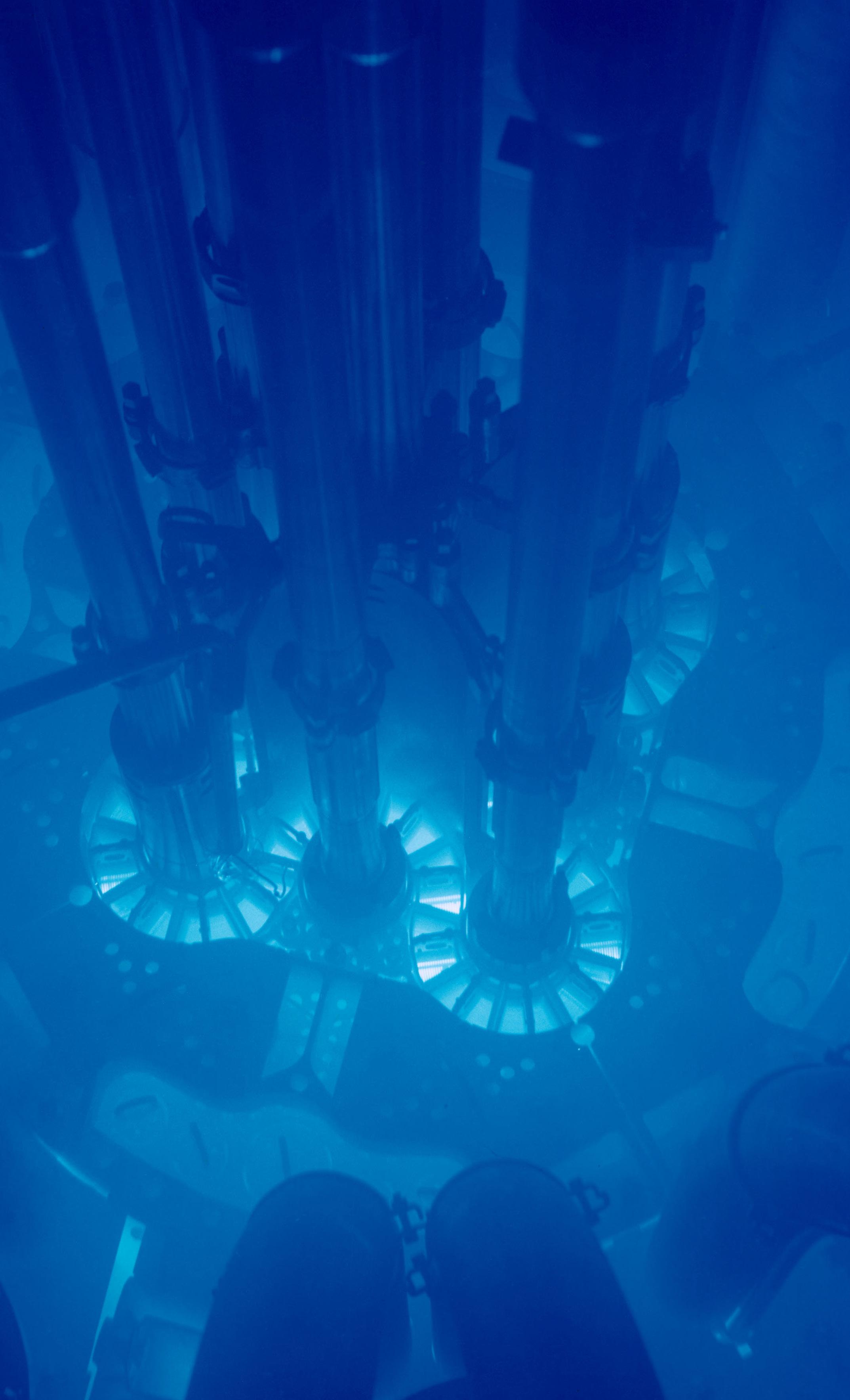
5 minute read
THE POWER OF VISUALISATION FOR CANCER NANOMEDICINE
BRAIN TUMOURS and the need for better therapies
Other than infection, childhood cancers result in the greatest number of diseaserelated deaths in children across the world. Of these, brain tumours have some of the highest incidence in children with over 5000 cases annually in the US alone.
Brain tumours have very poor survival, typically 12-18 months from diagnosis. Current treatment for brain tumours requires an aggressive combination of toxic chemo- or radiotherapy, with surgery. Despite treatment advances, there are very limited chemo- or radiotherapeutic options for brain cancers, and whist surgery can help to remove the main tumour mass, cancer often returns in 95% of patients.
This is due in part to the aggressive nature of brain tumours which can invade deep into the brain, their fortified location behind the blood-brain barrier, and the difficulty for surgical removal of surrounding brain tissue, which may have a significant impact on the patient’s quality of life.
For kids, brain stem tumours like diffuse intrinsic pontine glioma (DIPG) cannot be surgically removed, and diagnosis with this tumour has yet to see any longterm survival.
Effective treatments which can target these tumours continue to be of high, global priority.
The Promise of CANCER NANOMEDICINE
Nanomedicine (the medical application of nanotechnology) holds promise to revolutionise cancer therapy, by designing drug delivery vehicles (nanoparticles) which can target tumours whilst sparing normal tissue. Here, the power of microscopy can aid us in visualising the complex tumour environment during nanomedicine delivery.
This can help us to understand not only how tumours develop, but to also reveal clues to targeting tumour cells.
By combining this with the study of drug delivery and drug release, we have an invaluable platform to advance the development of nanomedicines and triumph over tumour progression.
My postdoctoral research project was focussed on tying these disciplines together, using advanced imaging methods to visualise nanomedicine uptake into 3D tumour models via microscopy. Whilst a promising area of research, our studies were challenged by how we were labelling our nanomedicine drug vehicles, using fluorescence, to track and follow them during tumour uptake.
For small molecule drugs, which are often ideal for delivery into the brain, using fluorescence labelling is akin to attaching a fridge to a Labrador retriever and expecting them to go for the same walk; it simply is not an option.
New frontiers: CERENKOV LUMINESCENCE IMAGING
Unlike fluorescence imaging-based techniques, Cerenkov luminescence imaging uses radiolabels rather than fluorescent labels to monitor drug delivery and tumour uptake.
Cheaper and more accessible using optical cameras instead of million-dollar scanners like those for other luminescence imaging approaches currently in clinic, this would be optimal for studying the delivery of nanomedicines.
Moreover, using combinations of specific radiolabels, we can also visualise and understand the metabolic pathways targeted during drug delivery in real time.
MEMORIAL SLOAN KETTERING and FULBRIGHT
Working at the nexus of cancer nanomedicine and imaging, I was undeniably drawn to the untapped potential of Cerenkov luminescence for visualising drug delivery in brain and other cancers.
The innovation of this technology for imaging in cancer is very new, and so there are only a handful of groups in the world who specialise in it.
Of these, there is only one group who are also deeply interested in cancer nanomedicine and how cancer cells can be targeted based on their changing metabolic pathways.
That group works in the lab of Professor Jan Grimm at the Memorial Sloan Kettering Cancer Center in New York – my Fulbright host.
Having first met Professor Grimm in 2019, he quickly became an influential mentor in my early career. With our aligned research interests and his renowned expertise in Cerenkov luminescence,
I am very excited to finally be able to work together to push the boundaries of Cerenkov luminescence and extend its application in the cancer nanomedicine field.
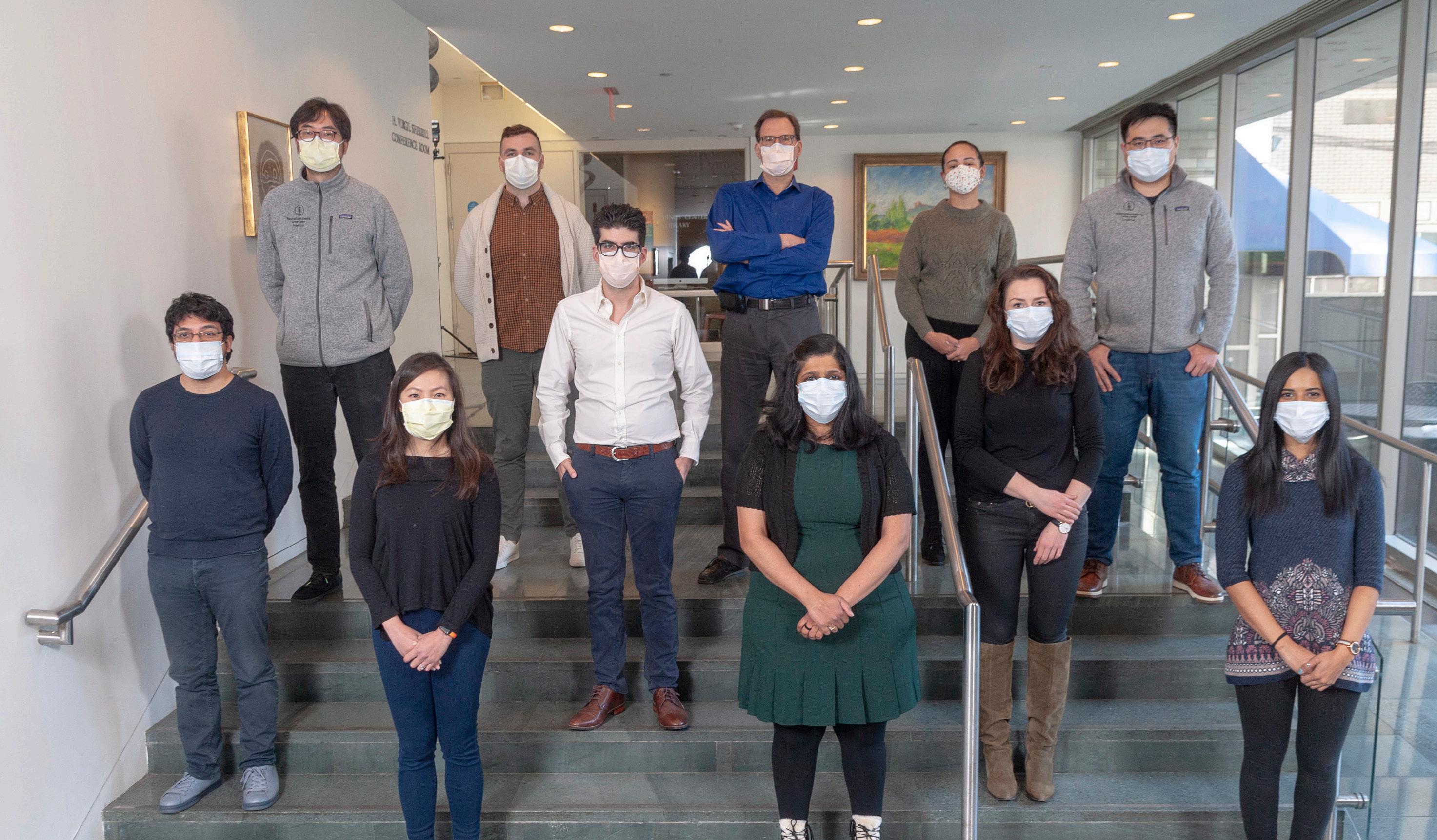
Key Goal: NEW IMAGING for NEW TREATMENTS
This Fulbright Future Scholarship will combine materials and expertise from both Australia and the US, using Cerenkov luminescence imaging to study the tumour uptake and efficacy of copper and ironbased nanomedicines.
Why copper and iron? Both of these are key minerals needed by our cells to breathe oxygen, produce energy and undergo growth and replication.
Recently, it has been found that many tumour cells, including those in the brain, increase their uptake of copper and iron to fuel their own growth. Thus, we at the Children’s Cancer Institute, and at Memorial Sloan Kettering, have been using copper and iron-based nanoparticles for preferred tumour uptake and tumour killing.
Together, we will combine these nanoparticles and look at their effect in tumours using Cerenkov luminescence imaging.
This project is key to enable us to expand our evaluation of nanomedicines for tumour delivery, particularly to the brain. By prioritising these for further clinical testing, we aim to ultimately improve therapies and save the lives of patients, children, and adults alike, who are suffering from these aggressive cancers.
I am incredibly humbled to be able to work with Professor Grimm and his team at MSKCC, and beyond delighted to build on this collaboration during and after my fellowship.
DR ARIA AHMED-COX
is a Postdoctoral Researcher and Imaging Scientist at Children’s Cancer Institute and Katharina Gaus Light Microscopy Facility at the University of New South Wales.
As a Fulbright Future Scholar, Aria will collaborate with research clinician Professor Jan Grimm and his team at the Memorial Sloan Kettering Cancer Center in New York, where she will employ an imaging technique called “Cerenkov luminescence” to visualise the uptake of cancer drugs into solid tumours. Her aim is to use this information to design more effective, less toxic treatments that improve the outcomes for children with these deadly diseases.