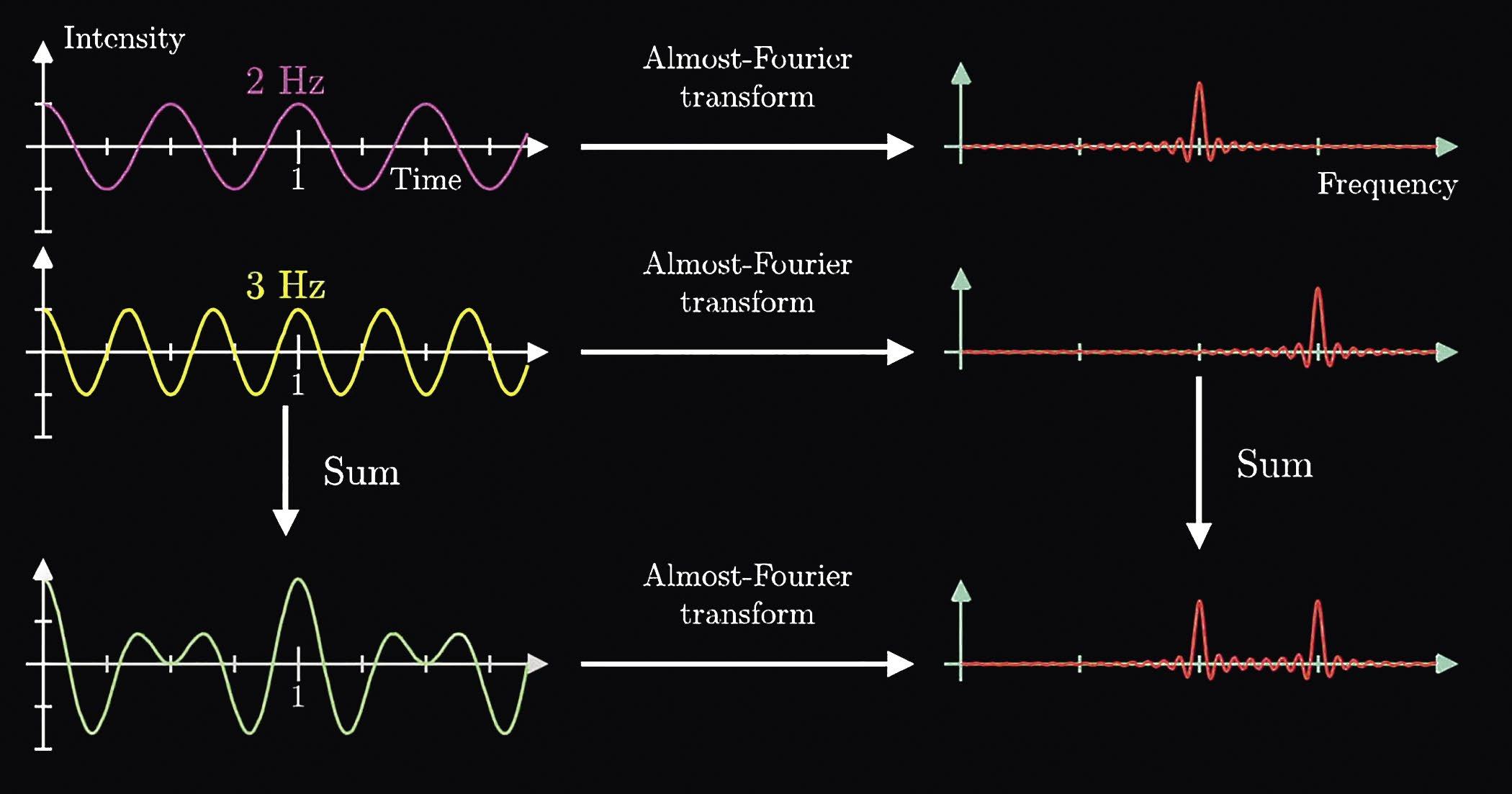
15 minute read
T.U.T.O.R
What Are Advanced Methods for Corrosion Monitoring?
Orin Hollander, Ph.D.
The purpose of this article is to review methods for monitoring corrosion in industrial water systems. The emphasis is on higher purity water systems such as are used in process water in microelectronics, pharmaceuticals, and medical devices. These are important applications for middle market situations. The requirements for these and related manufacturing are very stringent. Consequently, the importance of ever more sensitive analytical methods increases. Wet chemistry and many instrumental techniques are simply not up to the task of ensuring water quality of the necessary purity.
Most of the methods discussed in this review are more advanced than wet chemistry and coupons, or even continuous corrosion rate meters. Technology has brought the cost of these methods down so that they are within reach of small water treatment companies, and expert systems software has made their execution and interpretation accessible to the typical water treatment technologist. In addition, where it is impractical to acquire these capabilities, contract laboratories often can provide the capability and expertise to support the water treater’s needs.
While most of the methods discussed are primarily intended for high-purity waters, two methods (EIS and FTIR-ATR, defined below) are applicable to all water treatment situations, from cooling and boiler water to high-purity process water. Each method is briefly described, including what it does, what information it provides, comparisons of sensitivity and dynamic range, and pros and cons of its use. Industrial cooling systems are so full of contaminants that extremely high sensitivity is superfluous; parts per million (ppm) levels are usually sufficient. But in pharmaceutical systems, medical devices, and chip manufacture, these methods are indispensable. For example, as line widths in integrated circuits get smaller and smaller, contaminants in the low-parts per billion (ppb) or mid-parts per trillion (ppt) range become important. Methods described here are ion chromatography (IC), Fourier transform infrared-attenuated total reflectance (FTIR-ATR), X-ray fluorescence (XRF), X-ray diffraction (XRD), electrochemical impedance spectroscopy (EIS), and anodic stripping voltammetry (ASV). Older, more familiar methods, such as linear polarization or Tafel polarization, have been extensively covered for many years, so they are not included here.
The detailed discussion of each of these methods will be the subject of future articles where a more in-depth understanding will aid in method selection by the technologist. The methods are classified as liquid and surface, and each classification is divided as analytical or mechanistic.
Liquid These methods include IC, EIS, and ASV. Only EIS is mechanistic.
Anodic Stripping Voltammetry This method is for ultra-low concentrations of metal ions and is applicable mainly to microelectronics and medical device manufacturing. It has a sensitivity in the low-ppt range and is applicable to metal ions of atomic number greater than sodium. The equipment for this technique is available as a turnkey system and at a cost of around $25,000.
In this technique, a measured volume of the analyte is introduced into a cell where the anode is a mercury pool. The solution is vigorously stirred while maintaining a cathodic potential where all the metal ions of interest are reduced to the metallic state. The metal atoms dissolve in the mercury but remain at the surface, as diffusion into the mercury bulk is very slow. After the metal ions are exhaustively reduced, the voltage is scanned in the anodic direction using a ramp signal. As the voltage
reaches the potential of oxidation for a given species, there will be a current transient, and the area under the curve will correspond to the total amount of that species. The integrated current is the total charge, the total charge is related by moles of metal = Q/F, and Q=∫idT. The concentration can be calculated as shown in Equation 1.
∫idT x at. Wt. /F/volume of analyte = Concentration Eq. 1
Where:
F is the Faraday constant = 96,485 coulombs per mole.
The potential of the transient corresponds to the reversible potential for that metal when corrected for the reference potential.
Figure 1 shows a typical ASV trace for multi metals.
Figure 1: Typical ASV trace for multi metals.
current / nA
800
700
600
500
400
300
200
100
0
Zn+2
deposition potential from -1.2 to 2.4 V
Cd+2
Pb+2 Ca+2
-1.3 -1.2 -1.1 -1.0 -0.9 -0.8 -0.7 -0.6 -0.5 -0.4 -0.3 -0.2 -0.1 0 0.1 potential V vs Ag/AgCl
Ion Chromatography This method measures ions, cations, and anions in a solution with a tremendous dynamic range, from high ppm to low ppb. As such, it is almost a “one-stop shopping” method. Instrumentation is turnkey, and cost is in the $10,000 range.
Anions and cations are analyzed separately on different columns containing either anion or cation exchange resins. The sample is injected into a port, and the ions are eluted with an appropriate electrolyte optimized to provide separation of ions. The detector is based on conductivity. Separation is achieved due to the relative affinity of each ion for the resin in that eluent. Electrochemical Impedance Spectroscopy This is a very powerful tool for investigating corrosion and inhibition mechanisms. In cases where the corrosion inhibitor program is not working or working poorly, this can be a powerful investigative tool for understanding what is going on. The method uses a corrosion cell and a potentiostat, but this is where the comparison with potentiostatic polarization ends.
EIS involves passing a low amplitude AC current through the cell at various frequencies, from less than 1 Hertz (Hz) to more than 10,000 Hz. The output will be an attenuated voltage but also a phase shift. The input signal is sinusoidal as is the output, but there will generally be a phase shift between the input and output. For example, when the input signal is at 90 degrees (i.e., a maximum), the output signal may be at 0 degrees, or 45 degrees or any value from 0 to 360 degrees. Accordingly, a phase lock detector is needed for the instrumentation.
Here is the basis for mechanistic interpretation. Imagine a box containing a circuit composed of resistors, capacitor,s and inductors in various serial and parallel configurations. If you pass a DC current through the box, all you will be able to measure is the equivalent resistance of the circuit. But, if an AC current is passed through the box with amplitude and phase shift measurement, and the signal frequency is scanned over some range, you can in principle determine the circuit architecture and the value of each component and its resistance, capacitance, and inductance. Some contributors to corroding surface behavior will involve diffusional elements. This too can be measured, and the frequency (i.e., the corrosion rate) where diffusion takes over as the rate-determining step can be observed.
A corroding interface is analogous to a circuit with resistive, capacitive, inductive, and diffusional components. For example, a surface film of calcium carbonate is equivalent to a capacitor in parallel with a resistance (Rp,), which is the polarization resistance. The thicker the film, in general, the lower the capacitance. By measuring film capacitance over time, one can tell if the film is stable or is degrading or growing too thick. It is easy to see that this is a powerful tool.
Instrumentation and expert system software for examination are readily available in the $25,000 range.
However, interpretation of experimental results requires skills above a layman’s capabilities. Figure 2 illustrates a Randles cell of a corroding interface, and its impedance behavior—both in phase (real) and out-of-phase (imaginary) components. The Randles cell is simply the electronic equivalent of the simplest example of a corroding interface. It comprises a solution resistance in series with a parallel polarization resistance and a film capacitance. It reflects the fact that the current has two pathways: corrosion current and capacitive film charging.
Figure 2: Randles cell of a corroding interface (a) impedance plot, imaginary (out of phase) component versus real (in-phase) component at various frequencies (b).
(a) Circuit model
R2 R1
C1
(b) Nyquist plot
Zing (Ohm)
High ω Low ω
R2
0 50 100 150 100
Zreal(Ohm)
Here, solution resistance is in series with a parallel polarization resistance and double layer capacitance. This is the simplest corrosion cell. Note that the corrosion rate is determined by measuring Rp and applying the Stern-Geary Equation for linear polarization. Note also that solution resistance (Rs) is directly measured and can be factored out. Typical corrosion rate meters do not compensate for solution resistance, so in low-conductivity waters, a linear polarization corrosion rate meter will measure the solution resistance primarily, and badly underestimate the actual corrosion rate. If Rs >>> Rp, then the measured resistance is Rs + Rp ~ Rs .
Solid Phase XRD and XRF are analytical, while FTIR-ATR is primarily mechanistic.
X-Ray Diffraction This technique involves identification of ionic solids by means of X-ray diffraction. Each crystalline solid has a regular spacing of ions (e.g., calcium carbonate [CaCO₃]), which has the following crystal structures (calcite and aragonite), as shown in Figure 3.
Figure 3: Aragonite and calcite crystal structures.
Incident plane wave
kl
dhkl Θ Β
Ghkl
Θ Β
kl Scattered plane wave
A Θ Β
Θ Β B Θ Β O Θ Β (hkl) lattice planes
Source: Figure in Practical Electron Microscopy and Database, an online book (https://www.globalsino.com/EM/).
If you have ever driven past a corn field, you will see that regularly spaced rows can be observed from different angles and that the spacing between the rows at different angles is different also. The spacing between the rows of atomic crystals is on the order of the wavelength of X-rays. The following diagram shows how monochromatic X-rays behave when the angle that the beam makes with the crystal leads to constructive interference (Figure 4).
Figure 4: Monochromatic X-ray behavior that occurs when a crystal interferes constructively.
(a)
Aragonite
(c)
Calcite
b
c a
(b)
b
c a
(d)
Source: Soldati, A.; Dorrit, J.; Glatzel, P.; Swarbrick, J.C.; Geck, L. (2016), www.esrf.eu/home/news/spotlight/content-news/spotlight/spotlight263.html.
The d-spacing between successive parallel planes of the crystal is calculated as nλ=2dsin(θ), where λ is the wavelength of the X-ray beam, n is an integer, and θ is the angle between the plane and the normal to the plane, as shown in the diagram.
The distance AOB is the difference in the path length of X-rays scattering off two adjacent rows. If that path length, =2d sin(θ), is equal to an integral number of wavelengths, then the scattering is constructive; any other angle results in destructive interference. The angle θ, which coherently scatters the X-ray to give an image, is known as the Bragg angle. Every ionic species has a “fingerprint” of Bragg angles and intensities, and there are catalogs of Bragg angles and intensities for thousands of ionic crystals (this one is free: http://www. crystallography.net/cod/). In this way, the species can be identified. Mixtures can also be identified by determining the fingerprint of the dominant species from the pattern; what is left is due to the other species.
Imagine two corn farmers whose seeding machines have different spacings. The resulting corn fields will look different from any angle. By measuring the spacings between rows at different angles, one can tell if the field belongs to Farmer Jones or to Old MacDonald!
X-Ray Fluorescence This is a nondestructive method for determining atomic species (elemental analysis as opposed to molecular species). The principle of XRF is that high-energy X-rays can eject inner shell electrons from an atom, as opposed to valence shell electrons, which are ejected by lower energy excitations, such as heat in visible atomic absorption/emission. Ejecting an inner shell electron leaves a “hole” in the atomic electron configuration, and that hole is then filled by an electron from a lower energy shell “falling” into that hole with the emission of an X-ray photon. That photon will have energy (hν) equal to the difference between the hole energy level and the electron energy of the relaxing electron shell. Characteristically, that relaxation is delayed, leading to an extended emission process, hence fluorescence. Also, the emitted photon is necessarily of lower energy (longer wavelength) than the excitation photon.
The emission lines are characteristic of each atomic species. However, the spectra can be complex due to the presence of other elements.
Previously, the technique required complex equipment that was only found in laboratory contexts. But today XRF “guns” are readily available in the $30,000 range. These units can be used in the field on a wide variety of samples without sample preparation. They provide a real-time output of the atomic composition of a sample by type and atomic percentage.
In principle, one of these devices can be used in a plant to determine alloy composition of materials, chemical analysis of liquids, contamination of dirt or fuel, coupon films, etc. For example, heavy metal composition of coal or fuel oil, extent and distribution of toxic elements from an emitter such as a smokestack, verification of alloy composition. The possibilities are endless. Even a small local water treater can afford to own one of these devices, and they are powerful tools for monitoring and surveying.
Fourier Transform Infrared-Attenuated Total Reflectance This is a somewhat exotic technique combining two different processes and is applicable to the study of surface films, such as gamma-iron oxide versus magnetite; cupric oxide; organic surfactants; or deposited films such as iron phosphate, calcium phosphate, or calcium carbonate.
Infrared
Chemical bonds (not ionic bonds, however) vibrate at characteristic frequencies that typically lie in the infrared portion of the electromagnetic spectrum (300 Gigahertz [GHz] to 400 Terahertz [THz]), and so can absorb that radiation. If a beam of infrared radiation is passed through a sample, some of that radiation will be absorbed at characteristic frequencies (equivalent to wavelengths, λ, and wavenumbers, cm-1). The resulting spectra can be interpreted as arising from certain characteristic bonds such as C-H, N-H, C-N, C=C, C=O, C=N, etc.
Fourier Transform
A complex waveform can be decomposed to a superposition of sine waves of varying amplitude, frequency, and phase. Figure 5 shows an example of this superposition. The Fourier transform transforms the signal from the time domain (x-axis on the left) to the frequency domain (x-axis on the right). An associated transform transforms the signal from the time domain to the phase domain (not shown below).
Using this technique, a pulsed signal can be repeatedly passed through a sample to yield an output that is summed. The signal-to-noise ratio increases with the square root of the number of scans so that after 100 scans,
Figure 5: Superposition of two different sine waves (left) and the resulting Fourier Transform (right).
the signal-to-noise ratio is 10 times greater than for a single scan (1000.5 = 10); after 100,000 scans, the signalto-noise ratio is 100,0000.5 = 316. The summed signal, which is in the time domain, is then decomposed to the frequency domain using the Fourier transform mathematics. Pulses are typically less than one second so that thousands of spectra can be accumulated in a short period of time. Very weak single scan spectra can thus be amplified to very strong spectra. Hence, the spectra of very thin films of some substances can be obtained at good signalto-noise using this technique.
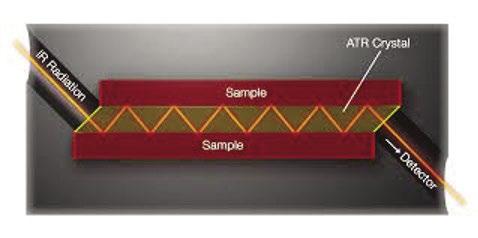
Attenuated Total Reflectance In this technique, a special crystal plate is clamped tightly to a surface, for example a corrosion coupon, The crystal transmits infrared radiation with minimal attenuation. The geometry of the crystal allows multiple passes of the beam through the sample. Each internal reflection leads to partial absorption of the infrared signal by the surface film on the sample. The penetration of the infrared beam into the sample is small so that only surface films are sampled, not bulk material.
Figure 6: ATR crystal between two metal plates. The geometry creates 12 internal reflections.
IR Radiation Sample
Sample ATR Crystal
Detector
In Figure 6, the angle of incidence (normal to the beveled edge of the ATR crystal) and the crystal thickness and length produces 6 beam absorptions per side for each Infrared pulse. So, after 100,000 pulses the signalto-noise is equal to 1,200,0000.5 = 1,095. However, even more importantly, only the film at the surface is being analyzed to the exclusion of other contaminants.
Consider trying to investigate the behavior of a tolyltriazole film on copper under various water chemistries. The FTIR-ATR method is ideal for this type of study. And, when combined with EIS studies, a detailed picture of the inhibition of copper corrosion by tolyltriazole can be elucidated.
Summary 2.2.8 Prohibited Organization. Any Water Treatment These techniques are not commonly considered by Company (WTC) that has, in the aggregate, more typical water treaters and contribute little to ordinary than one hundred fifty (150) full-time employees in the industrial water treatment business. But increasingly, water treatment division or annual gross revenues from some industrial water applications require very sophistia primary water treatment operation that is greater than cated monitoring and investigational techniques. This is $30M US. Sustaining Members are exempt from this especially true of microelectronics, medical devices, and provision. medical appliance production industries. Also, hospitals and clinics can require the application of sophisticated Rationale techniques for critical systems. AWT small business owners would gain access to a wider network both domestically and abroad. New Even if the water treatment company does not possess participants and their participation would enrich the the means or the technical capability to utilize these value of the AWT community. There are many talented techniques, it should be aware of the various investi-individuals who could contribute to the talent pool, gational techniques because they can be subbed out and thereby increase our collective intellectual capital. to various commercial laboratory suppliers if the need Individual membership would help AWT gain a greater arises. After all, the primary responsibility of a water international footprint. treatment provider is to supply whatever expertise may be needed to support the service program at a given This category would be restricted from: end-user. 1. Voting in AWT elections. 2. Sitting on the board of directors 3. Chairing any AWT committee 4. Participating in all “business owner only” functions, including the business resources section of the website. They would get: 1. Access to member educational resources 2. Member pricing for all events 3. Ability to participate in committees, but not chair 4. Greater connection to our community, but not any decision-making control To view all FAQs on these ballot measures as well as learn more about the voting process, please visit https:// www.awt.org/members-section/amplify-awt.
Uniphos 2020.pdf 1 4/23/2020 3:57:49 PM Orin Hollander, Ph.D., has a B.A. in chemistry from Northwestern University and a doctorate in chemistry from The Ohio State University. He worked for 16 years in the water treatment industry and for 26 years as president of Holland Technologies, a consulting and engineering firm serving water treatment companies and end-users.
Uniphos
C
M
Y
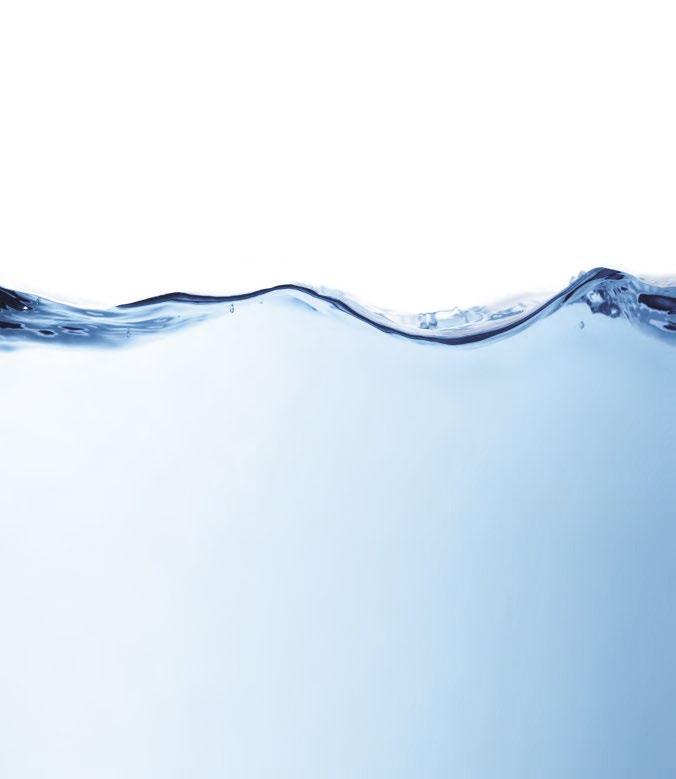
CM
MY
CY
CMY
K A US-based company helping North American water treatment chemical companies, blenders, and distributors. Our technical representatives have more than 25 years experience in water treatment chemicals.
Uniphos, Inc.
1011 West Lake Street Suite 210 Oak Park, IL 60301
Phone: 708 445-1294 • Fax: 708 445-1394 • Email: gcollias.uniphos@sbcglobal.net