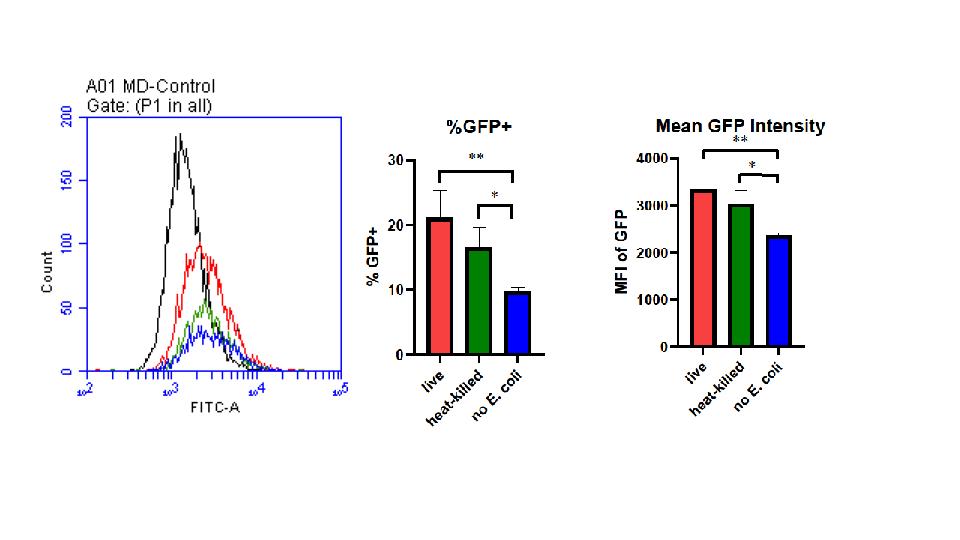
6 minute read
Alisiara Hobbs, Caroline Feig, and Lorena Ramirez
ALISIARA HOBBS CAROLINE FEIG LORENA RAMIREZ
Macrophages
Introduction
Phagocytosis, a type of endocytosis, is an engulfing process done by phagocytic cells to eliminate invading organisms. An example of a phagocytic cell undergoing this process is a macrophage. A macrophage is a type of white blood cell that identifies, engulfs, and eliminates harmful bacteria, in this case E. coli. Unlike other methods of transportation, such as osmosis and active transport, the particles involved in phagocytosis are too large to be carried across the membrane causing the cell to attach and engulf the particle (Richards, 2017). A method of quantifying the rate of E. coli being phagocytized is using flow cytometry. Flow cytometry is a technique used to measure particular components of given cells (McKinnon, 2018). A sample of cells, suspended in a fluid, is injected into a flow cytometer where they pass through very small channels that align them in a single file as they pass through the detector. In order for E. coli to be read by the machine, it needs to be changed to express glowing fluorescence done in a process called transformation. Transformation occurs when foreign DNA is added to bacteria, ultimately resulting in the uptake and expression of the traits in the DNA (Griffiths, Miller, Suzuki 2013). Plasmids, pieces of DNA encoding for specific traits, are used in the process of transformation. GFP plasmids are proteins that encode for a green fluorescent gene and can be transformed
into the bacteria, allowing E. coli to uptake and express a green fluorescence. During transformation, bacteria can acquire multiple genes from the plasmid. In addition to receiving the GFP from the plasmid, E. coli will receive a gene coding for antibiotic resistance, in this case ampicillin resistance or ampR. Positive ampR cells contain the resistance to ampicillin and can be used to distinguish those who successfully transformed the GFP gene (Feilmeier, 2020). This can be done by suspending the bacteria onto an antibiotic plate after transformation, in this case ampicillin agar plate, where those without this resistance will not be able to survive. Being specialized in the phagocytic process, a macrophage may have many reasons to want to undergo this process. E. coli, typically able to coexist in the human intestine, may exist as a strain that is deemed a harmful bacterium, ultimately turning it into a candidate for phagocytosis. Primarily, macrophages recognize harmful particles that are actively harming the body such as live E. coli, but this may not always be the case. In humans, abundant cell types often exhibit short life cycles, resulting in about 100 billion dead cells within a 24-hour period (Uribe-Querol & Rosales, 2017). Having such a large number of built-up cells requires a method or recognizing and removing the inactive cells. Inactive cells, such as heat-killed E. coli, will be recognized and removed by the macrophage also via phagocytosis. Although both reasons for phagocytosis are equally important, we were curious to see if there was a difference in rate of phagocytosis between alive and inactive bacteria. Comparing the rates of phagocytosis using flow cytometry, of live and heat-killed E. coli, we expect the rate to be higher for the alive bacteria.
Materials and Methods
GFP E. coli:
To begin setting up for this experiment, E. coli was transformed from a green fluorescent protein (GFP) into E. coli where it was then treated for antibiotic resistance. First, E. coli was taken from a plate during the log phase and transferred into a cold Calcium Chloride Solution, using a bacterial loop. Next ampR plasmids were added to the bacterial-chloride solution and were shocked with heat. Using another bacterial loop, the cells were streaked onto an ampicillin agar plate. Cells resistant to the antibiotic survived and were incubated for 24 hours resulting in isolated ampR E. coli.
Phagocytosis:
Isolated ampR ampicillin resistant positive E. coli cells were taken and suspended into a liquid. The number of bacteria was quantified using a spectrometer machine. The OD 600 from the machine was then compared to the ratio of 2.69x 109/ 1 OD 600 to determine the number of cells in the liquid. RAW264.7 Macrophages were observed under a microscope using a hemocytometer and Trypan blue to determine the number of cells present. A ratio of macrophages to E. coli was created (1:5) and was co-cultured with the GFP-E. coli under our two conditions. Using two six-well plates, a negative control, heat-killed, and a positive controlled E. coli was placed in each well with each having three replicates. In each well, 1 mL of macrophages containing 1x10^(6 )cells were added with 1.04 µl of E. coli. First, in three wells, live E. coli was placed with macrophages to create the negative control. Next, in six wells, E. coli was heat-killed using a microwave for 10 seconds. Lastly, in three wells, macrophages were placed with no bacteria creating the positive control.
Flow cytometry:
Once the well plates were set up, they were run through a flow cytometry machine where green cells were analyzed, giving us data on which type of E. coli is most readily phagocytized by macrophages.
Results
Figure 1
(A) Status of E. coli does not affect the rate of phagocytosis. (A) Distribution of cells expressing GFP+ in relation to its intensity. (B)
Percentage of E. coli expressing GFP+ gene within varying conditions. *P=0.0287 **P=0.0036 determined by one-way ANOVA for multiple comparisons. (C) Mean Fluorescence Intensity (MFI) of GFP in varying conditions *P=0.0268 **P=0.0078 determined by one-way ANOVA for multiple comparisons. 1.04 µL of heat-killed and live E. coli were co-cultured with 1mL of RAW264.7 macrophages containing 1x106 cells. Comparisons of heatkilled, live, and E. coli-absent cultures were completed using a flow cytometry, each replicated 6 times.
All conditions of E. coli were effectively phagocytized by macrophages with no significant difference between the two; however, there were significant differences between the live E. coli and the control. Displaying nearly double the number of cells within the distribution of cell intensity, live e. coli exhibited the most abundant cells within 10^3 and 10^4 intensity (A). Heat-killed E. coli also typically displayed higher intensities than the control in terms of area under the curve but was not significantly different. In relation to the GFP intensity, heat-killed E. coli had no significant difference compared to live but did in relation the control of no e. coli (B).
Discussion
Previous studies have shown that phagocytosis of macrophages predominantly attack detrimental E. coli when present in the human intestine. However, macrophages are known to phagocytize inactive bacteria as well (Uribe-Querol & Rosales, 2017). Herein, we identified the effects of phagocytosis of macrophages in live and heat-killed conditions of E. coli. and expected the rate of active bacteria to be higher than inactive bacteria. Our flowcytometry revealed there was no significant difference amongst the live and heat-killed bacteria. Additionally, we demonstrated a notable difference between live E. coli and the control, resulting in a pvalue of 0.0036. Coevality, the difference between heat-killed and the control was significant in relation to the GFP intensity, resulting in a pvalue of 0.0287. Based on these findings, we can conclude that in the terms of phagocytosis, macrophages prioritize live and heat-killed E. coli. equally. To complete the full understanding of this experiment, future experiments should also work on different types of noxious bacteria to discover if it has the same effect.
Feilmeier, B. J., Impinger, G., Schroeder, D., Webber, H., & Phillips, G. J. (2020, December 20). Green Fluorescent Protein Functions as a Reporter for Protein Localization in Escherichia coli. Journal for Bacteriology. https://doi.org/10.1128/JB.182.14.4068-4076.2000
Griffiths, A., Miller, J., & Suzuki, D. (2000). An introduction to genetic analysis (7th ed.). W. H. Freeman. https://www.ncbi.nlm.nih.gov/books/NBK21993/
McKinnon, K. M. (2018, Feb 21). Flow cytometry: An overview. Current Protocols in Immunology, 120. https://www.ncbi.nlm.nih.gov/pmc/articles/PMC5939936/
Richards, D. M. (2017, October 26). How cells engulf: A review of theoretical approaches to phagocytosis. IOPScience. https://pubmed.ncbi.nlm.nih.gov/28824015/
Uribe-Querol, E., & Rosales, C. (2017). Control of phagocytosis by microbial pathogens. Frontiers in Immunology, 8, 1368. https://doi.org/10.3389/fimmu.2017.01368