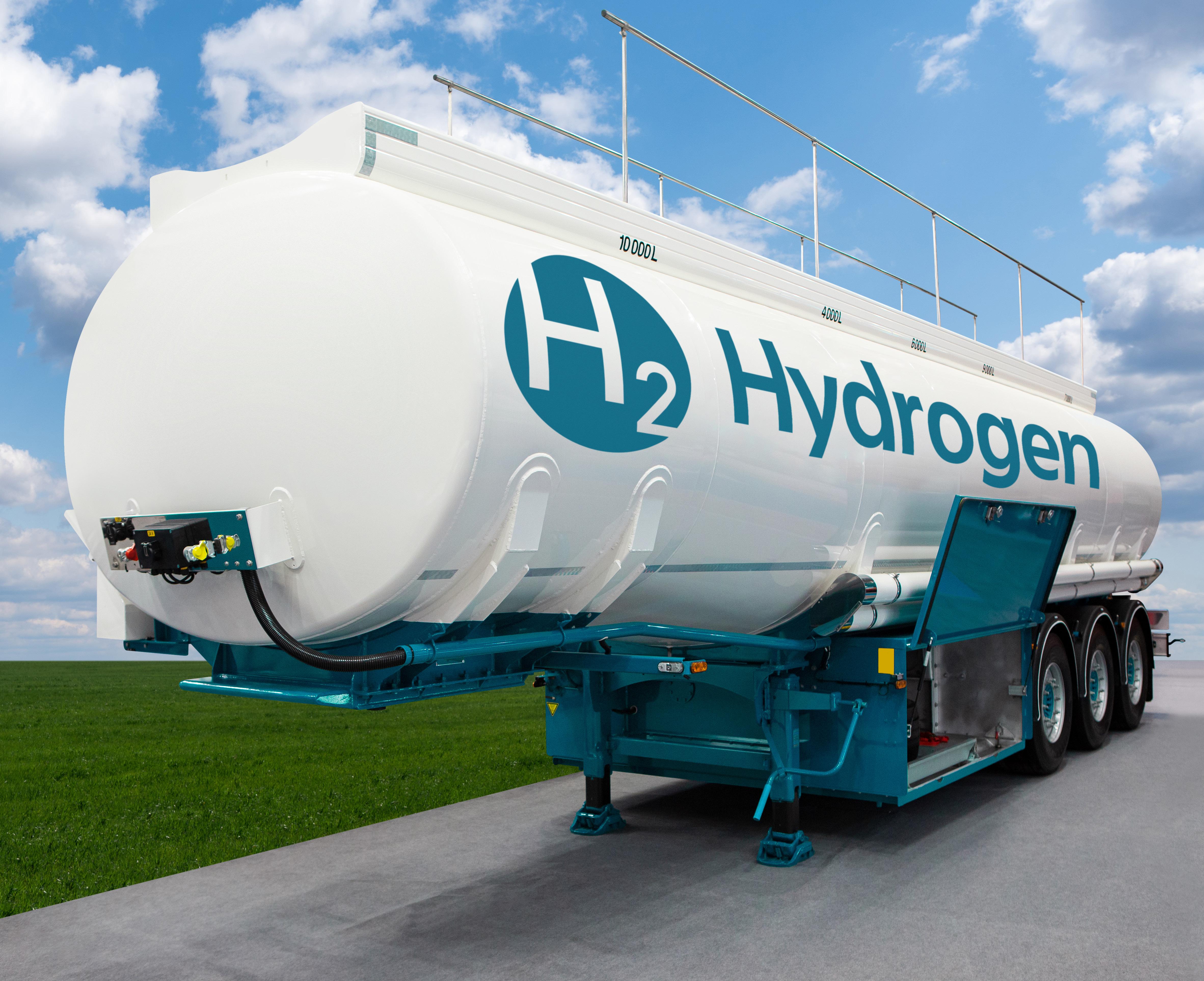
10 minute read
Hydrogen from biomass: challenges and perspectives
Marco Buffi, Matteo Prussi, Nicolae Scarlat, JRC, Directorate C - Energy, Transport and Climate Energy Efficiency and Renewables
Advertisement
Hydrogen is one of the main pillars of the Europe’s decarbonization strategy for the next years, offering a clean solution for mobility, power generation and industry. There are several available sustainable production options and there is still uncertainty about which is the most effective pathway for hydrogen. The larger part of the current production is mainly divided in three categories, which are commonly referred to as grey, blue and green hydrogen. While the first two classifications derive from natural gas (varying from the presence or not of carbon capture and storage strategies), the third one is produced from renewables energies, excluding biomass. A fourth type of hydrogen can be produced from the gasification of coal and is referred to as brown or black hydrogen, depending on the grade of coal being used. The “colours” of hydrogen (associated to the primary feedstock adopted for its production) are not sufficient to define its sustainability, and each production route has to be properly addressed according to
materials and energy requirements, environmental impact and the current technology readiness level (TRL). We could therefore consider another category, consisting in the hydrogen from biomass, which has currently no colours associated with; this is generally also called “bio-hydrogen”. However, this name is often associated only to the hydrogen produced from steam biomethane reforming, but among the various biomass to hydrogen pathways there are also gasification, biologic processes and others, thus this category will be simply named “hydrogen from biomass”.
THE EU HYDROGEN STRATEGY
From a legislative point of view, when renewable hydrogen is used as energy vector in the European context, the producers have to comply with the existing provisions, as defined in the recast Renewable Energy Directive (RED II) and its associated delegated acts. By analogy with other alternative fuels, all the imports of hydrogen will also have to result aligned with European provisions, regardless of other standards. In order to boost the sector, the European Commission on 8 July 2020 adopted a new hydrogen strategy with the communication ‘A hydrogen strategy for a climateneutral Europe’ [1]. This strategy is part of the European Green Deal [2] for the climateneutrality by 2050, which sets concrete targets for GHGs emissions reduction. The main pillar of this strategy is the promotion of green hydrogen production and the expected targets are the installation of at least 6 GW of electrolysers powered by renewables in the EU by 2024, and 40 GW by 2030. In addition, the “Next Generation EU” recovery fund of EUR 750 billion [3] will adopt over one third of this finance for this scope, thus it will be expected an immediate market uptake of the current production technologies. It is worth noticing that electrolysers should be powered by renewable electricity that require large, efficient and clean infrastructures, and hence major investments, and a particular attention to GHGs emissions associated to its production and distribution. In addition, a stable hydrogen supply should not be only dependent by those energy sources notcontinuously available such as solar and wind, but it should be integrated with stable production pathways that today are still powered by fossil fuels.
SUSTAINABILITY ISSUES
Regarding sustainability aspects, according to RED II the so-called “green hydrogen” is defined as “renewable fuel of non-biological origin” and must meet the 70% thresholds for minimum GHG emission savings. Therefore, a low-carbon hydrogen definition, tracking and tracing mechanisms are required to demonstrate compliance with the above-mentioned targets within a Delegated Act (due to 2021) for assessing greenhouse gas emissions savings from renewable liquid and gaseous transport fuels of non-biological origin and from recycled carbon fuels. Here the additionality concept applied to the environmental assessment has to be defined: it implies that the reduction in the GHG emissions happen only as a result of the new project, or rather new renewable electricity plants built for the purpose of hydrogen production. Both green hydrogen and hydrogen from biomass are part of the 14% target of RED II as minimum share of renewables in the overall energy consumption of the transport sector, but the only hydrogen produced from biomass sources contributes to the overall renewable energy target. In the case hydrogen is produced with an initial feedstock listed in Part A of Annex IX, it can be considered as advanced biofuel, subject to the double counting. Therefore, hydrogen from biomass sources is a ready alternative to integrate the production of renewable hydrogen in the coming years.
BIOMASS-TO-HYDROGEN CONVERSION PATHWAYS
In this phase, it becomes of primary importance to address technoenvironmental issues related to biomass conversion, since hydrogen is not directly available in its pure form but bound into the biomass chemical structure. High energy expenditure is needed to separate it from the other atoms, and complex conversion steps have to be considered in the whole supply chain. Hence, the environmental and energy performances of hydrogen energy systems strongly depend on the hydrogen donor and the energy sources needed by the conversion process. As first division, biomass-tohydrogen processes can be distinguished in two different categories: • thermochemical pathways including pyrolysis, liquefaction, and gasification; • biological pathways including water–gas shift reactions promoted by micro-organisms, photo-fermentation and darkfermentation, anaerobic digestion and biomethane upgrading, and bio-photolysis such as microbial electrolysis cell. Among these pathways, only few of them can be potentially integrated into an existing value chain fully dedicated to the commercial production. For instance, anaerobic digestion of agro-residues for biogas production is already used for biomethane production at commercial level, but rarely (or
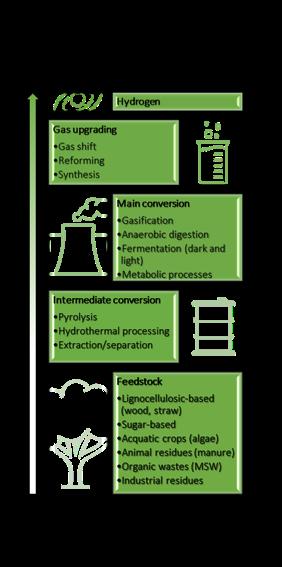
H2
3rd level
2nd level
1st level
Biomass
Figure 1 - Biomass to hydrogen production pathways at different conversion levels.
only at experimental level) biogas is further converted to hydrogen. On the other hand, the steam methane reforming is a full commercial technology largely adopted for the current hydrogen production. Thus, economic and technical barriers still exist, and the absence of a consolidated market is limiting the promotion of new value chains. Except pyrolysis, gasification and anaerobic digestion, all the other conversion pathways are still at low TRL, but creating synergies with other sector (such as the fossil fuel sector), they can largely grow in a short time. For this propose, a potential classification of the whole biomass-to-hydrogen conversion steps can be divided in three different levels as shown in Figure 1. Starting from pure biomass sources or residues, a potential 1st level of conversion consists in those processes aimed to pre-treat or upgrade the feedstock before the main conversion processes (i.e. 2nd level of conversion). By means of these processes, biomass can be converted into bio-intermediates such as fast pyrolysis oil, HTL oils, lignin and/or sugars, that are easier to be further converted to hydrogen gaseous precursors. • First level of conversion is also aimed to densify biomass and make it more similar to fossilderived feedstocks, to be suitable for those processes deriving from coal/oil processing (e.g. entrained flow gasification). • At the second level of conversion there are those processes that
can be fed by both pure biomass and bio-intermediates, but differently than the conventional value chains for bioenergy and biofuel production, they require an additional conversion step to hydrogen production. • Finally, the third level conversion step, which is the gas upgrading section, consists in those thermochemical processes such as methane reforming, gasshift and synthesis for hydrogen separation and purification.
Combining the TRLs of each conversion step to build a hydrogen-based value chain, just two production pathways (i.e. biomass gasification and gas upgrading section; steam biomethane reforming from anaerobic digestion) are currently ready to be used at commercial level, while the others need further assessment before the technology scale up to a potential market uptake.
ENVIRONMENTAL PERFORMANCES
Once the conversion pathways are established, it is of utmost importance to evaluate the energy and environmental performances of each route. For this purpose, this article recaps the findings reported in our upcoming review on biomass-to-hydrogen production pathways [4], focusing on energy and environmental issues. From the studies available in literature on GHGs assessments, we selected five biomass-to-conversion pathways that have been investigated in detail and can be potentially adopted at commercial level (as shows in Figure 2): wood gasification and gas upgrading (GASIF) and biogas production and steam biomethane reforming (SR-BG), are already consolidated pathways for the maturity of the technologies; steam reforming of gasified biointermediates (SR-IT) such as bio-oil or glycerol, are limited for economic
costs; other biological processes (FERM, i.e. bio-hydrogen from dark- or light-fermentation, and MEC, i.e. microbial electrolysis cells) are promising but still at low TRL, and require large infrastructures for very limited productions. The method used to perform the analysis is the life cycle assessment (LCA), that is a well-established methodology for the comprehensive evaluation of the potential environmental impacts of product systems. Among the relatively high number of LCA studies on hydrogen available in the scientific literature, the present analysis selected a number of 35 peer-reviewed papers reporting detailed environmental assessments of biomass to hydrogen routes. Figure 2 shows the averaged values of the calculated carbon footprints (including the lower and the upper figures) per mass unit of hydrogen produced, divided per production pathway. In addition, the carbon footprint (in orange) of steam methane reforming (SMR) of natural gas is reported as benchmark value. It is noteworthy to point out that each averaged value (dark green bars) is within or below the NG SMR line, showing the environmental benefit of using biomass instead of natural gas as initial feedstock. In addition, the lower limits representing the most optimistic studies showed how GHGs emissions can be reduced at very low level, generally considering renewable energy input for biomass processing, carbon credits due to biomass cultivation and optimistic conversion yields. Despite international standardisation of ISO 14040 [5] and the availability of both general and specific LCA guidance documents produced by EC JRC [6], each selected study follows its own methodological choices. This strongly affects the results of calculated GHGs emissions and its final interpretation, leading to a wide range of values for each pathway. Furthermore, the differences in system boundaries, functional units, allocation approach and other technical parameters introduce uncertainties for robust comparison of results from different studies. Harmonization protocols might be applied to reduce some gaps of results, as well as confirm the validity of a branch of methodologies instead of the others here evaluated.
CONCLUSIONS
The scope of this article is to remark how hydrogen produced from biomass already exhibits a good technology maturity with a favourable environmental impact. The upcoming EU strategy promoting the growth of the hydrogen market will make available large amounts of funding for the immediate market uptake of the existing technologies. Beside green hydrogen production, current available technologies for hydrogen production from biomass can be potentially integrated in a biorefinery concept. Limits and barriers related to economic aspects of novel technologies (e.g. fermentation, MEC) need further assessment, and largescale generation is recommended only for thermochemical routes, while biological processes are most appropriate for small scale production due to the simultaneous generation of hydrogen and waste and residues recycling.
The views expressed here are purely those of the authors and may not, under any circumstances, be regarded as an official position of the European Commission.
References available at page 51.
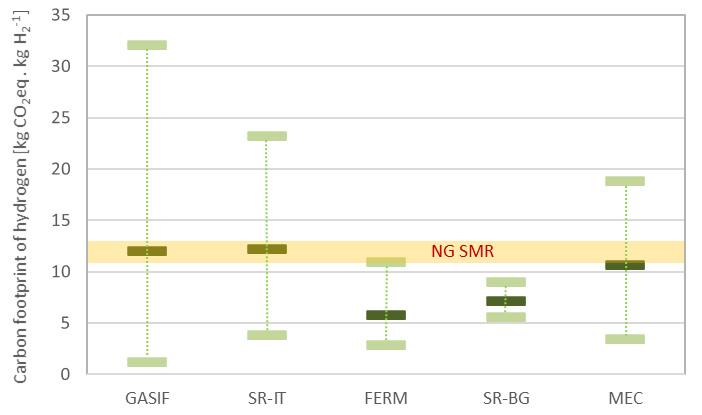
Figure 2 - Carbon footprints of five selected biomass-to-hydrogen production pathways [4]: wood gasification and gas upgrading (GASIF); biogas production and steam biomethane reforming (SR-BG); steam reforming of gasified bio-intermediates (SR-IT); dark- or light-fermentation (FERM); microbial electrolysis cells (MEC). Dark green bars represent the averaged values among the calculated footprints available in literature, while the light green bars are the upper and lower limits among the selected figures. In orange, the range of the calculated carbon footprints of the natural gas steam methane reforming (NG SMR) as benchmark zone.