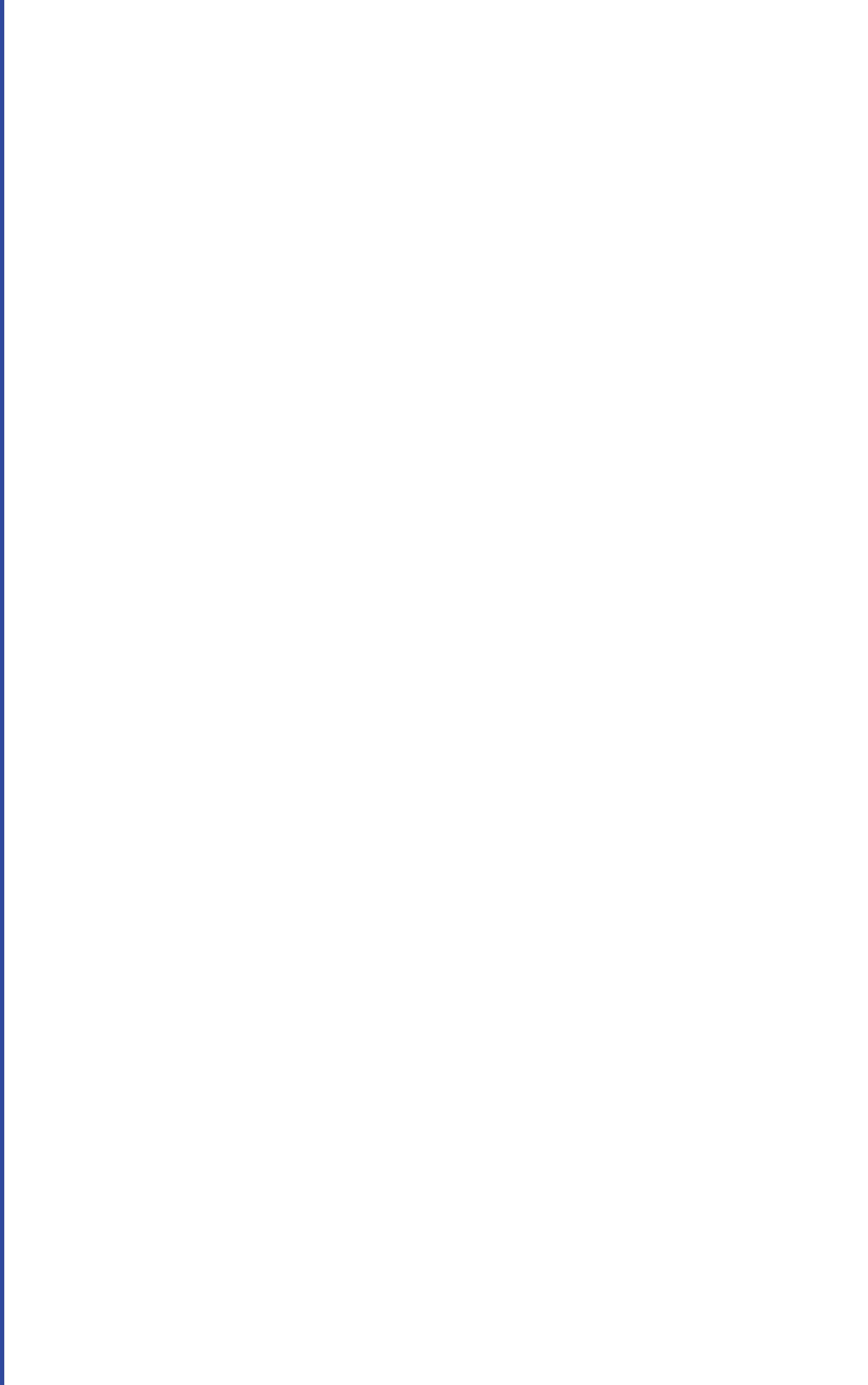
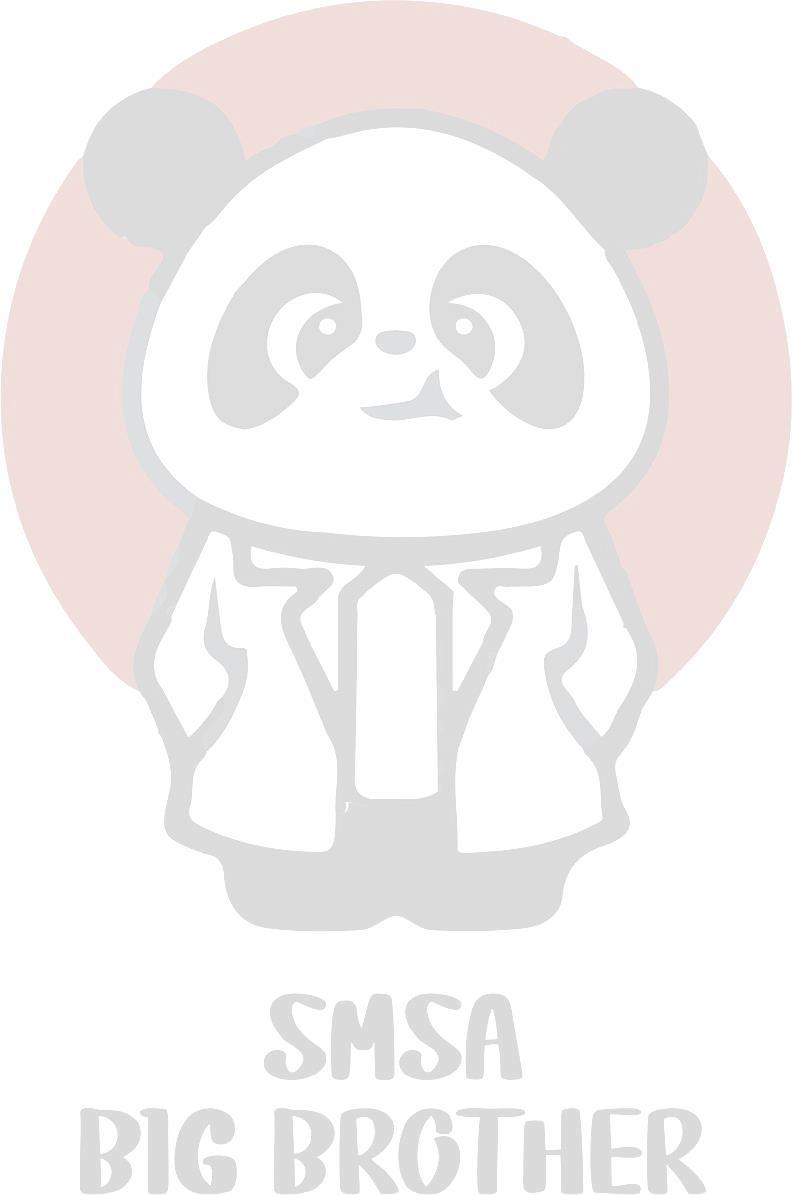
Definition
Proteins are organic compounds with a high molecular weight formed of subunits called amino acids linked together by peptide linkage. The term protein is applied to describe molecules greater than 50 amino acids.
What is the biological importance of proteins ?
1. Nutritional role : Provide the body with essential amino acids, nitrogen and sulfur.
2. Catalytic role : All enzymes are proteins in nature.
3. Hormonal role : Most of hormones and all cellular receptors are protein in nature.
4. Defensive role : The antibodies (immunoglobulins) that play an important role in the body’s defensive mechanisms are proteins in nature.
5. Osmotic pressure : Plasma proteins are responsible for most effective osmotic pressure of the blood. This osmotic pressure plays a central role in many processes, e.g., urine formation. Thus help in maintenance of electrolytes and water balance in the body.
6. Transport role : Proteins carry lipids in the blood forming lipoprotein complexes. Proteins also carry, hormones, e.g., thyroid hormones and minerals, e.g., calcium, iron and copper. Hemoglobin (a chromo-protein) carries O2 from the lung to tissues is a protein.
7. Structural role : Proteins are the main structural component in bone, muscles, cyto-skeleton and cell membrane.
8. Blood clotting : coagulation factors are proteins.
9. Control of gene expression : Most factors required for DNA replication, transcription and mRNA translation are protein in nature.
Amino acids are organic acids that contain NH2 group. They are the structural units of proteins and are obtained from them by hydrolysis. The general formula of any amino acid is as follows:
Although about 300 amino acids exist in nature, only 20 of them can polymerize in protein structure. For each a specific codon exists in the genetic code. All these amino acids are alpha-amino acids. This means that the amino group is attached to the -carbon atom (next to the carboxyl group).
All amino acids present in mammals are L-amino acids (amino group is on the left side configuration). Whereas D-amino acids are found in the cell walls of bacteria.
Each amino acid (except proline and hydroxyproline) has a carboxyl group (COOH), an amino group (NH2) and a characteristic side chain (R).
In -amino acids, both-COOH and –NH2 groups are attached to the same (-) carbon atom. This why they are amphoteric, i.e., react as acid (by COOH) and as alkali (by NH2).
At physiological PH (approximately PH 7.4) the carboxyl group is dessociated forming a negatively charged carboxylate ion (-COO- ) and the amino group is protonated forming positively charged ion (-NH3 + ).
All amino acids (except glycine) are optically active, i.e., can rotate plane polarized light. This is because the 4 groups attached to -carbon are different. In glycine, the -carbon is attached to 2 hydrogen atoms, therefore, is optically inactive.
Amino acids can be classified by one of three methods:
Chemical classification: Biological classification: Metabolic Classification:
Based upon the number of amino groups or carboxyl groups in the amino acid:
Neutral amino acids (mono-amino, monocarboxylic).
Based upon whether the amino acids can be synthesized in human body or not:
Based upon the fate of amino acid inside the body:
Acidic amino acids (mono-amino, dicarboxylic).
Indispensable or essential amino acids: Not synthesized in the body and must be supplied in the diet.
Glucogenic amino acids, that can be converted to glucose.
Ketogenic amino acids, that can be converted to ketone bodies.
Basic amino acids (diamino, monocarboxylic)
Dispensable or nonessential amino acids: Can be synthesized in the body and hence is not essential to be present in diet.
Mixed amino acids, i.e., can be converted to both glucose and ketone bodies.
(According to number of carboxyl and amino groups)
Amino acids can be classified into:
a) Neutral amino acids.
b) Acidic amino acids. c) Basic amino acids.
They contain one amino group and one carboxyl group. They have 5 types:
1. Aliphatic amino acids
2. Hydroxy amino acids: contain –OH group in their side chain e.g., serine, threonine, tyrosine, hydroxyproline and hydroxy-lysine.
Serine gives choline for phospholipid and acetylcholine synthesis and the OH groups of threonine and serine are site for protein glycosylation and phosphorylation.
3. Aromatic amino acids: e.g., phenylalanine, tyrosine and tryptophan.
Tyrosine is synthesized from phenyl alanine and both give triiodothyronine and thyroxin, adrenaline and noradrenaline, melanin pigment and cresol and phenol in the body.
4. Sulfur-containing amino acids: e.g., - Cysteine gives cystine and its SH group is very essential in activity of many proteins particularly the active sites of enzymes. It is also important for intra- and interpolypeptide disulfide bond formation that stabilizes protein structure. Sadenosylmethionine is active form of methionine and is the main methyl donor.
e.g., - Histidine is basic in solution on account of the imidazole ring and often considered as basic amino acids.
Histidine gives histamine a very important inflammatory mediator. Tryptophan is often considered as aromatic amino acids since it has aromatic ring in its structure. Tryptophan gives nicotinic acid, melatonin, serotonin and indican in the body.
Proline and hydroxyproline do not have a free -NH2 group but only a basic pyrrolidone ring in which the nitrogen of the imino group is in a ring but can still function in the formation of peptides. Therefore, they are called as imino acids.Proline gives hydroxyproline that is essential for collagen cross-linking.
They contain 2 carboxyl groups and one amino group, e.g., glutamic acid and asparatic acid.
These acidic amino acids can occur in the tissue in the form of amides, e.g., glutamic acid glutamine and asparatic acid asparagine.
Glutamine has a major role in removing ammonia from the brain and glutamic acid is a neurotransmitter and essential for urea and glutathione synthesis.
Asparagine is important for protein cross-linking and glycosylation.
They contain 2 amino groups and one carboxyl group, e.g., Ornithine and Arginine. Ornithine does not enter in the synthesis of proteins and is usually present in the free form. It is synthesized from arginine. Both are participate in urea synthesis from ammonia.
Arginine is used in synthesis of nitric oxide, a hormonal second messenger. Ornithine is used for synthesis of polyamines that are important for cell cycle control.
Citrulline is formed from ornithine during urea synthesis but does not participate in protein structure.
Lysine and Hydroxy lysine: They participate in protein cross-linking.
Amino acids may be classified into:
a) glucogenic amino acids, i.e., those which can be converted into glucose, b) ketogenic amino acids, i.e., those which can be converted into ketone bodies c) mixed amino acids, i.e., those which can be converted into both glucose and ketone bodies.
C. Biological or Nutritional Classification:
Some amino acids can not be synthesized inside the body. If these amino acids are not taken in diet they will affect the growth and the health.
Thus, amino acids may be classified into:
A- Essential amino acids: (Indispensable amino acids):
These are amino acids that can not be synthesized in the human body and should be taken in the diet, otherwise their deficiency will lead to a nutrition deficiency disease that affect both growth and health.
Arginine and histidine are semi-essential, i.e., they are mainly required in growing children, pregnant and lactating women and convalescent patients.
The main source for these amino acids are animal proteins (milk, egg, meat, liver, fish, chicken) and a few plant proteins (bean and lintels). They are as follows (VITTAL LyMPH):
B- Non essential amino acids: (Dispensable amino acids):
The rest of amino acids can be synthesized inside the human body and their deficiency in diet does not affect the growth or the health.
Peptides are compounds, formed of less than 50 amino acids linked together by peptide bonds.
1- Dipeptide (2 amino acids and one peptide bond).
2- Tripeptide (3 amino acids and 2 peptide bonds).
3- Oligopeptide (3-10 amino acids).
4- Polypeptide (10-50 amino acids).
Examples for physiologically active short peptides: Glutathione (glutamyl, cysteinyl, Glycine):
It is a tripeptide formed of 3 amino acids: glutamic, cysteine and glycine.
Functions of glutathione:
1. It has a role in absorption of amino acids.
2. It activates many enzymes.
3. It inactivates insulin hormone, by breaking its disulfide bonds.
4. It protects the cell membrane from damage, e.g., prevents hemolysis of erythrocytes.
5. It prevents rancidity of fat (or lipid peroxidation) as it acts as antioxidant, 2 GSH GSSG + 2 H
There are 4 levels or orders of organization of the structure protein molecule: primary, secondary, tertiary and quaternary structures. This complication gives the molecule its functional domain to explain its structure-function requirements that if changes due to mutation will give nonfunctional protein and, therefore, a disease.
Primary structure is the number, type and linear sequence of amino acids held together by peptide bonds in its peptide chain. The peptide bonds (primary bond) are responsible for the primary structure.
It is dedicated by the genetic code in specific gene and it determines the final conformational shape of a polypeptide. The free -NH2 group of the terminal amino acid is called as N terminal end and the free –COOH is called as C-terminal end.
Substitution of a single amino acid for another in the linear sequence of amino acids in the polypeptide chain, may reduce or abolish biologic activity of the protein with potentially serious consequences. An example is Sickle Cell Anemia where the normal glutamic acid at position 6 of the chains is replaced by a valine.
It is the fine folding of polypeptide chain into specific regular coiled structure. It is due to the interaction of amino acids located very close to each other. They are held together by hydrogen, ionic and disulfide bonds.
If the hydrogen bonds form between peptide bonds in the same chain, helical structures develop such as the -helix.
If the hydrogen bonds form between the peptide bonds in different chains, extended structures form such as -pleated sheet. This gives the polypeptide chain a specific space conformation.
The -helix:
It is a rod-like structure with the peptide bonds coild tightly inside and the side chains of the residues (R) extending outward from the chain.
Characterstics :
1. Each (C=0) of one amino acid is hydrogen bonded to the (-NH) of the next fourth amino acid in the chain (1 4) (intrachain hydrogen bonding).
2. The complete turn distance equals 54 nm.
3. Each turn contains 3.6 amino acids residues.
Examples :
The proteins of hair, nail and skin (skin contains a group of proteins called keratins rich in -helical structure.
-Pleated sheets
This structure is formed between two or more separate polypeptide chains. It may also be formed between segments of the same polypeptide chain (intrachain).
Hydrogen bond is also responsible for its formation. It occurs between (-NH) group of one chain (or segment) and (C=0) group of adjacent chain (or segment).
Two types of -sheets are present:
1. Parallel -sheets: in which the two polypeptide chains run in the same direction.
2. Anti.parallel -sheets: in which the two polypeptide chains run in opposite direction.
Examples: -keratins present in spider web and silk fibers.
It is the final three-dimensional form due to the more complicated course folding and super-folding of the polypeptide chain in its secondary level into globular or fibrous form of different size.It is due to interaction of amino acids located far apart.
It is the biologically active conformation of the polypeptide and the protein in this conformation is called native protein. Therefore, it is the most liable to denaturation.
The bonds stabilizing the tertiary structure are disulfide bonds, hydrogen bonds, ionic bonds, van-der-Waal’s attraction (occur between nonpolar side chains) and hydrophobic interaction (all are secondary bonds). According to it the protein is classified as :
fibrous (extended form e.g. keratin, collagen and elastin) or
globular (impact form and results from folding of polypeptide chain e.g. myoglobulin )
Proteins consist of two or more polypeptide chains (subunits) in their tertiarystructure united by forces other than peptide bonds are said to possess a quaternary structure.
Quaternary structure therefore, is the positional relationship between individual polypeptides associating to form one protein molecule. If there are two subunits the protein is called dimeric, three subunits it is called trimeric and if formed . of several subunits, so it is called multimeric The bonds responsible for the quaternary structure are as those in the tertiary structure. It is the most liable to denaturation . Examples are : globin of hemoglobin and lactate dehydrogenase each is composed of 4-polypeptide chains. Apoferritin is composed of 24 identical subunits. Creatine kinase . is composed of two subunits
Finally, different proteins – each of these proteins alone has all 4 orders of structure – ,aggregate into macro-molecular complexes as exemplified by cell membrane proteins . electron transport proteins and fatty acid synthase enzyme.
✔ Different classifications of protein. ✔ Types of proteins according to its classification.
I.The biological importance of the protein II.The axial ratio of the protein molecule
Proteins are classified according to 1. Proteins of high biological value E1 2. Proteins of low biological value E2 2. Globular proteins
III.The composition of the Protein
I. According to the biological importance of the protein:
Proteins of high biological value E1 :
These are all proteins of animal origin (with a few exceptions) and some proteins of plant origin , that contain all the 10 essential amino acids in well balanced amounts and are easily digestible
1. Fibrous proteins C) Derived Proteins
B) Conjugated proteins
proteins B. Secondary derived proteins
Examples of animal proteins include; milks and its products, egg, liver, fishes, red and white meats. Examples of the few plant proteins of high biological value are lentils and broad beans
These are proteins that is deficient in one or more of the essential amino acids or containing very little amount of one of them or are indigestible.
Most of plant protein are of low biological value and a very few animal proteins are also of low biological value such are collagen because is deficient in tryptophan and cysteine and keratins because they are indigestible. This does not imply that a person should eat only a protein of high biological value to avoid deficiency of essential amino acids, but this can be also avoided by eating two or more proteins of low biological value that complete each other’s deficiency.
- Studies on the shape of the protein molecule using ultramicroscope indicates that there are two types of proteins in nature:
1. Fibrous proteins.
They have an axial ratio of more than 10. ( Axial ratio = Length/Width of the protein molecule. )
They are fairly stable proteins in which the straight polypeptide chains lie parallel (or antiparallel) to one another along a single axsis forming fibers or sheets.
They are usually insoluble and non motile
Examples :-
a. Keratin proteins in hairs, wool and skin. In its native state, it is present in the form of coiled polypeptide chains called -keratin. It can be stretched by denaturation forming -keratin.
b. Myosin is the major protein of muscles. During muscle relaxation it is called myosin but during muscle contraction, it undergoes a change in its structure and it becomes -myosin.
2. Globular proteins.
Their axial ratio is less than 10.
Their one or more peptide chains are folded or coiled on themselves in a very compact manner.
They are less stable than fibrous proteins and they are usually soluble and motile
Examples are albumin, globulins, and insulin.
III. According to the composition of the Protein: -
There are 3 main groups (on the basis of their solubility and physical properties):
A) Simple proteins. B) Conjugated proteins. C) Derived proteins.
- These are proteins which on hydrolysis produce amino acids only. Simple proteins are subdivided according to their physical properties, solubility, molecular weight and amino acid composition into:
Albumins are much distributed in nature. They are usually associated with globulins.
Albumins are characterized by giving positive heat coagulation test. Coagulation happens at about 75oC. Slight acidity accelerates the coagulation, while alkalinity retards it. The heat coagulation test is one of the most important investigations done for the detection of albumin in urine in kidney problems.
Albumins are readily soluble in distilled water and also soluble in very dilute salt solutions, alkalis and acids.
They are not precipitated by half saturation with magnesium sulfate nor sodium chloride, but precipitated by full-saturation with ammonium sulfate.
Albumins are proteins of high biological value since it is not deficient in any of the essential amino acids but deficient in glycine.
They give all the color tests of proteins. They are acidic proteins at pH 7.4 and have low isoelectric pH (4.7).
Examples: Serum albumin present in blood, lactalbumin of milk, ovalbumin of egg, leucosin of cereals and legumelin of legumes such as peas. Pepsin is probably an albumin.
Globulins are always present associated with albumins and are also positive with coagulation test
Euglobulins or true globulins are soluble in saline and insoluble in distilled water. Pseudoglobulins (false globulin) are soluble in distilled water.
Like albumins, they are soluble in dilute acids and alkalis.
Globulins are of high biological value and it is not deficient in any of the essential amino acids.
Globulin is precipitated by half saturation with ammonium sulfate or full saturation with NaCl or MgSO4. This is due to the fact that globulins are bigger in molecular weight than albumins.
The most important members of globulins are serum globulins. By electrophoresis blood globulin was found to be of three , B and types, which possess different molecular weights.
Examples: Myosin of muscle (myosin forms the chief protein of the muscle and forms about 50% of the total proteins of the muscles), thyroglobulin of the thyroid gland, antibodies of the blood, phosphatase enzyme, urease, trypsin, ceruloplasmin, transferrin, chymotrypsin and legumin of peas.
- Soluble in water and salt solution
- Coagulated by heat
-They are of high biological value.
- Contain all essential amino acids.
- Easily digested.
- M.W.: 68 KDa.
-Precipitated by full saturation with ammonium sulfate
-They are present in: serum albumin, egg albumin, milk: lactalbumin
- It functions as transporting protein for elements, vitamins, and hormones other than keeping blood osmosis.
- Soluble in salt solution.
- The same as albumin
-The same as albumin
-The same as albumin
-The same as albumin
- M.W.: 150 KDa
-Precipitated by half saturation with ammonium sulfate
-They are present in: serum globulin, milk Lactglobulin, egg globulin.
- It functions in transport also but its major function is being antibodies.
In blood and biological fluids, globulin is present in solution due to the presence of salts. If blood serum (or egg white solution) is much diluted with distilled water partial precipitation of globulin happens. This can be considered as a rough method for separation of albumin from globulin.
Another method used for separation is to dialyze the solution containing mixture of albumin and globulin against water, as the salt dialyses out, the globulin precipitates. Thus, globulin can be easily separated from albumin.
Protamines are the simplest natural proteins. Their molecule is small and contains no more than 20 amino acid residue with pI of 7.4.
The molecular weight is comparatively low (does not exceed 3.0 KDa).
They also exist in combination with nucleic acids in the nucleus (nucleoprotein).
Protamines are water-soluble and ammonia soluble proteins, non-coagulable and have the property of precipitating aqueous solutions of other proteins.
Their characterstic solubility in ammonia, differentiates them from histones, which are simple proteins also.
Protamines are strongly basic, due to the presence of large amount of basic amino acids specially arginine. Salmine, which is an example of protamines, contains arginine in amount reaching 90% of the total amino acids. Other basic amino acids may be present e.g. lysine and histidine. They do not contain cysteine, tryptophan and tyrosine.
Due to the excessive basic charges of protamines they are readily soluble in dilute acids.
The protamines are classified according to their component of basic amino acids into:
1. Mono-protamines which contain arginine only.
2. Diprotamines which contain lysine and histidine.
3. Triprotamines which contain arginine, lysine and histidine.
Protamines are present in sperms, especially those of fishes. Protamines are also present in ova. In plants, protamines are present in pollen grains.
Examples: Salmine and thymine, which are obtained from salmon and tuna fishes.
Histones are basic proteins, which are characterized, by being soluble in water, dilute acids, and insoluble in dilute ammonia. Their insolubility in ammonia differentiates them from protamines.
They are usually present associated with nucleic acid and porphyrins. They are not present in plants.
The most important amino acids entering in the structure of histones are arginine, histidine and less lysine. The amount of their basic charges is less than protamines.
Histones are weakly coagulated by heat. The coagulum is soluble in very diluted acids thus differing from coagulated albumins and globulins that increase in this condition.
Histones are present in glandular tissues as liver and spleen. The protein globin, which enters in the formation of the hemoglobin of blood, is similar to histones.
Globins are found to contain tryptophan, tyrosine, arginine, histidine and lysine in the molecular ratio of approximately, 2 : 3 : 3 : 8 : 9. Thus they are atypical histones because they contain low arginine.
Gliadins are never present in animals but only present in plant kingdom. They are present in high concentrations in cereals and have been obtained from all grains except rice
Gliadins are also called prolamines due to the presence of a high percentage of the amino acids proline (10-14%) and glutamine.
The amino acid lysine is deficient in gliadins.
Gliadins are proteins, which are insoluble in water and saline, but soluble in 7080% alcohol due to the presence of excess proline.
The examples of prolamines are gliadin of wheat and zein of maize. Gliadins are deficient in lysine and tryptophan, while herodein is low in lysine and valine.
They are plant proteins.
They are soluble in diluted acids but not in water or diluted salt solutions.
They are very rich in glutamic acid
They have very large molecular weight and are heat coagulable.
Examples are oryzenin of rice and glutelin of wheat.
Scleroproteins are characterized by their extreme insolubility in water, dilute acids and the most common reagents. They are extracellular fibrous proteins and never present inside the cells.
Their main function is the protection of the body. Hairs, nails, natural silk and connective tissues, contain scleroproteins. Albuminoids are never present in plants.
The main important groups of scleroproteins are: a.
Keratin is a typical fibrous protein. It consists of long polypeptide chains. The peptide chains may be coiled or reset in spiral or helix types.
-They are cross-linked by S-S bonds as well as by hydrogen bonds. The coiled form is referred to as alpha keratin. It changes to beta keratin by unfolding due to denaturation.
Keratins are highly insoluble compounds. They are insoluble in all protein solvents, and are not digestible by proteolytic enzymes (e.g., pepsin and trypsin).
Keratins are hydrolyzed by prolonged boiling with alkalis. The sulfur content of keratin is high. It is present in the form of cystine, which is responsible for the stability and insolubility of keratins. Most keratins yield histidine, lysine and arginine amino acids on hydrolysis, in a molecular ratio of, 1:4:12, respectively.
Barium sulfide is one of the important substances that dissolve keratins. For this reason this material enters in the formation of cosmetics dealing with removal of hair.
Keratins are present in hairs, nails and superficial layer of the skin. They form the intermediate filaments of the cytoskeleton in the epithelial cells where their expression is stable to trace the origin of these cells. They composed of more than 20 type of polypeptides. The cystine content is about 14%. This is why is a good source of cystine.
They are present in white fibrous connective tissues, tendons and bones. They form about 30% of total body protein.
Collagen molecules consist of 3 polypeptide chains, they are twisted around each other forming triple helix molecule . They are held together by hydrogen bonds .
Each helical turn contains only 3 amino acids. There are 19 types of collagen formed of different combinations of 30 type of subunits (polypeptides).
Collagen is rich in glycine, proline and hydroxyproline but low in sulfur containing amino acids. Glycine, proline and hydroxy proline form about 2/3 of the total amount of amino acids present in the collagen molecule. The amino acids sequence is glycine -X-Y where X is frequently proline and Y is often hydroxyproline or hydroxylysine
Collagen is deficient in tryptophan and cysteine. Collagen differs from keratins in containing much less sulfur.
Collagen is insoluble in water, dilute acids and alkalis. From this point of view it is similar to elastins.
Collagen is resistant to peptic and trypsin digestion, but when boiled for a long time with water, dilute acids or alkalis, it changes to gelatin. Thus, gelatin is a derived protein obtained from the partial hydrolysis of collagen. Both gelatin and collagen are of little nutritive value because they contain about 40% of the non-essential amino acid glycine.
It is the product of prolonged boiling of collagen in water. It is easily digested and has the property of forming a gel on cooling (gel formation).
Gelatin is a very good diet for patients because it is an appetizer and easily digested.
Gelatin is deficient in certain essential amino acids. So it is not an adequate protein diet, as it is deficient in tryptophan and cysteine and contains very small amounts of methionine (protein of low biological value).
They are present in the yellow fibers of the connective tissues which are mainly present in lungs, uterine wall during pregnancy, tendons and ligaments. Elastins are also present in the elastic tissues of tendons and big arteries.
It is formed of 4 polypeptide chains interconnected through their lysine residues.
It is rich in alanine, leucine, valine and proline but deficient in cysteine, methionine, hydroxylysine and histidine.
They are insoluble in water, dilute acids, dilute alkalis and saline. Boiling with strong acids or strong alkalis or hydrolysis by digestive elastase librates free amino acids.
Reticulins are present in the reticular tissues such as spleen, liver and lymph glands.
In properties, reticulins are very much similar to elastin and collagen. Reticulins are unaffected by dilute acids or alkalies.
They are simple proteins combined with a non-protein group called prosthetic group. So on hydrolysis, they give amino acids and prosthetic group.
They include:
1. Phosphoproteins:
- These are proteins conjugated with phosphate. Phosphate is attached to OH group of serine, tyrosine or threonine present in protein.
- They are found in: a. Casein: The main milk protein.
b. Vitellin: It is present in egg yolk.
2. Lipoproteins:
- These are proteins conjugated with lipids converting them into water soluble Substances. Examples: Plasma lipoproteins.
- These are proteins conjugated with carbohydrates in varying amounts attached as short or long chains.
- Examples: Mucous secretion of gastrointestinal tract and Glycoproteins of cell wall.
- These are proteins conjugated with metals such as;
a) Iron: e.g., ferritin is intracellular iron-binding protein and Transferrin is an iron-binding transport protein in the blood.
b) Zinc: e.g., Insulin hormone present in crystals containing zinc.
c) Copper: e.g., Ceruloplasmin: is a protein present in blood. It is responsible for the oxidation of Fe2+ ions to Fe3+ ions.
d) Selenium: e.g., Glutathione peroxidase.
- These are proteins conjugated with colored pigment.
- Example; Hemoglobin and cytochrome enzyme present in mitochondria contains haem pigment, which is red in color.
- Nucleoproteins are conjugated proteins formed of non-protein prosthetic group (nucleic acid) attached to one or more molecules of a simple protein.
- This simple protein is usually a basic protein such as histones or protamines. Nucleoproteins are found in all animal and plant tissues but are most easily isolated from yeast or from cells whose nuclei are densely packed, e.g., thymus cells. Structure of viruses is almost a nucleoproteins complex.
- Ribosomes and other ribonucleoprotein complexes: They are proteins conjugated with RNA.
- They include:
A. Primary derived proteins:-
Denatured protein: e.g., coagulated albumin or globulin or gelatin
B. Secondary derived proteins :Hydrolytic product of protein: e.g., Protein Proteoses Peptone Polypeptide.
1. Proteoses: they are soluble in water, not coagulable and precipitated by saturated salt solution.
2. Peptones: are soluble in water and not coagulable by heat.
3. Peptides: are soluble in water and salt solution, not coagulable by heat. They are formed from 2 or more amino acids.
- The amino acids in any polypeptide chain are arranged so that the first amino acid has a free amino group (N-terminal end) and the last one has a free carboxyl group (C-terminal end). So the tripeptide alanine-cysteine-tryptophan is different from the tripeptide tryptophan-cysteine-alanine.
4. Amino acids.
What is an enzyme
Enzymes specificity
Mechanisms of enzyme action
Nomenclature / enzyme classification
Factors affecting velocity of reactions
Enzyme kinetics
Enzyme inhibition
It is globular protein
Ribozymes are RNA molecules with enzymatic activity
behaviour of any enzyme depends upon its primary, secondary, tertiary or quaternary structure
Enzymes of digestive tract and those found in blood are present in inactive form called zymogen or proezymes.
a. Enzymes are composed of long chains of amino acids that have folded into a very specific three-dimensional shape which contains an active site.
2-Relative, low or bond specificity
In this type the enzyme acts on substrates that are similar in structure and contain the same type of bonds e.g.
Amylase, which acts on α 1-4 glycosidic, bonds in starch, dextrin and glycogen
Lipase that hydrolyzes ester bonds in different triglycerides
3-Moderate, structural or group specificity
In this type of specificity, the enzyme is specific not only to the type of bond but also to the structure surrounding it for example:
a-Pepsin is an endopeptidase that hydrolyzes central peptide bonds in which the amino group belongs to aromatic amino acids e.g. phenyl alanine, tyrosine and tryptophan.
b-Trypsin is an endopeptidase that hydrolyzes central peptide bonds in which the amino group belongs to basic amino acids e.g. arginine, lysine and histidine
4-Optical or stereo-specificity
In this type of specificity, the enzyme is specific not only to the substrate but also to its optical configuration b-α- glycosidase acts only on αglycosidic bonds, which are present in starch, dextrin and glycogen.
We can digest glycogen and starch due to presence of αglycosidase, but we can not digest cellulose due to the absence of β- glycosidase
A- The enzyme may act on two substrates by one reaction type. e.g. xanthine oxidase enzyme acts on xanthine and hypoxanthine (two substrates) by oxidation (one reaction type).
hypoxanthine Xanthine oxidase Xanthine acid
Xanthine oxidase uric
B- The enzyme may act on one substrate by two different reaction types e.g. isocitrate dehydrogenase enzyme acts on isocitrate (one substrate) by oxidation followed by decarboxylation (two different reaction types).
The enzymatic reactions takes place by binding of the substrate with the active site of the enzyme molecule by several weak bonds. E + S ‹ › ES › E + P Formation of ES complex is the first step in the enzyme catalyzed reaction then ES complex is subsequently converted to product and free enzyme
IUBMB has recommended system of nomenclature for enzymes & according to them each enzyme is assigned with two names: Trivial name (common name, recommended name). Systemic name ( official name ).
Systemic name
o Each enzyme is characterized by a code no.called Enzyme Code no. or EC number and contain four Figure (digit) separated by a dot. e.g. EC m. n. o. p
o First digit represents the class;
o Second digit stands for subclass
o Third digit stands for the sub-sub class or subgroup;
o Fourth digit gives the serial number of the particular enzyme in the list.
o e.g. EC 2.7.1.1 for hexokinase
According to the IUBMB system of enzyme nomenclature enzymes are grouped into 6 major classes
EC 1 OXIDOREDUCTASES
EC 2 TRANSFERASES
EC 3 HYDROLASES
EC 4 LYASES
EC 5 ISOMERASES
EC 6 LIGASES
Rate of the reaction or velocity is directly propostional to the Enzyme Concentration when sufficient substrate is present.
Study of reaction rate and how they changes in response to change in experimental parameter is known as kinetics.
Amount of substrate present is one of the key factor affecting the rate of reaction catalyzed by an enzyme in vitro.
Effect of Substrate Concentration on Reaction Velocity
o Regulate:to control or direct according to rule,principle or law
:is the control of the rate of a reaction catalyzed by an enzyme by some effector (e.g inhibitors or activators ) or by alteration of some condition (e,g ph or ionic strength
o Five ways by which enzyme activity in the cell can be changed:
1. Enzyme production: synthesis or degradation.
2. Compartmentation:different metabolic pathways in different cell compartments.
3. Activation and inhibition:by activators or inhibitors, for example, feedback inhibition by one of the products of reaction.
4. Post-transitional modification:by phosphorylation,methylation and glycosylation
5. Localization to a different environment:from an reducing cytoplasm to oxidizing periplasm, high ph to low ph,low salinity to high salinity or high to low energy charge
o Any substance that can diminish the velocity of an enzyme catalyzed
o These include drugs, antibiotics, poisons, and anti-metabolites.
o Useful in understanding the sequence of enzyme catalyzed reactions, metabolic regulation, studying the mechanism of cell toxicity produced by toxicants.
o Forms the basis of drug designing
1. Reversible inhibitors
2. Irreversible inhibitors
A reversible inhibitor inactivates an enzyme through noncovalent, more easily reversed, interactions. Unlike an irreversible inhibitor, a reversible inhibitor can dissociate from the enzyme.and once removed, allows the enzyme it was inhibiting to begin working again. It has no permanent effects on the enzyme - it does not change the shape of the active site,
Reversible inhibitors can be classified into : 1.Competitive 2.Non-competitive 3. Un-competitive
In competitive inhibition:
o an inhibitor that resembles the normal substrate binds to the enzyme, usually at the active site, and prevents the substrate from binding. At any given moment, the enzyme may be bound to the inhibitor, the substrate, or neither, but it cannot bind both at the same time.
o V max is decreased in competitive inhibitors
o Km increases if a competitive inhibitors needs more substrate to achieve half of Vmax
Example: is the antineoplastic drug methotrexate. Methotrexate has a structure similar to that of the vitamin folic acid (Fig. 4-5). It acts by inhibiting the enzyme dihydrofolate reductase (DHFR), preventing the regeneration of dihydrofolate from tetrahydrofolate. This interferes with DNA synthesis and blocks cell division in rapidly dividing cancer cells
Non-competitive inhibition:
This poison causes death by halting ATP production. In essence, it binds to the allosteric site of the enzyme cytochrome oxidase (a carrier molecule that is part of the electron transport chain). Cyanide changes the shape of the active site so that the enzyme cannot pass electrons on
Un-competitive Inhibiton:
o Binds only to the enzyme-substrate complex.
o Does not have the capacity to bind to the free enzyme.
o Not overcome by increasing substrate concentration .
o Both the Km and Vmax are reduced
Irreversible enzyme inhibition: is the modification of an enzyme by an inhibitor that makes the chemical reaction irreversible
Example:
The organophosphate pesticides such as malathion, parathion, and chlorpyrifos irreversibly inhibit acetylcholinesterase
has several functions in cellular energy metabolism
composed of 3 isoenzymes that differ slightly in structure
Clinically, creatine kinase is assayed in blood tests as a marker of damage of CK-rich tissue
CPK is a dimer made up of 2 subunits called “B” for brain and “M” for muscle.
catalyzes the reversible transfer of high-energy phosphate from ATP to creatine creatine, facilitating storage of energy in the form of phosphocreatine
In muscle cells, it represents extra energy that plays a pivotal role in maintaining ATP homeostasis
B)
: In tissues and cells that consume ATP rapidly, especially :
skeletal muscle and smooth muscle
brain
photoreceptor cells of the retina
hair cells of the inner ear
spermatozoa
C) Reference range in serum affected by: Amount of lean muscle mass o Thin, sedentary= 30- 50 U/L o Muscular, exercising regularly = 500- 1000 U/L Age inneonated CK- MB 5- 10%of total CK Gender Race african 30% higher than Europeans muscle activity direct relationship between intensity of exercise and CK level.
Lactate dehydrogenase
it is tetramer , made up of 2 subunits , M-muscle & H-heart consists of 5 iso-enzymes LDH1,LDH2,LDH3,LDH4 &LDH5 which are separated by cellulose acetate electrophoresis at pH 8.6 A)Normal values :
Urine 40 -100 U/L
Is technique by which separation of movement of charged particles through an electrolyte when subjected to electrical field.
C) Identification using gel electrophoresis :
The different form can be separated by electrophoresis
The difference in electrophoretic mobilities dueto different electric charges on the isoenzymes due to difference in content of acidic and basic amino acids.
H gene (higher number of acidic residues) is more strongly negatively charged than M gene
LDH-1 has the highest negative charge moves fastest due to it has more acids
In normal serum
LDH5 is rarely seen.
LDH1 levels are greater than LDH2. myocardial infarction,
50 times upper limit of LDH 1 and LDH 2 Megaloblastic anemia
LDH 3 is increased Renal disease- tubular necrosis or pyelonephritis, pulmonary embolism (massive destruction of platelets)
Total LDH is increased neoplastic diseases
LDH5 (M4) is increased Muscular dystrophy , cancer, malignancies of CNS, prostatic carcinoma
10 times more LDH5 Toxic hepatitis with jaundice
LDH 2 & LDH 3 is increased leukaemias
LDH 2 , LDH3 & LDH5 levels are increased malignant tumors of testis and ovaries
✔ Characters of vitamins.
✔ Classification of vitamins.
✔ Minerals
• They are essential organic compounds for normal health and growth since they are not synthesized in human body.
• They must be supplied in the diet in the required very small amounts that deficiency of any vitamin in the body results in a disease.
• They are present in normal food in small amounts.
• They act as coenzymes.
• They do not enter in tissue structure as carbohydrates, fats and proteins.
• They are not oxidized for energy production as carbohydrates, fats and proteins.
Provitamins: These are precursors of vitamins that converted into vitamins inside the body e.g., Carotenes are provitamin A.
Vitamers: These are different forms of one vitamin e.g. Vitamin D has 2 vitamers; D2 & D3.
Classification
Vitamins can be classified according to their solubility into two main categories:
Animal sources: (RETINYL ESTER)
• Liver, eggs and milk fat.
• Fish liver oils e.g. shark liver oil.
Plant sources:
• in plants as provitamin A; Carotenes
• carrots, potato and tomatoes.
• Vitamin A acts as a hormone and acts on DNA
it has the following functions:
- a role in Reproduction
- a role in Growth.
- Retinol is essential for normal growth and bone & teeth formation.
- Maintenance of epithelial cells.
- Antioxidant (anticancer) action
Role of vitamin A in Vision = Visual cycle =Rhodopsin cycle:
Deficiency of vitamin A:
1-Eye:
a- Night blindness: impaired dark adaptation.
b- Xero-ophthalmla: dryness and roughness of cornea.
2-Growth retardation.
3-Skin and mucus membranes: a-Roughness of skin (goose skin) b-mucus membranes e.g. urinary system. This leads to infection.
• It’s called Anti-haemorrhagic vitamin.
• It has three vitamers: K1, K2 and K3
1. Naturally occurring vitamin K (fat soluble):
a- Vitamin K1 (phylloquinone): is the major form of vitamin K in plants e.g. spinach, cauliflower and cabbage.
b- Vitamin K2 (menaquinone): is formed inside the intestine by intestinal bacteria.
2. Synthetic: (e.g. K-viton drug)) is water-soluble Vitamin K3 (Menadione) and it is the most potent member.
Functions of vitamin K:
1) In liver Synthesis of some blood clotting factors prothrombin (factor II), and factors VII, IX and X
2) In bones Synthesis of osteocalcin (calcium binding protein).
Deficlency of vitamin K: - It is rare because intestinal bacteria synthesize it and it leads to impairment of blood clotting.
Vitamin K Vitamin E (tocopherols)
• It is called Antioxidant & anti-sterility vitamin.
Vitamin E in the diet is called Tocopherols and there are four types of tocopherols
;
1) tocopherol 2) β- tocopherol
2) γ- tocopherol 4) δ-tocopherol
Sources: Vegetables and seed oils e.g. cotton, olive and coconut oils.
Functions:
A. Antioxidant:
1) It prevents oxidation of cell components (e.g. polyunsaturated fatty acids, DNA and cell membranes) by free radicals.
2) It is natural antioxidant that added to oils to prevent the rancidity.
3) It protects against heart diseases: As it acts as antioxidant so it prevents oxidation of LDL. because Oxidized LDL causes heart diseases
4) It prevents hemolytic anemia through keeping of the integrity of RBCs membrane preventing their heamolysis.
B. Antisterility effect
Vitamin D (Calciferol)
Vitamin D has two vitamers: a-Vitamin D2(ergocalciferol) : its Provitamin is Ergosterol found in plants especially yeast.
b-Vitamin D3(Cholecalciferol)(inactive): its Provitamin is 7- dehydrocholesterol derived from Cholesterol.
Functions of vitamin D: • 1,25 dihydroxycholecalciferol (calcitriol) (active form) acts as a hormone, having the following functions:
1. Normalization of serum calcium:
- On intestine: It stimulates synthesis of a protein called "calcium binding protein" that responsible for calcium absorption
- On bones: It stimulates calcium moblization from bones. - On kidneys: It increases reabsorption of calcium. 2. Mineralization of bones: After normalization of serum calcium 3. Absorption of phosphate: From intestine and it increases also reabsorption of phosphate by kidney.
Vitamin C = L-Ascorbic Acid
Deficiency of vitamin D: • Deficiency of vitamin D causes demineralization of bones that leads to: 1) Rickets in children: characterized by bone deformities. 2) Osteocalcin in adults: characterized by bone fractures. • It is called Antiscorbutic vitamin. Sources: - Fruits especially citrus fruits like lemon, orange, melon and strawberry. - Vegetables especially green leafy vegetables such as lettuce, tomatoes, raw cabbage, green peppers and germinating seeds are rich source of the vitamin. - Liver and adrenals are animal sources of vitamin C but are useless due to destruction of vitamin C by cooking. Guava is very rich in vitamin C while Milk is a poor source of vitamin C. Functions of vitamin C: A) Formation of collagen protein. Procollagen Collagen
Deficiency:
1- Pellagra: because PLP is needed for the conversion of tryptophan to niacin.
2- Growth retardation & mental retardation: disturbance in amino acids metabolism.
Vitamin B12 = Cyanocobalamin
• It is called Anti-pernicious anemia or it is an extrinsic factor
Sources:
- Meat, egg, milk and milk products.
- Intestinal microorganisms synthesize B12 in human colon.
Vitamin B12 is not present in vegetables.
Functions:
- Vitamin B12 acts as coenzyme after being converted to methylcobalamin for:
A) Methylation of homocysteine to methionine: Is important for haemopoiesis (participate in purine, pyrimidine, and nucleic acid syntheses).
B) oxidation of odd number fatty acids which help in the formation of myelin sheath through the metabolism of odd number fatty acids.
Deficiency:
1- Megaloblastic anaemia. 2- Neurological manifestations.
Sources:
• NOTE: Folacin = Peteroyl Glutamic Acid
• The major source is leafy vegetables.
• Liver, kidney and yeast are also rich in folate.
Functions:
• Tetrahydrofolic acid (H4 folate) act as a carrier for one–carbon groups.
Deficiency:
1- Pancytopenia: all blood cells are affected
a) Megaloblastic anaemia (Marocytic, hyperchromic). b)Leucopenia: W.B.Cs. c)Thrombocytopnea: Platelets.
2- Impaired growth.
Functions:
• It has Physiological Functions
• It acts as coenzyme in e.g. pyruvic acid into acetyl coA.
Functions:
A) Coenzyme A (CoASH)
B) Acyl carrier protein (ACP)
• NOTE: ACP acts as acyl carrier for fatty acid synthesis.
• The minerals, while forming only a small portion of the total body weight, are nonetheless of great importance in the vital economy.
• Most of the minerals are essential components of an adequate diet.
• The principal sources of the mineral elements are fruits, vegetables and cereals. According to the body needs Minerals may be divided into two groups
- They are required in amounts greater than 100 mg/day.
- They include seven elements:
• Calcium • phosphorus • magnesium • sodium •potassium • chloride
•sulfur.
- They are required in amounts less than 100mg/day.
- Some are essential trace elements e.g:
• Chromium • cobalt •copper • fluorine • iodine •iron •manganese • molybdenumn
•selenium • zinc.
Sources:
• Iron is present in all cells.
• It does not exist in the free state but always present in organic combination.
•The best source of iron is organ meats, liver, heart, kidney and spleen.
Functions of iron : Iron plays a number of important roles in the body:
1. As a component of hemoglobin and myoglobin it is required for O2 and CO2 transport.
2. As a component of cytochromes and non-heme iron proteins it is required for oxidative phosphorylation.
3. As a component of the essential enzyme, (lysosomal) myeloperoxidase it is required for phagocytosis and killing of bacteria by neutrophils.
Trace elements*: Elements in the body in very low amounts (micrograms/gram or less)4. Lactoferrin binds iron in milk, it facilitates the transfer of iron to intestinal receptor in the infant. It also inhibits microbial growth.
- The total body iron of an adult male is 3-5g. It is distributed as follows:
• RBCs iron (hemoglobin) 66%,
• tissue iron 33%
• plasma iron 1%.
Alterations of plasma iron:
1. Iron deficiency anemia is caused by:
• Deficient intake.
• Impaired absorption: e.g. Steatorrhea, abdominal surgery.
•Excessive loss e.g. menstrual loss, gastrointestinal bleeding, bleeding due to some parasites (anchylostoma).
Biochemical changes:
1) Plasma iron is decreased.
3) Plasma TIBC is increased.
2) Plasma ferritin is decreased. 4) RBCs are hypochromic and microcytic.
2. Iron overload is caused by:
• Repeated blood transfusion.
• Intravenous administration of iron.
• Hemochromatosis (hemosiderosis, Bronz diabetes)
- This is a rare hereditary disease characterized by abnormal increase of iron absorption.
-Iron is deposited in the form of hemosiderin in liver , pancrease and skin .
Biochemical changes:
1)Plasma iron is increased. 2)Plasma TIBC is decreased.
3)Plasma ferritin is increased.
Requirements: - Adults: 10 mg/day. - Pregnant and lactating women: 30 mg/day.
Copper (Cu2+ )
Sources: - The richest sources of copper are nuts, liver, kidney - cow’s milk is a poor source of copper.
Body Copper :
• The adult human body contains 100 – 150 mg of copper.
Functions of Copper :
1. It is essential for hemoglobin synthesis.
2. It enters in the formation of bone.
3. Maintains the myelin within the nervous system. Alterations of plasma copper:
1. Hypercupremia: Occurs in infections,
2. Hypocupremia: decreased plasma copper or Wilson’s disease* (hepatolenticular degeneration).
* This disease is characterized by accumulation of large amounts of copper in: 1. Liver causing hepatic cirrhosis.
2. Lenticular nucleus of the brain causing lenticular degeneration with abnormal movement. 3. Cornea: Causing greenish-brown discoloration of the corneal margin which is called: Kayser-Fleisher rings.
Zinc (Zn2+)
Sources:
• liver, eggs, seafood and milk.
Body zinc: The adult male body contains about 2g of zinc.
Functions of zinc:
1. Zinc is important for growth and reproduction. It is a cofactor for RNA and DNA polymerases, so RNA and DNA synthesis.
2. It plays a role in tissue repair, wound healing and hair and nail integrity.
3. Zinc is an essential cofactor for a number of enzymes including: alkaline phosphatase, lactate and malate dehydrogenases,
4. Zinc is required for mobilization of vitamin A from the liver.
5. Insulin forms complex with zinc, this increases the duration of insulin action when given by injection. In the B cells of the pancreas is used to store and release insulin.
6. Immunostimulant.
- Carbohydrates are substances containing carbon, hydrogen and oxygen having the general formula CnH2nOn. (eg. C3 H6 O3)
H3C-CH-COOH OH
- Hydrogen and oxygen are present in 2:1 ratio the same ratio as water, so the French called them “Hydrates de Carbon”, i.e., carbohydrates Cn(H2O)n
- Carbohydrates are aldehyde (CHO) or ketone (C=O) derivatives of polyhydric alcohols (have more than one OH group) or compounds which yield these derivatives on hydrolysis.
The old definition is inaccurate because:
1. There are substances which are not carbohydrates but have the formula CnH2nOn, e.g., acetic acid CH3COOH (C2H4O2) and lactic acid.
2. There are some carbohydrates, which do not have this general formula, e.g., rhamnose, amino sugars and deoxy sugars.
1. The chief source of energy.
Importance of carbohydrates:
2. Important structural components in animal and plant cells.
3. Important part of nucleic acids and free nucleotides and coenzymes.
5. Biological role as a part of hormones and their receptors and enzymes.
4. Major antigens are carbohydrates in nature, e.g., blood group substance.
They contain one sugar unit, i.e., and the simplest form of sugars and cannot be further hydrolyzed. They represent the end products of carbohydrate digestion in the human body.
According to the number of sugar units in the molecule there are three types:
They contain 2 – 10 monosaccharide units per molecule and give monosaccharides on acid hydrolysis.
They contain more than 10 monosaccharide units per molecule and give monosaccharides on acid hydrolysis.
They are classified according to the number of carbon atoms into five important groups.
Each of these groups is subdivided according to the type of functional chemical group into: Aldoses (sugars containing aldehyde group) and Ketoses (sugars containing ketone group).
1. Bioses: are monosacch arides containing 2 carbon atoms, e.g. CHO CH2OH Glycolaldehyde
2. Trioses: are monosaccharides containing 3 carbon atoms, e.g. A- Aldotriose: e.g,.
3. Tetroses: are monosaccharides containing 4 carbon atoms:
A- Aldotetroses: - D- and L-isomers are mirror images to each other that is only recognizable when there are more than one asymmetric carbon atom (see later on in this chapter).
B- Ketotriose: e.g., Dihydroxyacetone, the hydroxylated form of Acetone
4. Pentoses: they contain 5 carbon atoms. Ribose is the most important pentose because it enters in the structure of DNA and RNA and important free nucleotides such as ATP and coenzymes (see nucleic acids):
A- Aldopentoses:
B- Ketotetroses:
B- Ketopentose:
5. Hexoses: are monosaccharides containing 6 carbon atoms. They are the most important monosaccharides particularly glucose:
A- Adohexoses:
Glucose: is called grape sugar, blood sugar or Dextrose because it is dextrorotatory. It is fermentable, reducing and gives It is produced by hydrolysis of starch, dextrins, glycogen, sucrose, maltose and lactose. All monosaccharides have to be converted into glucose to be utilized in the body. It appears in urine in diabetes mellitus.
Galactose: is a subunit of the milk sugar, lactose. It is synthesized from glucose in the body. It enters in structure of glycoproteins, glycolipids and mucopolysaccharides in its amine form. It is nonfermentable
B- Ketohexoses:
Fructose: is the sweetest sugar known. It is the main sugar in bee's honey and fruits. semen sugar.
Metabolizable sugars in human body are D-forms only. Examples are Dglucose and L-glucose or D-mannose and L-mannose. Glyceraldehyde is called the reference sugar because it contains one asymmetric carbon atom.
They are stereoisomers which differ in distribution of H and OH group around the asymmetric anomeric carbon atom C1 in aldoses or C2 in ketoses after cyclization of the molecule, e.g., - and -glucose are anomers.
Chemical reactions of monosaccharides
Aldose (-CHO) is oxidized into Acid (-COOH).
The product of oxidation depends on the nature of oxidizing reagent,
1. Oxidation by mild or weak oxidizing reagent:
It oxidizes the first carbon and gives Aldonic acid, e.g., Bromine water, and examples include:
(A) Glyceric acid is important in metabolism of carbohydrates in the body.
(B) Gluconic acid is an intermediate in carbohydrate metabolism and used as Ca2+ gluconate for intravenous supplementation of calcium for slow dissociation. Another example from hexoses is galactose gives galactonic acid.
(C) L-ascorbic acid (vitamin C), is synthesized from gulose (a glucose derivative)in plants and animals except human, primates and guinea pig. It is an antioxidant.
(D) Sialic acid is a carbohydrate-derived acid that is enters in glycolipids and glycoproteins synthesis.
2. Oxidation by moderate oxidizing reagents:
- Such as H2O2 (hydrogen peroxide) or diluted HNO3. They oxidize the last carbon (CH2OH) to give uronic Acids.
Example include:
(A) Glucuronic acid: It is synthesized in the liver. Biological importance of glucuronic acid
1. Glucuronic acid enters in the structure of very important molecules such as mucopolysaccharides.
2. It is used for excretion of bilirubin and steroid hormones and detoxication of certain drugs and several toxins by conjugation.
3. L-iduronic acid is the 5-epimer of Dglucuronic acid and it enters in structure of mucopolysaccharides.
Concentrated HNO3 oxidizes the first and last carbon to give aldaric acid. This reaction is important to differentiate between glucose and galactose since glucaric acid is water soluble, whereas, mucic acid is insoluble in water and precipitates in the form of insoluble crystals of specific shape.
II. Reduction of monosaccharides (sugar alcohols):
Reduction of monosaccharides gives sugar alcohols. Examples: Reduction of Glyceraldehyde gives glycerol that enters in structure of lipids, creams and explosives.
Ribose reduction gives Ribitol that is a part of the structure of vitamin B2 (Riboflavin).
Reduction of glucose gives sorbitol or glucitol that enters in medical industries
Replacing OH group on C2 by an amino group (NH2) produces them.
1. Glucosamine: Glucosamine is also called chitosamine because is the only sugar derivative in chitin. It can be acetylated into N-acetyl glucosamine and sulfated into sulfated glucosamine.
Biochemical importance:
1. Enters in the structure of exoskeleton of insects (chitin, so it is called chitosamine).
2. Enters in the structure of mucopolysaccharides, hyaluronic acid contain N-acetyl glucosamine and heparin contain sulfated glucosamine
2. Galactosamine (Chondrosamine): the major sugar derivative in chondroitin sulfate), it may be acetylated or sulfated.
Biochemical importance:
It enters in the structure of the sulfate containing mucopolysaccharides (chondroitin sulfate) and glycolipids.
IV.Deoxysugars:
These are sugars in which OH group is replaced by H. This may occur at either of three places:
1. At C2 gives Deoxy sugar proper, e.g., deoxyribose that enters in structure of DNA.,
2. At C6 gives methyl pentoses (Methylose), e.g., L-galactose gives L-fucose and, L-Mannose gives L-rhamnose. L-rhamnose and L-fucose enter in the structure of glycoproteins, e.g., blood group substance.
3. At C3, as in Sialic acid, see above for importance.
A) Maltose (malt sugar):
It consists of 2 -glucose units linked by 1,4-glucosidic linkage, B) Isomaltose:
- It is formed of a -glucose linked by 1,6-glucosidic linkage with glucose.
- It is not present in nature. It is produced during digestion of starch by amylase enzyme
C) Lactose:
- It is formed of -galactose and -glucose linked by -1,4-glucosidic linkage galactose participates by C1 while glucose participates by C4. - It is the milk sugar
D) Cellobiose:
It is formed of 2 -glucose units linked by 1,4-glucosidic linkage. It is a reducing disaccharide. It is the building unit of cellulose. It is nonfermentable, indigestible, reducing, shows mutarotation and have and - forms.
A) Sucrose:
- It is table sugar and sugar of cane and molasses and is formed of -glucose linked to fructose by --1,2linkage. It is a fermentable sugar.
- The 2 anomeric carbons (C1 of glucose and C2 of fructose) are involved in the linkage, therefore it is:
Non-reducing sugar.
They are classified into: 2. Heteropolysaccharides.
They yield only one type of monosaccharides on hydrolysis and they are named according to the type of that monosaccharide, e.g.,
Pentosans + H2O Pentoses
Glucosans:
Hexosans + H2O Hexoses
They produce only glucose on hydrolysis. They include; starch, dextrins, dextrans, glycogen and cellulose.
A. Starch:
It is the stored form of carbohydrate of plants. It never exists in animals.
It is present in cereals such as wheat and rice and tubers such as potatoes and sweet potatoes.
It is in the form of starch granules.
The core is amylose (20%) and the shell is amylopectin (80%). Due to its high molecular weight it forms colloidal solution in hot water.
It forms the inner part of starch granules and is watersoluble and gives blue color with iodine. Straight chain compound present in the form of a helix formed of a large number of -glucose units linked by -1,4-glucosidic bond.
It forms the outer coat of starch granule and is insoluble in water. It gives red color with iodine. It is branched chains formed of a large number of -glucose units linked by 1,4-glucosidic linkage along the branch and by -1,6-glucosidic linkage at the branching point that occur periodically every 25-30 glucose units
It is the stored form of carbohydrate in animal, particularly in muscles and liver. It is water-soluble and gives red color with iodine.
Its structure is similar to amylopectin a branched tree with -1,4- glucosidic linkage along the branch and -1,6-glucosidic linkage at the branching point. The glycogen tree is shorter and more branched (a branch point every 8-10 glucose units) than amylopectin.
It is digestible because human amylases hydrolyze -glucosidic linkage.
It is a structural polysaccharide and forms the skeleton of plant cells and does not enter in animals cell structures.
It is a straight chain molecule formed of a large number of -glucose units linked by -1,4-glucosidic linkage.
Neutral nitrogenous heteropolysaccharides or Glycoproteins:
They do not contain uronic acids or sulfate groups (which give acidic characteristics).
They are formed of a large protein core to which are attached smaller branched or unbranched chains of carbohydrate.
Distribution: Glycoproteins are widely distributed in mammalian tissues.
They include:
1. Mucins of epithelium lining of gastrointestinal, urogenital and respiratory tracts are lubricant and protective.
4. Mineral and vitamin transporting protein, e.g., trasferrin.
7. Some enzymes such as peptidases and alkaline phosphatase.
2. Cell membranes where they play an important part in cell-cell attachment and viral or bacterial cell recognition.
3. Blood group substances A and B antigens.
5. Immunoglobulins (antibodies), IgG, IgM, IgA … etc.
8. Intrinsic factor that is responsible for vitamin B12 absorption.
6. Some hormones, e.g., anterior pituitary hormones: LH and FSH.
9. Structural function as a part of collagen of connective tissue.
B. Acidic nitrogenous heteropolysaccharides or mucopolysaccharides:
Proteoglycans are conjugated form of mucopolysaccharides with proteins.
They consist of very small core protein molecule to which a huge carbohydrate tree is attached. The carbohydrate part is highly complex and is called glycosaminoglycans or mucopolysaccharides. Mucopolysaccharides also exist as free soluble form, e.g., heparin. Sugars participating in mucopolysaccharides include glucuronic acid, iduronic acid, glucosamine, galactosamine and xylose. It constitutes 5% of cell membrane structure, presenting on the outer surface of the membrane.
They have two types: sulfate-free and sulfated
A. Sulfur-free mucopolysaccharides: Their sugar units are not sulfates, e.g., hyaluronic acid.
B. Sulfur-containing mucopolysaccharides:
1. Chondroitin sulfate.
2. Heparin.
✔ Steps of glycolysis
✔ Bioenergetics of glycolysis
✔ Oxidation of extramitochondrial NADH.H+
✔ Regulation (or Control) of Glycolysis:
It is a cascade of reactions that converts glucose into two pyruvate molecules (When O2 is available) or into lactate (when O2 is not available, i.e., no mitochondria) aiming at production of ATP and other intermediates. It is also utilized in its opposite direction in gluconeogenesis.
It occurs in the cell cytoplasm of all tissues of the body. It is especially important in:
1.RBCs: are devoid of mitochondria and depend on glycolysis as the main source of energy. Mammalian erythrocyte is unique in that about 90% of its total energy requirement is provided by glycolysis
2. Cornea, lens and some parts of retina which have a limited blood supply and lack mitochondria which if present would absorb and scatter light interfering with transparency.
3. Kidney (medulla), testicles, leukocytes and white muscle fibers, where there are relatively few mitochondria.
4. Contracting muscles due to occlusion of blood vessels by the muscular contraction that decreases oxygen.
Sites
5. Cancer cells due to dissociation of the high rate of glycolysis from Krebs', i.e., aerobic production of lactate
6. Brain and gastrointestinal tract also normally derive most of their energy from glycolysis
- It is oxidation of glucose (or glycogen in muscles) into pyruvate when oxygen is available for respiratory chain or lactate in the absence of oxygen.
- Glucose is transported into cells by glucose transporter (at least 5 types) that is insulin dependent in muscles and adipose tissue but not in other vital tissues, e.g., brain, heart, kidney and RBCs.
- Liver is freely permeable to glucose in and out but insulin activates glucose utilization in liver by activating glucokinase.
1. Activation of glucose:
- Glucose is activated to glucose-6-phosphate by hexokinase or glucokinase, in presence of ATP as coenzyme and magnesium ion as a cofactor, where ATP reacts in the form of Mg+2 (ATP complex.)
- It is irreversible because glucose-6-phosphate is at a higher energy level than glucose and products as a whole are at lower energy level than reactants as a whole.
Only D-hexoses are utilizable.
Glucokinase is in liver parenchymal and pancreatic islet cells, whereas hexokinase is in all extrahepatic cells
2. Glucose-6-phosphate is isomerized into fructose-6-phosphate by phosphohexose isomerase that is an aldose-ketose isomerase. Only the aanomer of glucose-6-phosphate is acted upon.
3. Activation of fructose-6-phosphate by phosphofructokinase into fructose-1, 6-diphosphate, requiring ATP and magnesium as a cofactor.(This is one of the rate limiting irreversible reactions of glycolysis) since fructose-1,6-diphosphate is at a higher energy level than fructose-6phosphate and products as a whole are at lower energy level than reactants as a whole.
4. Cleavage of fructose-1,6-diphosphate into 2 triose phosphates by aldolases: there are several tissue specific aldolase isoenzymes, all of which contain 4 subunits.
1. Aldolase A occurs in most tissues and acts on fructose-1-6diphosphate.
2. Aldolase B occurs in liver and kidney and acts on fructose-1phosphate and fructose-1,6-diphosphate (see fructose metabolism).
5. Interconversion of the two triose phosphates by phosphotriose isomerase enzyme, a reversible reaction. Withdrawal of glyceraldehyde-3phosphate make the equilibrium is in favor of its formation.
6. Glyceraldehyde-3-phosphate (two molecules) is oxidized into 1,3diphosphoglycerate by glyceraldehyde-3-phosphate dehydrogenase, an SH-containing enzyme, in presence of NAD as a coenzyme and inorganic phosphate (Pi).
- glyceraldehyde-3-phosphate dehydrogenase is formed of 4 identical polypeptides, i.e., homotetramer. Four SH groups are present on each polypeptide, derived from cysteine residues within the polypeptide chain. One of these SH groups are found at active site of the enzyme.
- The substrate initially combines with this SH group (E-SH), forming a thiohemiacetal that is converted to a high energy thiol ester by oxidation, the hydrogen removed in this oxidation are transferred to enzyme-bound NAD.
NADH.H+ produced is easily displaced by another molecule of NAD. Phosphorolysis liberates the free enzyme with reconstituted SH group by adding inorganic phosphate (Pi) to form 1,3-diphosphoglycerate. Energy released during the oxidation is conserved by the formation of a highenergy sulfur bond that is replaced by phosphorylation into a high-energy phosphate bond at C1 of 1,3- diphosphoglycerate.
7. Phosphoglycerate kinase converts 1,3-diphosphoglycerate into 3phosphoglycerate and the high-energy phosphate at C1 is utilized to produce an ATP from an ADP. - It is an important reaction and one example of the substrate level phosphorylation, i.e., production of ATP from ADP + Pi utilizing high-energy bond in a substrate directly without intermediates.
8. The phosphate group is moved from C3 to C2 in 3-phophoglycerate by phosphoglycerate mutase. 2,3-bi-phosphoglycerate is an intermediate and/or cofactor in this reaction.
9. Enolase enzyme: in presence of Mg+2or Mn+2 it dehydrates, i.e., removes a water molecule, from 2-phosphoglycerate and redistributes energy within the molecule to form phosphoenol pyruvate which contains a high phosphate energy bond at C2.
10. A second substrate level phosphorylation then occurs, at pyruvate kinase, that utilizes the high-energy bond at C2 of phosphoenol pyruvate to convert an ADP into an ATP. This reaction is irreversible because phosphoenol pyruvate is at a higher energy level than pyruvate.
11. Enol-pyruvate is spontaneously rearranged into pyruvic acid. Under aerobic conditions (presence of oxygen and mitochondria), steps of glycolysis end at this point and pyruvate is oxidatively decarboxylated into acetyl-CoA that enters Krebs' cycle for further oxidation.
12.Under anaerobic conditions, i.e., absence of O2 or mitochondria, NADH.H+ is reoxidized to allow glycolysis to continue by lactate dehydrogenase (LDH) that reversibly reduces pyruvate into lactate.
The isoenzymes of LDH in muscles and RBCs have high affinity for pyruvate, whereas those in liver and heart have high affinity for lactate
Under anaerobic conditions:
1- Total ATP lost = 2 ATP as follows,
One ATP in the activation of glucose to glucose-6phosphate. (STEP 1)
One ATP in the activation of fructose-6-phosphate to fructose1,6-diphosphate. (STEP 3)
2- Total ATP gained = 4 ATP as follows,
2 ATP by substrate level phosphorylation from 1,3diphosphoglycerate (STEP 7)
2 ATP from substrate level phosphorylation from phosphoenol pyruvate. (STEP 10)
3- Net ATP gained = 4 ATP gained - 2 ATP lost = 2 ATP for the anaerobic oxidation of one mole of glucose into lactate.
Under aerobic conditions:
1.Total ATP lost = 2 ATP, as under anaerobic conditions.
2. Total ATP gained = 9 or 7 ATP are generated as follows, 4 ATP (obtained by substrate level phosphorylation) + 2 NADH.H+ Chain (produced from oxidation of glyceraldehyde-3phosphate) = 2 X 1.5 or X 2.5 ATP (according to shuttle) = 3 or, 5 ATP, after oxidation in the functioning respiratory, depending on the type of transporting shuttle used. Thus, 8 or 10 ATP are generated.
3.Net ATP gained = 5, Or, 7 ATP as follows, 9 ATP – 2 ATP = 7, Or, 7 ATP – 2 ATP = 5 ATP for the aerobic oxidation of one mole of glucose.
- The two NADH.H+ produced are oxidized by respiratory chain producing 3 or 5 molecules of ATP depending on the type of shuttle used to transfer H2 equivalents to mitochondria, since mitochondria membrane is impermeable to NADH.H+
- Both cytoplasmic and mitochondrial malate dehydrogenases are NAD dependent. Thus, glycolysis gives 2 cytoplasmic NADH.H+ = 2 mitochondrial NADH.H+ = 2 X 2.5ATP = 5 ATP.
- Thus, glycolysis gives 2 cytoplasmic NADH.H+ = 2 mitochondrial FADH2 = 2 X 1.5 ATP = 3 ATP.
- Regulation of any biochemical cascade targets the key regulatory enzymes of it. Key regulatory enzymes are those enzymes that catalyze the irreversible steps of glycolysis that include three steps as follows,
I. Phosphofructokinase (STEP 3 ) : The most important site of control because fructose-1,6-diphosphate is unique to glycolysis. It is an allosteric enzyme stimulated by high levels of fructose-6- phosphate, fructose-2,6diphosphate (in liver), ADP and AMP, Pi, and ammonia. It is inhibited allosterically by ATP, low pH and citrate.
II. Hexokinase (STEP 1) : Accumulation of glucose-6-phosphate and inhibition of phosphofructokinase results in accumulation of fructose-6-phosphate and glucose-6-phosphate that allosterically inhibit hexokinase. However, phosphofructokinase is more important than hexokinase since glucose-6phosphate is an intermediate for other cycles, e.g., uronic acid pathway, glycogenesis and HMP shunt, while fructose-1,6-diphosphate is unique to glycolysis, so it is key regulatory for glycolysis only.
III. Pyruvate kinase (STEP 10) : It is inhibited also by excess ATP, alanine, fatty acids, and acetyl-CoA and is stimulated by fructose-1,6-diphosphate, ADP and AMP and is inducible by high carbohydrate intake and insulin. It is regulated by cAMP-dependent phosphorylation-dephosphorylation mechanism
1. Insulin: stimulates synthesis of glucokinase, phosphofructokinase and pyruvate kinase, so it stimulates glycolysis. It also induces glucose transporters to provide cells with glucose for glycolysis.
2. Adrenaline and glucagon are inhibitory by inhibiting pyruvate kinase.
A. 2-Deoxyglucose inhibits hexokinase and glucokinase
B Iodoacetate, a SH poison inhibits glyceraldehyde-3-phosphate dehydrogenase which contains this group at its active site.
C. Fluoride inhibits enolase. So, on accurately measuring blood glucose levels, the samples should be fluorinated to stop glycolysis in RBCs.
D. Arsenate closely resembles inorganic phosphate and replaces it in the reaction catalyzed by glyceraldehyde-3-phosphate dehydrogenase producing 1-arseno-3-phosphoglycerate, which is unstable. This compound rapidly decomposes to give 3-phosphoglycerate plus heat
This means that glycolysis continues in the presence of arsenate, but producing one ATP less. Thus, arsenate uncouples oxidation and phosphorylation (ATP synthesis) at substrate level.
✔ Biological importance and diseases glycolysis
✔ Rapoport-Lubering cycle and Biochemical significance of 2,3-Diphosphoglycerate
✔ Oxidative Decarboxylation of Pyruvate Steps and Regulation
Glucose oxidation producing ATP. It is the major source of energy in certain tissues, e.g., RBCs and skeletal muscles.
Reversal of glycolysis is gluconeogenesis, an important source of glucose
It is the major source of lactic acid that is gluconeogenic
Production of 2,3-DPG that is important in tissue oxygenation.
It provides pyruvic acid needed for Krebs' cycle.
A small number of genetic diseases occur due to deficiency in activity of enzymes of glycolysis, are manifested mainly as hemolytic anemias.
It a link with;
from the diet.
Reversal of glycolysis is gluconeogenesis, an important source of glucose
• fat metabolism, e.g., dihydroxyacetone phosphate into glycerol 3-phosphate in adipose tissue.
• amino acid metabolism, e.g., 3-phopshoglycerate into serine and pyruvate into alanine and vice versa.
Blood lactate comes from glycolysis in RBCs and muscles. It is taken up by the heart to be oxidized into energy in Krebs' cycle, by liver and kidney to be converted into glucose, by liver to be converted into fatty acids, or excreted as sodium lactate in sweat and urine. This depletes the blood alkali reserve leading to hyperlactemia and lactic acidosis if blood lactate exceeds it normal level, i.e., reaching more than 5 mM (normal level is 0.44 - 1.8 mM/L). Uncontrolled lactic acidosis could cause coma.
Sever exhaustive muscle exercise that increases lactate production (fatigue is caused in part by lactate).
Liver diseases that decrease lactate utilization.
Hypoglycemic antidiabetic drugs that increase lactate production by anaerobic oxidation of glucose.
Anoxia that increases lactate production and decreases its utilization. As in shock and diseases that impair blood flow, respiratory disorders, and severe anemia
This is due to genetic reduction of pyruvate kinase activity to 5% - 25% of normal level. This causes decreased rate of glycolysis and leads to deficiency of ATP produced in RBCs. RBCs can not control its size, swell and lysis occurs (hemolysis) due to the stoppage of the ATP-dependent membrane pumps. - 2,3-DPG level is increased in this condition.
Genetic deficiency of hexokinase causes decreased rate of glycolysis and low 2,3-DPG level leading to high hemoglobin affinity to O2 that will be less available for tissues.
RBCs depend on glycolysis as a main source of energy because they lack mitochondria. However, the energy produced anaerobically by glycolysis far exceeds the need of RBCs (because RBCS lack many metabolic reactions that require energy), so these cells must dissipate energy by an important cycle called Rapoport-Lubering cycle.
Rapoport-Lubering cycle is a side-pathway (a shunt) from glycolysis that is formed of two steps:
• 1,3-diphosphoglycerate is mutated into 2,3-diphosphoglycerate (2,3-DPG) transfer of phosphate from C1 to C2 results in transformation of phosphate from high to low energy bond. 2,3-DPG is an important compound.
• 2,3-diphosphoglycerate phosphatase to 3-phosphoglycerate activated by phosphoglycerate mutase
the phosphate group at C2 of 2,3-DPG is hydrolyzed producing 3phosphoglycerate. 3-phosphoglycerate rejoins the ordinary pathway of glycolysis.
significance of 2,3-DPG
• 2,3-DPG acts as a cofactor in the conversion of 3-phosphoglycerate to 2phosphoglycerate by mutase
• It decreases the affinity of hemoglobin (Hb) to oxygen helping oxygen dissociation and unloads oxygen in tissue capillaries for oxygenation. Therefore, the levels of 2,3-DPG increases markedly in peripheral hypoxic tissues and hypoxic conditions (high altitudes, hypoxic hypoxia, stagnant hypoxia and anemic hypoxia)
Fetal hemoglobin binds 2,3-DPG less strongly than adult hemoglobin and therefore has a higher O2 affinity to extract O2 from mother's blood.
Red blood cells have a high concentration of 2,3-DPG (4 - 5 mM, equimolar to Hb) in contrast to traces amount in most other cells. During storage of blood in blood banks, 2, 3-DPG concentration decreases gradually to reach traces at ten days. So, Hb of this blood has a high affinity to O2 and is not suitable for blood transfusion to hypoxic patients suffering from, e.g., respiratory disorders and severely ill patients. RBCs membrane is impermeable to 2,3-DPG. So, instead of adding 2,3-DPG, inosine is added which penetrates RBCs easily and its ribose is changed very slowly into 2,3-DPG by HMP shunt and glycolysis.
steps
It is a preparatory phase for Krebs' cycle. Pyruvate passes into the mitochondria by the help of a special transporter on inner mitochondrial membrane. Oxidative decarboxylation is removal of CO2 and hydrogen from pyruvate to give acetyl-CoA (i.e., oxidation and decarboxylation) catalyzed by an enzyme complex called pyruvate dehydrogenase which is composed of three enzymes:
• Pyruvate dehydrogenase component
• Dihydrolipoyl transacetylase, DHLTA
• Dihydrolipoyl dehydrogenase, DHLD Pyruvate dehydrogenase utilizes 5 coenzymes:
• Thiamin pyrophosphate (TPP) • Lipoic acid • Coenzyme A (CoASH) • Flavin adenine dinucleotide (FAD)
• Nicotinamide adenine dinucleotide (NAD)
• Pyruvate first binds with TPP and then undergoes decarboxylation by the help of pyruvate dehydrogenase part of the enzyme complex.
• Oxidized lipoic acid then reacts with active acetaldehyde to release TPP and form S-acetyl lipoate. This reaction is also catalyzed by dihydrolipoyl transacetylase component of the enzyme complex.
• S-acetyl lipoic acid reacts with coenzyme A to form acetyl-CoA and reduced lipoate catalyzed by dihydrolipoyl-transacetylase of the complex.
• Reduced lipoic acid is oxidized by dihydrolipoate dehydrogenase using FAD as a coenzyme.
• The reduced FAD is oxidized by dihydrolipoyl dehydrogenase giving its 2 hydrogen to NAD.
Pyruvate dehydrogenase has 2 forms:
• Inactive form (phosphorylated)
• Active form (Dephosphorylated)
Pyruvate dehydrogenase is susceptible to regulation by covalent modification by phosphorylation-dephosphorylation mechanism. Activation of the enzyme (dephosphorylation) occurs by the action of a phosphatase in a reaction requiring Ca+2 , Mg+2 and probably insulin. Protein kinase inactivates the active enzyme by phosphorylation.
Arsenate and deficiency of vitamin B1 (Beri-Beri) inhibit the enzyme. The enzyme is allosterically inhibited by accumulation of:
• acetyl-CoA
• NADH.H+
• ATP
The enzyme is allosterically stimulated by pyruvate Thus, pyruvate dehydrogenase and glycolysis is inhibited by high-energy potential, e.g., fatty acid oxidation that produces large amount of energy.
✔ Definition of Krebs' cycle
✔ Steps and Biological importance of Krebs' cycle
✔ Regulation of Krebs' cycle
• It is the cycle by which active acetate (acetyl-CoA) produced from pyruvate, ketogenic amino acids or oxidation of fatty acids is completely oxidized into CO2, FADH2, and NADH.H+
• Hydrogen of these coenzymes is converted into H2O by the respiratory chain, see Biological Oxidation. Thus, Krebs' cycle is a common pathway for oxidation of all nutrients (carbohydrate, lipids and proteins).
• It occurs in the mitochondria where the enzymes required are located free in the matrix or attached to the inner mitochondrial membrane.
• This makes enzymes of the Krebs' cycle in close proximity to the enzymes of the respiratory chain to facilitate the transfer of reducing equivalents
• by condensation of oxaloacetate with active acetate irreversibly catalyzed by citrate synthase, which condenses carbon-to-carbon binding of the methyl carbon of acetyl-CoA and the carbonyl carbon of oxaloacetate.
- Hydrolysis of the thioester bond in acetyl-CoA intermediate compound is accompanied by considerable loss of free energy as heat, ensuring irreversibility for the reaction.
Step2 : Reversible conversion of citrate into isocitrate
• by aconitase enzyme, water is removed from citric acid producing cisaconitic acid. Aconitase then adds water again to produce isocitrate. It has dual specificity, acting on 2 different substrates, i.e., citrate and cis-aconitate catalyzing the same reaction.
- Ferrous iron is required, where the enzyme contains iron sulfur protein.
Step3 : Oxidative decarboxylation of citrate into α-ketoglutarate
• by isocitrate dehydrogenase which is an enzyme with dual specificity acting on the same substrate (isocitrate) catalyzing 2 different reactions:
Dehydrogenation (oxidation), removing 2 hydrogen from isocitrate giving them to NAD producing NADH.H+ and oxalosuccinate. Decarboxylation of oxalosuccinate to produce α-ketoglutarate, in a reaction requiring Mn+2
• There are 3 different species of isocitrate dehydrogenase:
1- NAD-dependent mitochondrial isocitrate dehydrogenase. 2- NADP-dependent mitochondrial isocitrate dehydrogenase 3- NADP-dependent cytoplasmic isocitrate dehydrogenase
Step4 : Oxidative decarboxylation of α-Ketoglutarate into succinyl-CoA
• It is an irreversible reaction similar to oxidative decarboxylation of pyruvate but utilizing α-ketoglutarate dehydrogenase enzyme complex that have 3 enzymes
Step5 : Succinyl-CoA is converted into succinate
• It is a substrate level phosphorylation reaction in Krebs' cycle.
- Succinyl thiokinase utilizes the high carbon sulfur bond to generate GTP from GDP and Pi. The GTP is then used to generate ATP from ADP and Pi.
Step6 : Succinic acid is oxidized into fumarate
• by succinic acid dehydrogenase in a reversible reaction in presence of
FAD
- This is the only enzyme in Krebs' cycle that is bound to the inner mitochondrial membrane surface unlike the other enzymes, which are soluble in the matrix.
Step7 : Fumaric acid is hydrated into malate by fumarase
• Fumarase catalyzes the reversible addition of the elements of water to the double bond of fumarate to produce malate.
Step8 : Malic acid is oxidized into oxaloacetate
• Malate dehydrogenase reversibly catalyzes the reaction in presence of NAD. The equilibrium of the reaction favors malate formation, but in absence of energy (accumulation of NAD) the net flux is toward the direction of oxaloacetate because oxaloacetate and NADH.H+ are removed continuously in further reactions.
Thus, the cycle started with acetyl-CoA and oxaloacetate and ended with oxaloacetate while acetyl-CoA is oxidized into 2 CO2 and hydrogen (as NADH.H+ and FADH2) that is oxidized by respiratory chain into H2O.
Moreover : It should be noticed that enzymes similar to the enzymes of the citric acid cycle are also found outside the mitochondria except for α- ketoglutarate dehydrogenase. While they may catalyze similar reactions, some of the enzymes, e.g., malate dehydrogenase, may not be in fact the same proteins as the mitochondrial enzymes of the same name.
It provides intermediates for synthesis of non-essential amino acids, e.g,:α-Ketoglutarate can give rise to glutamic acid by transamination.
Oxaloacetate can give rise to asparatic acid by transamination.
5. It is an amphipathic pathway for gluconeogenesis, transamination, deamination and lipogenesis particularly in the liver.
• Oxidative decarboxylation of the two mole of pyruvate produced from one mole of glucose gives 2 NADH.H+ that gives 5 ATP.
• Oxidation of isocitrate by isocitrate dehydrogenase gives 2 NADH.H+ that gives 5 ATP.
• Oxidative decarboxylation of α-ketoglutarate to succinyl-CoA gives 2NADH.H+ that gives 5 ATP.
• Substrate level phosphorylation from succinyl-CoA gives 2 ATP.
• Oxidation of succinate to fumarate gives 2 FADH2, thus 3 ATP.
• Oxidation of malate to oxaloacetate gives 2 NADH.H+ , i.e., 5 ATP.
Thus, for each mole of glucose oxidized by oxidative decarboxylation followed by Krebs' cycle 32 ATP are produced.
• Complete oxidation of one glucose molecule in aerobic conditions gives 9 ATP at aerobic glycolysis + (3+20) ATP at Krebs' cycle giving a total of 32 ATP.
• It is difficult to identify regulatory enzymes of citric acid due to the presence of many pathways with which the cycle interacts.
- Regulation occurs at the following sites:
1. Citrate synthase: it is an allosteric enzyme inhibited by ATP and long chain
fatty acyl-CoA. It is competitively inhibited by succinyl-CoA.
2. Isocitrate dehydrogenase: The enzyme is allosterically activated by ADP and NAD and inhibited by ATP and NADH.H+.
3. α-Ketoglutarate dehydrogenase: The enzyme is regulated by phosphorylation/dephosphorylation mechanism in a manner similar to pyruvate dehydrogenase. This enzyme is inhibited by accumulation of ATP, succinyl-CoA and NADH.H+.
4. Succinate dehydrogenase is inhibited by oxaloacetate and the availability of oxaloacetate as controlled by malate dehydrogenase depends on the NADH/NAD ratio.
Respiratory chain itself exerts an overall control through oxidative phosphorylation being inhibited by excess ATP/ADP ratio. This ensures oxidation of reduced coenzymes.
In vitro inhibitors of Krebs' cycle
1. Fluoroacetate: Inhibits aconitase enzyme. This compound in the form of fluoroacetyl-CoA condenses with oxaloacetate to form fluorocitrate that inhibits aconitase leading to accumulation of citrate.
2. Arsenate: inhibits both pyruvate and α-ketoglutarate dehydrogenases.
3. Malonate or oxaloacetate: Inhibits succinate dehydrogenase enzyme (competitive inhibition).
4. Mercury : inhibits succinate dehydrogenase.
sources
1. Carboxylation of pyruvate (see gluconeogenesis).
2. Oxidation of malate (Krebs'). 3. Cleavage of citrate (see lipogenesis).
4. Transamination of aspartate (see protein metabolism).
1. Reduction into malate (Krebs' during gluconeogenesis).
2. Synthesis of citrate (Krebs').
3. Transamination into aspartate (see protein metabolism).
4. Conversion into phosphoenolpyruvate (see gluconeogenesis).
✔ Understand the definition Redox Potentials.
✔ Know the component of Electron Transport Chain.
✔ Be able to define the mechanism of the oxidative phosphorylation.
Electrons carried by reduced coenzymes (NADH or FADH2) are passed sequentially through a chain of proteins and coenzymes (electron transport chain) to O2.
(for your information) It is a series of protein complexes and other molecules that transfer electrons from electron donors to electron acceptors via redox reactions and couples this electron transfer with the transfer of protons across a membrane.
Biological oxidations are catalyzed by intracellular enzymes, to obtain energy.
(FYI) It is the combination of oxidation-reduction transformations of substances in living organisms.
Coupling e- transport, oxidation and ATP synthesis (Phosphorylation). Site of oxidative phosphorylation: inner mitochondrial membrane (Eukaryotic cells).
(FYI) oxidative phosphorylation is a cellular process that harnesses the reduction of oxygen to generate high-energy phosphate bonds in the form of adenosine triphosphate (ATP).
Can be expressed in terms of Redox potential or oxidation –reduction potential which is a quantitative measure of the tendency of redox pair to lose or gain electrons.
Free energy change is proportionate to the tendency of reactants to donate or accept the electrons. ( ve) redox potential. (+ve) redox potential.
- Arranged in the order of increasing redox potential - From electro (-ve) to electro (+ve)
Complex I Complex II
1. NADH-ubiquinone oxidoreductase (NADH dehydrogenase).
2. Embedded in mitochondrial membrane.
3. Transfers electrons from NADH to Q.
4. NADH transfers two electrons as a hydride ion (H: H:-) to FMN.
5. Electrons, one at a time, are passed through Complex I to Q via FMN and iron-sulfur proteins.
1. Succinate-ubiquinone oxidoreductase (or succinate dehydrogenase complex).
2. Accepts electrons from succinate and catalyzes the reduction of Q to QH 2.
3. FAD of II is reduced in a 2electron transfer of a hydride ion from succinate.
4. Complex II does not contribute to proton gradient, but supplies electrons from succinate.
• Exist in oxidized (Fe³⁺) & reduced (Fe²⁺) state.
• Transfers electrons FMNH₂, Q and Q b.
Iron sulfur centers (clusters)
• Iron-sulfur centers (Fe-S) have prosthetic groups containing 1-4 iron atoms
• They transfer only 1 electron, even if they contain 2 or more iron atoms.
• E.g., a 4-Fe center might cycle between redox states:
• Fe+++, Fe++1 (oxidized) + 1 e- Fe+++1, Fe++ (reduced).
i. Coenzyme Q (Co Q, Q or ubiquinone) is lipid-soluble. It dissolves in the hydrocarbon core of a membrane.
ii. The only electron carrier not bound to a protein. It is a mobile electron carrier.
iii. It has ability to accept electrons in pairs and pass them one at a time through a semiquinone intermediate to complex III.
iv. This is called “Q cycle”.
Cytochrome c is a small, water-soluble protein.
Cytochrome c is also a mobile electron carrier.
Cytochromes are electron carriers containing heme, heme in the 3 classes of cytochrome (a,b,c) differ in substituents on the porphyrin ring.
Some cytochromes (b, c1, a, a3) are part of large integral membrane protein complexes.
✔ Be able to define Free Radicals
✔ Understand the environment and the causes.
✔ Know the harmful effects of free radicals
✔ Get to know the concept and the phases of lipid peroxidation.
✔ Study the topic of Antioxidants.
✔ Know some important examples of antioxidant enzymes.
Before we get started we should know some important points… What’s the definition of free radicals and what’s their classification?
Definition of free radicals:
# A free radical can be defined as any molecular species capable of independent existence that contains an unpaired electron in an atomic orbital.
# A type of unstable molecule that is made during normal cell metabolism (chemical changes that take place in a cell).
Classification of free radical can be as followed: (the classification is just FYI)
1. Extreme reactivity.
2. Short life span.
3. Generation of new ROS by chain reaction.
4. Damage to various tissues
Environmental effects:
-Result of drug metabolism.
-Cytochrome P450 related reactions.
-Due to damage caused by ionizing radiations on tissues (X-rays) Photolysis of O2 by light
Photoexcitation of organic molecules.
-Cigarette, smoke and alcohol promote lipid peroxidation.
So what is “lipid peroxidation”?
H2O2 & singlet oxygen are not free radicals
Because of their extreme reactivity, they are included in the group of ROS.
ROS means reactive oxygen species
Lipid peroxidation occurs in 3 stages- initiation, propagation & termination.
1 2
This step involves the removal of hydrogen atom (H) from polyunsaturated fatty acids (LH), caused by hydroxyl radical.
LH + OH- → L- + H2O
form peroxy radical (LOO-).
The latter, in turn, can remove H-atom from another PUFA (LH) to form lipid hydroperoxide (LOOH).
Under aerobic conditions, the fatty acid radical (L-) takes up oxygen to LH + OH- L- + H2O
LOO- + LH → LOOH + L-
The hydroperoxides are capable of further stimulating lipid peroxidation as they can form alkoxy (LO-) & peroxyl (LOO-) radicals.
2LOOH - → LO- + LO2- + H2O
LOOH → LO- + LOO- + aldehydes
Lipid peroxidation proceeds as a chain reaction until the PUFA gets oxidized.
this figure shows examples of the enviromental effects
Free radicals cause oxidation of sulfhydryl groups & modification of certain amino acids (e.g. methionine, cysteine, histidine, tryptophan).
PUFA are susceptible to damage by free radicals.
Carbohydrates:
Lipids: Nucleic acid:
Oxidation of monosaccharides (e .g. glucose) can produce H2O2 & oxoaldehyde.
Glycation increases the susceptibility of proteins to the attack by free radicals. e.g. DM.
Free radicals may cause DNA strand breaks, fragmentation of bases & deoxyribose.
Antioxidants in relation to lipid peroxidation:
Preventive antioxidants that will block the initial production of free radicals e.g. catalase, glutathione peroxidase. Chain breaking antioxidants that inhibit the propagative phase of lipid peroxidation e.g. Superoxide dismutase, vitamin E, uric acid.
According to location:
1. Plasma antioxidants: [e.g. β-carotene, ascorbic acid, bilirubin, uric acid, ceruloplasmin, transferrin].
2. Cell membrane antioxidants: [α- tocopherol].
3. Intracellular antioxidants: [SOD, catalase, glutathione peroxidase].
According to nature & action: Enzymatic antioxidants: [SOD, catalase, glutathione peroxidase & glutathione reductase].
Non-enzymatic antioxidants:
1)Nutrient: carotenoids, α-tocopherol, selenium & vitamin C.
2)Metabolic: glutathione, ceruloplasmin, albumin, bilirubin, transferrin, ferritin, uric acid.
Superoxide Dimutase: 1
It converts superoxide (O2) to hydrogen peroxide & O2.
This is the first line of defense to protect cells from the injurious effects of superoxide
Catalase: 2
H2O2 is metabolized by catalase.
Glutathione Peroxidase: 3
It detoxifies H2O2 to H2O, while reduced glutathione (G-SH) is converted to oxidized glutathione (GS-SG).
The reduced glutathione can be regenerated by glutathione reductase utilizing NADPH.
HMP shunt is the major source of NADPH.
✔ Glycogen Storage Diseases (glycogenosis)
• The body obtains glucose either directly from the diet or from amino acids and lactate via gluconeogenesis
• Glucose obtained from these two primary sources either remains soluble in the body fluids or is stored as glycogen.
• Glycogen is considered the principal storage form of glucose and is found mainly in liver (10% of its weight is glycogen).
• Other tissues such as skeletal muscle, brain, kidney, heart, and adipose tissue are capable of glycogen synthesis and breakdown. Muscle has a much lower amount of glycogen per unit mass of tissue, but since the total mass of muscle is so much greater than that of liver, total glycogen stored in muscle is about twice that of liver.
1. Glucose is phosphorylated to glucose-6phosphate by hexokinase or glucokinase.
2. Glucose-6-phosphate is then converted to glucose-1-phosphate by phosphoglucomutase.
3. Glucose-1-phosphate is converted to the active nucleotide UDP-glucose by reaction with UTP catalyzed by UDPglucose pyrophosphorylase.
4. Glycogen synthase connects C1 of UDPglucose with C4 of a terminal glucose residue in a pre-existing glycogen chain (glycogen primer, formed of a few molecules of glucose linked by 1,4glucosidic linkage).
5. When the glucose units added to the glycogen primer reach a minimum straight chain length of 11 units, a branching enzyme (1,4 1,6transglucosidase) transfers a block of 6 - 7 glucose residues minimum length and connects it into the side of a neighboring chain by 1,6-linkage.
The branch point should be at least 4 residues from the previous branch point in the same chain. The branches grow by further additions of -1,4-glucosyl units and further branching occurs.
✓ Glycogen synthase enzyme exists in two forms:
1- Glycogen synthase A
2- Glycogen synthase B.
✓ Glycogen synthase a is an active form of enzyme while Glycogen synthase b is normally inactive form of enzyme. Glycogen synthase b is converted into Glycogen synthase a by the process of dephosphorylation. It is catalysed by Protein phosphatase.
✓ Active Glycogen synthase, i.e. dephosphorylated glycogen synthase, is converted back into inactive phosphorylated glycogen synthase by the process of phosphorylation. It is catalyzed by enzyme Protein kinase A.
✓ Initially there no glycogen primer to elongate by the synthase.
✓ A protein called glycogenin adds UDP-glucose at C4 onto an OH group of one of its tyrosine residue.
✓ This is a self-glycosylation reaction or synthase catalyzed (particularly after addition of 8-glucose molecules in a linear manner).
✓ Glycogenin may stay attached (muscles) or get partially hydrolyzed (liver).
Definition:
It is the process of glycogen catabolism into:
Glucose to blood, in the liver.
Or
Glucose-6-phosphate Lactic acid, in the muscles.
Site: Cytoplasm
Steps:
I. Glycogen phosphorylase hydrolyzes the terminal -1,4-glucosidic bond of a linear branch of glycogen molecule by addition of inorganic phosphate (Pi, i.e., phosphorolysis). This results in release of glucose-1-phosphate. Pyridoxal phosphate is a coenzyme for this reaction. Phosphorylase continues acting on the 1,4-glucosidic linkage of linear branch and stops working when there are only 4 glucosyl units remaining from the branch point (-1,6).
II. The released glucose-1-phosphate is transformed into glucose-6-phosphate by the reversible action of phosphoglucomutase. In the liver, glucose-6phosphate is dephosphorylated by glucose-6-phosphatase into glucose that is transported into blood. In muscles, there is no glucose-6-phosphatase and glucose-6-phosphate enters glycolysis to produce lactate.
III. Glucan transferase It transfers 3 out of these 4 glucose units from the branch to another branch to be in linear sequence with it, i.e., by -1,4-glucosidic linkage. This exposes the last glucose unit (-1,6-linked at branching point).
IV. Debranching enzyme (-1,6-glucosidase) removes that last glucose unit by hydrolysis as free non-phosphorylated glucose that diffuses into blood.
Glycogen homeostasis involves the concerted regulation of the rate of glycogen synthesis (glycogenesis) and the rate of glycogen breakdown (glycogenolysis). Theses two processes are reciprocally regulated such that hormones that stimulate glycogenolysis (e.g. glucagon, cortisol, epinephrine, norepinephrine) simultaneously inhibit glycogenesis. Conversely, insulin, which directs the body to store excess carbon for future use, stimulates glycogenesis while simultaneously inhibiting glycogenolysis.
Definition:
They are groups of inherited disorders characterized by deposition (overstorage) of an abnormal type or quantity of glycogen or failure of storage of glycogen in the tissues due to deficiency of one of enzymes of glycogenesis or glycogenolysis, phosphofructokinase or lysosomal glycosidases
Types:
Type I : Von Gierke’s disease
It is the commonest type of this group. It is due to deficiency of glucose6-phosphatase in liver and kidney. It is characterized by:
1. Accumulation of large amounts of glycogen in liver and kidney causing disturbance in liver functions.
2. Enlargement of liver and kidney.
3. Fasting hypoglycemia associated with ketosis and hyperlipidemia.
4. Hyperuricemia: Deficiency of glucose-6-phosphatase leads to accumulation of glucose-6-phosphate that is utilized through HMP shunt to give large amount of pentose-5-phosphate that enters in purine synthesis that is catabolized into large amount of uric acid, i.e., hyperuricemia.
5. Lactic acidosis and failure to thrive.
(Acid Maltase Deficiency) Acid maltase is a lysosomal α-1,4-glucosidase that releases glucose from glycogen, oligosaccharides and maltose. Acid maltase deficiency (AMD) is separated into three major groups: infantile, childhood, and adult. All types are transmitted by autosomal recessive inheritance.
It is characterized by deficiency of debranching enzyme in muscle and liver, which causes the accumulation of glycogen with many short branches. Clinically, it is like type I but milder.
It is due to deficiency of branching enzyme in liver and spleen. Glycogen formed has very long branches. Death due to cirrhosis and liver failure occurs before age 2.
It is due to deficiency of muscle glycogen phosphorylase. Glycogen is accumulated in the skeletal muscles in an abnormally high content (2.54.1%). As its breakdown doesn’t occur during exercise, inadequate energy supply and muscle cramps and damage occurs
It is the formation of glucose from non-carbohydrate precursors. It is particularly important for tissues dependent on blood glucose such as RBCs and brain. The daily glucose requirements of the adult brain is 120 grams, whereas, the whole body requires 160 grams. The body stores are 210 grams (190 grams from liver glycogen and 20 grams in body fluids) enough for a day. In a longer period of starvation or during intense exercise, glucose must be formed from noncarbohydrate sources for survival.
These non-carbohydrate precursors include lactate, pyruvate, propionate, glycerol (from diet and lipolysis) and glucogenic amino acids.
Mitochondria and cytosol of Liver and kidney are almost the only organs able to synthesize glucose from non-carbohydrate sources.
The liver being the largest organ in the body, exceeding the combined weights of the kidneys and thus contributes more in maintaining blood glucose levels by gluconeogenesis.
The liver is unique in that it has the capacity to convert three carbon precursors (such as lactate, glycerol, pyruvate and propionate) along with glucogenic amino acids into glucose.
Gluconeogenesis is very limited in:
• Skeletal muscle due to deficiency of glucose-6-phosphatase.
• Heart and smooth muscles and adipocytes due to deficiency of fructose 1,6-diphosphatase.
A. There is always a basal requirement of glucose even if fatty acid oxidation is supplying enough energy in the tissues.
Gluconeogenesis supplies body cells with glucose after 4 - 6 hours of last meal and becomes fully active as stores of glycogen are depleted. Glucose produced by gluconeogenesis has the following functions:
1. It is used to meet the needs for glucose by other cells of the body, especially tissues dependent upon blood glucose as the only source of energy. These tissues include brain and nervous system, RBCs, renal medulla, Lens, cornea and some regions of the retina, white and red skeletal muscles under anaerobic conditions, testes and leukocytes.
2. Glucose is especially needed for adipose tissue as a precursor of glycerol (glycerokinase is absent) and mammary gland as a precursor of lactose.
3. Glucose produces oxaloacetate (from pyruvate) and other intermediates of citric acid cycle in many tissues.
B. Besides its role in glucose production, gluconeogenesis is the way by which lactate produced during muscular contraction and in RBCs is converted into glucose.
It is essentially the reverse of glycolysis, except at the three irreversible reactions that different enzyme(s) to be reversed.
Cori's Cycle is the cycle illustrating the fate of lactic acid produced by active muscles and RBCs into glucose.
▪
Lactate and/or pyruvate formed by the anaerobic oxidation of glucose from skeletal muscle glycogen or glycolysis in RBCs, diffuse to blood stream and is transported to the liver and the kidney where it is transformed into glucose by gluconeogenesis.
▪
Pyruvate also leaves muscles as alanine after transamination particularly during long starvation, where muscle proteins break down. Alanine goes to liver where transamination converts it back into pyruvate.
1. Conversion into glucose during starvation.
2. Conversion into pyruvate and joins Krebs' of heart and muscles (20%).
3. Small amounts are excreted in sweat and urine as sodium lactate.
The glucose formed diffuses back to the blood to be used by various tissues.
1. Conversion into pyruvate is reversibly activated by lactate dehydrogenase.
2. Pyruvate enters mitochondria by pyruvate transporter to be irreversibly carboxylated into oxaloacetate by pyruvate carboxylase. Oxaloacetate leaves mitochondria by several ways.
3. In cytoplasm, oxaloacetate is converted irreversibly into phosphoenol pyruvate and CO2 by phosphoenol pyruvate carboxykinase in the presence of GTP as a source of phosphate and Mg+2 .
4. Phosphoenol pyruvate takes opposite direction of glycolysis to glucose activated by fructose1,6-diphosphatase and glucose-6-phosphatase.
1. Conversion of Pyruvate to phosphoenol pyruvate activated by pyruvate kinase is reversed by the combined action of two enzymes:
a. Pyruvate carboxylase in mitochondria requires CO2, ATP, biotin as a coenzyme and Mn2+ as a cofactor. The reaction is activated by accumulation of acetyl-CoA (and ATP), a marker of excess acetyl-CoA that needs oxaloacetate to enter the citric acid cycle and/or a marker of no need for energy and thus favors gluconeogenesis.
b. Phosphoenol pyruvate carboxykinase in the cytoplasm. Cytoplasmic oxaloacetate is converted into phosphoenol pyruvate by pyruvate carboxykinase utilizing GTP as phosphate donor and releasing H2O and CO2.
2. Conversion of Fructose-1,6-diphosphate into fructose-6- phosphate activated by phosphofructokinase is reversed by fructose-1,6diphosphatase. Then, Fructose-6-phosphate is isomerized to glucose-6phosphate by the reversible action of phosphohexose isomerase.
3. Conversion of Glucose-6-phosphate into glucose and phosphate is activated by glucose-6-phosphatase. Glucose is transported (Glut2 transporter) from liver into blood. In muscles, glucose-6-phosphatase is absent, and glucose-6-phosphate stays there to be used in glycolysis.
❖ Since oxaloacetate can't traverse the mitochondrial membrane, it leaves the mitochondria in the form of:
1. Malate: Oxaloacetate is reduced reversibly to malate by the mitochondrial malate dehydrogenase Malate then leaves mitochondria to be oxidized back to oxaloacetate by the cytoplasmic enzyme
2. Aspartate: mitochondrial glutamic oxaloacetic transaminase (GOT) converts oxaloacetate into aspartate, which passes to the cytoplasm to be converted back by the cytoplasmic GOT into oxaloacetate.
3. Citrate: Citrate synthase converts oxaloacetate into citrate that leaves mitochondria (in exchange with malate) where it is cleaved by citrate lyase in cytoplasm into oxaloacetate and acetyl-CoA
• Glycerol absorbed from diet or derived from lipolysis of fat is activated by glycerol kinase in liver, kidney, lactating mammary gland, heart and intestine into glyerol-3- phosphate.
• Glyerol-3-phosphate dehydrogenase oxidizes glyerol-3-phosphate into dihydroxyacetone-phosphate to cross glycolysis into glucose.
• Propionic acid is derived from, methionine, isoleucine, -oxidation of odd number fatty acid, and large intestinal and ruminal fermentation of fibers. Oxidation of odd number chain fatty acids ends with propionyl-CoA. Methionine catabolism gives rise to propionyl-CoA.
• Microfloral fermentation of dietary fibers in human large intestine and rumen of ruminants produces volatile fatty acids (propionic, acetic and butyric acids). Propionic acid is the major source of glucose synthesized by gluconeogenesis in ruminants.
Glucogenic amino acids from the diet or from tissue proteins during starvation are transformed to glycolytic or Krebs' intermediates either by transamination or deamination. Examples are glutamate that gives - ketoglutarate, aspartate that
gives oxaloacetate and alanine that gives pyruvate by transamination. Serine, threonine, and cysteine give pyruvate and proline, arginine, histidine, lysine and glutamate give -ketoglutarate by deamination
1. The key regulatory enzymes: Glucose-6-phosphatase, fructose-1,6diphosphatase, phosphoenolpyruvate carboxykinase, and pyruvate carboxylase.
2. Hormones: glucocorticoids, adrenaline and glucagon are released in prolonged fasting and starvation. They induce synthesis of the key regulatory enzymes. Glucagon increases gluconeogenesis from lactate and amino acids. Glucocorticoids increase protein catabolism and activity of transaminases. Adrenaline increases glycerol availability from lipolysis.
3. Allosteric effectors: excess ATP, citrate, acetyl-CoA and NADH.H+ stimulate, whereas, ADP and AMP inhibit the key regulatory enzymes.
❖ Conditions characterized by active gluconeogenesis: Severe muscular exercises (more lactate), Cushing's syndrome and high doses of cortisone or ACTH (more cortisol), diabetes mellitus (no insulin).
✓ Alcohols . ✓ Glycerol definition and uses . ✓ Fatty acid classification . ✓ Phospholipids .
⚫ Lipids are organic compounds formed mainly from alcohol and fatty acids combined together by ester linkage.
Lipids = Alcohol + Fatty acids (by ester linkage).
⚫ Lipids are insoluble in water, but soluble in fat or organic solvents (ether, chloroform, benzene, acetone, etc.). Lipids include fats, oils, waxes and related compounds.
⚫ They are widely distributed in nature both in plants and in animals.
Lipid-associating substances: They include fat-soluble vitamins (E and K), carotenoids, squalene and hydrocarbons.
Alcohols associated with lipids include: glycerol, cholesterol and higher alcohols (e.g. cetyl alcohol, mericyl alcohol, dolicols……….etc.).
Trihydric alcohol (contain 3 OH groups )
Called glycerin Synthesized from glucose
Colorless viscous oily liquid with sweet taste. Soluble in water and alcohols and insoluble in non polar fat solvents
On heating with sulfuric acid or KHSO (dehydration)
it gives acrolein (acryl aldehyde) that has a bad Odour. (detection of free glycerol or any compound containing glycerol)
Nutritive value by conversion into glucose and enters in structure of phospholipids
Glycerol enters in pharmaceutical and cosmetic preparations (hygroscopic)
◼ Nitroglycerin (glyceryl trinitrate) is used as vasodilator especially for the coronary arteries, thus it is used in treatment of angina pectoris. Also, enters in explosives manufacturing. Glycerol is used in treatment of glaucoma (increased intraocular pressure) due to its ability to dehydrate the tissue from its water content.
Fatty acids are aliphatic mono-carboxylic acids obtained from the hydrolysis of fats and oils.
General formula: R-(CH2)n-COOH
Saturated Unsaturated Short chain Long chain Monounsaturated Polyunsaturated
1. Saturated: they contain no double bonds and have the following general formula C n H 2n+1 COOH
They may be :
A-Short chain : 2-10 carbon atoms.
B-Long chain: more th an 10 carbon atoms
Ex: 16 C palmitic acid: CH 3 -(CH 2 ) 14 - COOH
18 C stearic acid: CH 3 -(CH 2 ) 16 -COOH
2. Unsaturated fatty acids: They contain double bonds. All unsaturated fatty acids are liquid at room temperature. They may be:
1- monounsaturated :contain one double bond.
2- polyunsaturated:contain more than one double bond.
Polyunsaturated fatty acids(Essential fatty acids) : They are essential fatty acids that can not be synthesized in the human body (due to lack of enzymes that can form more than one double bond) and must be taken in adequate amounts in the diet.
- They are required for normal growth and metabolism.
-They are liquids at room temperature.
⚫ Linoleic:c18:2∆ 9,12CH3-(CH2)4-CH=CH-CH2-CH=CH-(CH2)7-COOH
- Alpha Linolenic acid: in corn, linseed, peanut, olive, cottonseed and soybean oils.
CH3-CH2-CH=CH-CH2-CH=CH-CH2-CH=CH-(CH2)7-COOH
-Gamma Linolenic acid: CH3-(CH2)4-CH=CH-CH2-CH=CH-CH2-CH=CH-(CH2)4-COOH
⚫ Arachidonic acid: an important component of phospholipids in animal and in peanut oil.
CH3-(CH2)4-CH=CH-CH2-CH=CH-CH2-CH=CH-CH2-CH=CH- (CH2)3-COOH
⚫ Hydrolysis : They are hydrolyzed into their constituents (fatty acids and glycerol) by the action of super heated steam, acid, alkali or enzyme (e.g., lipase of pancreas).
NB: During their enzymatic and acid hydrolysis glycerol and free fatty acids are produced.
1- every animal and plant cell contain phospholipid. 2- cellular and subcellular membranes composed mainly of phospholipids.Thus the trasfer of substances throuh these membranes is controlled by the properties of phospholipids.
✓ Sources of dietary lipids ✓ Types of body lipid ✓ Lipolysis of Triglycerides ✓ Control of lipolysis in adipocytes ✓
-Oxidation - On an average, adult human eats about 100 - 150-gram lipids per day. The main lipids in diet are triacylglycerols which constitute most of fat and oils we eat, but diet contains also some phospholipids and cholesterol.
1-cell membrane and nervous system
2-mainly phospholipids and cholesterol
3-not affected by starvation
4- no energy production
1- Adipocytes (sub-cutaneous fat around organs)
2- mainly triglycerides 3-affected by starvation 4-energy production
Lipolysis is the hydrolysis of triacylglycerols to free glycerol and free fatty acids with both products leaving the adipocyte.
• Most tissues (with the exception of the brain due to blood brain barrier) use the fatty acids as a metabolic fuel for energy production or as a source of carbon for biosynthetic reactions.
• The glycerol, which cannot be used by adipose tissue cells (no glycerol kinase), is taken up by the liver, intestine or kidney as a substrate for gluconeogenesis or re-esterification.
A. Covalent modification: Hormone-sensitive lipase is activated by covalent modification by cAMP-dependent protein kinase activates it.
Steps of lipolysis: NOTE
2.Antilipolytic hormones: Insulin: 0, Prolactin, in large doses acts similar to insulin. Prostaglandins such as PGE1 and nicotinic acid inhibit lipolysis by reducing cAMP levels. see below
- Fatty acids pool: The half-life of fatty acids in blood is very short (1-3minutes).
– They are derived from:
✓ lipolysis in adipose tissue
✓ degradation of chylomicrons and VLDL by lipoprotein lipase
✓ direct absorption from intestine (short and medium-chain fatty acids)
✓ synthesis in the liver.
They are transported in blood as free fatty acids (FFA).
✓ Short-chain fatty acids are water soluble and exist as fatty acid anions
✓ long-chain FFA are water insoluble and are combined with albumin.
–Fatty acids are taken by liver, kidney, muscles, mammary glands, intestine, adipose tissues and heart to be oxidized.
oxidation; is the most important pathway for fatty acid oxidation.
Definition:
-, and -oxidation of fatty acids are specialized pathways.
-Oxidation
-oxidation of fatty acids is the principal pathway for the catabolism of fatty acids.
-Oxidation involves oxidation of the -carbon to form a -keto acid catalyzed by a number of enzymes collectively called fatty acid oxidase.
Site:
Mitochondrial matrix of tissues such as liver, heart (80% of its fuel is from oxidation), lungs, muscles, kidney, testes, and adipose tissue.
Brain cells have -oxidation ability but blood brain barrier prevent the entrance of fatty acids to these cells, therefore brain depends on ketone bodies
- Fatty acids are activated by a reaction catalyzed by thiokinase (acyl CoA synthetase), in the presence of CoA – SH and ATP.
Long chain fatty acyl-CoAs cannot freely diffuse across the inner mitochondrial membrane, which is impermeable to acyl-CoA. Acyl CoAs can penetrate the inner mitochondrial membrane only in combination with carnitine. It is synthesized from lysine and methionine in liver and kidney.
- Carnitine transports long chain fatty acid (acyl CoA) into the mitochondria.
Three enzymes are associated with carnitine:
1- Carnitine acylcarnitine transferase I: It is present at the outer surface of the inner mitochondrial membrane,
2- Carnitine acylcarnitine translocase: It is present in the inner mitochondrial membrane.
3- Carnitine acylcarnitine transferase II: It is present at the inner surface of inner mitochondrial membrane
-Several enzymes, known collectively as “fatty acid oxidase” are found in the mitochondrial matrix adjacent to the respiratory chain (which is found in the inner membrane).
-These catalyze the oxidation of acyl CoA to acetyl CoA, the system being coupled with the phosphorylation of ADP to ATP:
✓ In -oxidation, 2 carbons are cleaved at a time from acyl-CoA molecules, starting at the carboxyl end.
✓ The chain is broken between the (2) and (3) carbon atoms, hence the name -oxidation.
✓ The 2-carbon units formed are acetyl CoA. Thus, palmitoyl-CoA forms 8 acetyl-CoA molecules. Then acetyl CoA groups undergo oxidation in citric acid cycle.
- After activation of the fatty acid to the acyl-CoA:
Step I: Unsaturation (dehydrogenation)
✓ Catalyzed by acyl CoA dehydrogenase enzyme.
✓ The coenzyme for this reaction is a flavoprotein, containing FAD as a prosthetic group, whose reoxidation by the respiratory chain requires the mediation of another flavoproteins termed electron transferring flavoprotein (ETFp).
✓ FADH2 is oxidized in mitochondrial respiratory chain to give 2 ATP.
✓ Catalyzed by enoyl CoA hydratase (crotonase) enzyme, which helps the addition of H2O to saturate the double bond.
Step
✓ Catalyzed by B hydroxyacyl CoA dehydrogenase.
✓ The coenzyme is NAD. Oxidation of NADH + H+ in mitochondrial respiratory chain gives 3 ATP.
✓ It is catalyzed by thiolase (-ketothiolase). It splits acyl CoA into acetyl CoA and acyl CoA (which is shorter than the first one by 2 carbon atoms).
• The process is repeated untill the whole fatty acid is broken into acetyl CoA, which are then oxidized to CO2 and H2O in Krebs’ cycle.
e.g., palmitic acid (16 C)
1) -oxidation of palmitic acid will be repeated 7 times producing 8 molecules of acetyl CoA.
2) In each time, FADH2 and NADH + H+ is produced and will be transported to the respiratory chain where:
FADH2 → 1.5 ATP NADH + H+ → 2.5 ATP So 7 times = 7 x 4 ATP → 28 ATP
3) Each acetyl CoA which is oxidized in citric acid cycle gives 10 ATP. 8 x 10 ATP → 80 ATP
4) 2 high energy phosphate bonds are utilized in the activation of fatty acid (first reaction and it occurs once).
Energy gain = Energy produced – Energy utilized = 28ATP + 80 ATP – 2 ATP = 108 ATP – 2 ATP
Energy gain = 106 ATP
Calculation of energetics of any fatty acid oxidation:
= [ (N/2 – 1) x 4 ATP] + [N/2 x 10 ATP] – 2 ATP
Where N = number of carbons of a fatty acid.
e.g. Stearic acid: Number of carbon atoms = 18 C. So energy produced = [(18/2-1) x 4 ATP] + [18/2 x 10 ATP] – 2 ATP = [(9-1) x 4 ATP] + [9 x 10 ATP] – 2ATP = 32 ATP + 90 ATP – 2 ATP = 120 ATP 1- Source of energy 2- Production of acetyl CoA: Acetyl CoA is converted to e.g., cholesterol, acetyl choline. 3- Ketone bodies formation. ➢ Regulation of -oxidation: - The key regulatory enzyme is CPT1 - When energy increases ( ATP), -oxidation is inhibited and vice versa.
•
esterification due to:
1. glycerol-3-phosphate
2. insulin acyl transferase enzyme
• B-oxidation due to:
1. carnitine acyl transferase in mitochondria entry of acyl groups into mitochondria
During starvation
• ketogenesis is stimulated due to:
Note ⬇️B-oxidation ➡️acetyl CoA⬇️ ➡️ketogenesis⬇️
1. glucagon lipolysis FFA in blood uptake of FFA by liver more FFA converted to acetyl CoA Krebs cycle or ketone bodies
• According to amount of energy in cell:
1. If there is excess ATP molecules acetyl CoA forms ketone bodies (one molecule of palmitate gives 33 mol of ATP on conversion to acetoacetate)
2. If there is decreased ATP mol acetyl CoA undergo Krebs cycle (one molecule of palmitate gives 129 mol of ATP on oxidation via Krebs cycle)
• ketogenesis allows liver to oxidize large quantities of FFA without consuming excess energy
• Not in liver because liver does not contain enzymes of ketolysis • enzymes of ketolysis: 1. thiophorase 2. aceto-acetate thiokinase • So, ketone bodies diffuse from liver into blood to be transported for extrahepatic tissues for ketolysis • extrahepatic tissues able to use ketone bodies as important sources of energy include: 1. skeletal muscles 2. heart muscle 3. renal cortex 4. Brain: also, in prolonged starvation it obtains 75% of its energy requirements by oxidation of acetoacetate, they are also oxidized in preference to glucose and to FFA
Loss:
• Normally 1 GTP (=ATP) is lost during succinyl-CoA ➡️ succinate in Krebs' cycle (Succinyl-CoA is used in for thiophorase)
Gain:
• B-hydroxybutyrate ➡️ acetoacetate yields an NADH = 3 ATP molecules by electron transport and oxidative phosphorylation
• Each mole of acetyl-CoA that is formed = 12 mol of ATP via TCA cycle, electron transport, and oxidative phosphorylation
• So, oxidation of acetoacetate = -1 + 24 = 23 mol of ATP
• Oxidation of B-hydroxybutyrate = -1 + 3 + 24 = 26 mol of ATP
• ketone bodies in blood above the normal level (0.3-2 mg/dL)
• leading to ketonemia associated with excretion of ketone bodies above normal level in urine causing ketonuria (Normally the urinary excretion of ketone bodies is less than one mg/24 hours)
• is a type of metabolic acidosis which is due to ketosis (ketone bodies are moderately strong acids)
• when ketone neutralized by blood buffers (bicarbonate) This depletes bicarbonate blood pH transfer K+ from intracellular fluid to blood causing hyperkalemia
• In severe cases of ketosis coma may be developed
• In advanced cases as in uncontrolled diabetes the condition may be fatal
• Causes of Ketosis:
Ketoacidosis Note Insulin is an antiketotic hormone
Sources of acetyl CoA:
- The acetyl CoA is formed from carbohydrate via the oxidation of pyruvate within the mitochondria
- but acetyl CoA doesn’t diffuse into the cytoplasm, the principle site of fatty acid synthesis.
- Thus acetyl CoA condenses with oxaloacetate to form citrate which can pass out the mitochondrial membrane.
- In the cytoplasm, citrate is splitted again by ATP-citrate lyase enzyme into acetyl CoA and oxaloacetate.
Active acetate
+ oxaloacetate Citrate synthase
(Acetyl CoA)
- Acetyl CoA molecules are used for palmitic acid synthesis.
- Oxaloacetate which cannot cross the mitochondrial inner membrane is converted to malate.
Sources of NADPH + H
I- HMP shunt:
The main source of NADPH + H+ :
1.Glucose-6-phosphate dehydrogenase (G6PD).
2.6-phosphogluconate dehydrogenase.
II- Malic enzyme:
- This is a cytoplasmic enzyme which catalyzes the following reaction:
III- The action of cytoplasmic isocitrate dehydrogenase on isocitrate:
The coenzyme of the cytoplasmic isocitrate dehydrogenase is NADP+.
IV- Glutamate dehydrogenase reaction
UDPglucuronic acid hydroxylase H20 UDP
UDP-glucose Glucuronic acid VI- Mitochondrial transhydrogenase ( NADH.H + NADP ↔ NAD + NADPH.H )
Enzymes of extramitochondrial pathway: (fatty acid synthase)
- The enzyme is a dimer (formed of two monomers).
- Each monomer contains 7 enzymes and a terminal protein called acy lcarrier protein (ACP)
- ACP is a protein contains the vitamin pantothenic acid in the form of phosphopantothenate
- Each monomer contains 2 SH group , one provided by phosphopantothenate and attached to ACP . the other provided by cysteine attached to the enzyme ketoacyl synthetase.
- The 2 monomers are arranged head to tail. The diagramatic presentation of multienzyme complex is: HS-Cyst A P ant-sh
Synthesis of malonyl CoA
It is synthesized from acetyl CoA, ATP, biotin and CO2 in the presence of acetyl CoA carboxylase which is the rate limiting enzyme regulating the denovo synthesis of fatty acids.
1- Formation of acetyl malonyl enzyme complex.
⚫
Initially a priming molecule of acetyl-CoA combines with the cysteine-SH group catalyzed by acetyl transacylase.
⚫
Malonyl CoA combines with the adjacent –SH on the 4’phosphospantetheine of ACP of the other monomer, catalyzed by malonyl transacylase to form acetyl- malonylenzyme.
⚫ Both acetyl transacylase and malonyl transacylase are the same enzyme (transacylase).
2- The acetyl group attacks the methylene group of the malonyl residue catalyzed by ketoacyl synthase, and liberates CO2, forming 3-ketoacyl enzyme (acetoacetyl enzyme).
3- Reduction of 3- or Beta-ketoacyl enzyme to 3- or Beta-Hydroxy acyl enzyme by 3- keto acyl reductase in the presence of NADPH+H+ as a coenzyme.
4- A molecule of H2O is removed from Beta-Hydroxy acyl enzyme, forming Alpha ,Beta- unsaturated acyl enzyme or 2,3-unsaturated acyl enzyme. This reaction is catalyzed by hydratase enzyme.
5- The Alpha ,Beta unsaturated acyl enzyme is reduced by NADPH+H+ in the presence of enoyl reductase enzyme to acyl enzyme containing acyl group (fatty acid group) composed of 4-carbons.
6- A new malonyl–CoA molecule combines with the –SH of the 4’phosphopantetheine, displacing the saturated acyl residue onto the free cysteine –SH group.
7- The sequence of reactions is repeated 6 more times, a new malonyl residue being incorporated during each sequence, until a saturated 16-carbon acyl radical (palmityl) has been formed.
8- Palmitic acid is liberated from the enzyme complex by the activity of a sixth enzyme in the complex; thioesterase (deacylase) enzyme
The overall reaction is :
Acetyl CoA+ Malonyl CoA + 14NADPH+H+
Palmitic + 7CO2 + 6H2O +8CoA-SH+ 14 NADP +
Fete of palmitate
1- Esterification: Palmitate may undergo esterification with glycerol or cholesterol
2-Chain elongation: Palmitate may be elongated to form a fatty acid having a number of carbons more than 16.
3- Desaturation :
i.e. synthesis of unsaturated fatty acids; stearic acid which is derived from elongation of palmitate may undergo desaturaiton at C9 and C10 to form oleic acid (unsaturated fatty acid).
4- Sphingosine formation: It is formed from palmityl CoA and the amino acid serine.
The rate limiting reaction in the lipogenic pathway is at the acetyl CoA carboxylase step
1-Citrate: Which increases in concentration in the well fed state and is an indicator of a plentiful supply of acetyl CoA.
2-Insulin: The presence of insulin leads to dephosphorylation and activation of acetyl CoA carboxylase enzyme through the inhibition of cAMP.
- Acetyl CoA carboxylase is covalently regulated by phosphorylation dephosphorylation process.
- The dephosphorylated form is the active form whereas the phosphorylated form is inactive.
- Insulin activate the pyruvate dehydrogenase complex and citrate lyase both of which supply acetyl CoA.
Inhibted by long-chain acyl CoA:
1- inhibits allosterically acetyl CoA carboxylase enzyme.
Thus if long-chain acyl CoA molecules are increased either due to excessive lipolysis or decreased esterification Inhibition of acetyl CoA carboxylase Inhibition of synthesis of a new fatty acids 2- inhibits transport of citrate from the mitochondria to cytosol. 3- inhibits pyruvate dehydrogenase (PDH). This decreases acetyl CoA which is essential for lipogenesis. 4- inhibits citrate synthase.
Site :occurs in mitochondria under anaerobic conditions .
It is the same steps as Beta -oxidation but in the opposite direction.
- Active acetate acts as a donner for the 2-carbons used in elongation of the preexisting F.A. in this type of synthesis.
- The enzymes which are involved are those of Beta -oxidation except :
1- Condensation of acetyl CoA with acyl CoA:
- Pyridoxal phosphate acts as a coenzyme for the enzyme responsible for condensation of acetyl CoA with acyl CoA.
- Therefore thiolase enzyme not used in this reaction.
2- Conversion of Alpha , Betaunsaturated CoA to acyl CoA:
- Acyl CoA dehydrogenase and flavoprotein coenzyme are not used.
- This reaction needs reductase enzyme and NADPH + H+ as coenzyme.
This is the main site for chain elongation of pre-existing fatty acid molecules. i.e. production of fatty acids higher than 16 carbon atoms.
- The microsomal pathway needs malonyl CoA as the two carbons donor and NADPH + H+ coenzyme as a reductant.
Function: This system utilizes NADH+H+ it will act only when there is high NADH+H+ /NAD+ ratio that occurs under anaerobic conditions.
-Thus the cells can get rid off excess NADH+H+ .
Function: This system becomes active during myelination of the nerve in order to provide C22 and C24 fatty acids that are present in sphingolipids.
- Acylglycerols constitute the majority of lipids in the body.
- Triacylglycerols are the major lipids in fat deposits and in food.
- Both glycerol and Fatty acids must be activated by ATP before they can be incorporated into Acylglycerols.
- It occurs mainly in microsomes of tissues as liver, kidney, intestine, adipose tissues and lactating mammary gland
- Glycerol kinase will catalyze the activation of glycerol to glycerol-3phosphate. - If this enzyme is absent or low in activity, as it is in muscle or adipose tissue; - Most of the glycerol 3-phosphate must be derived from an intermediate of the glycolytic system, (dihydroxy acetone phosphate), which forms glycerol-3-phosphate by reduction with NADH catalyzed by glycerol-3-phosphate dehydrogenase.
Activation of Fatty Acids.
✔ Catabolism of amino acids. ✔ Blood ammonia.
Unlike fats and carbohydrates, amino acids are not stored by the body, Therefore, amino acids must be obtained from the diet, synthesized de novo, or produced from normal protein degradation.
Any amino acid in excess of the biosynthetic needs of the cell is rapidly degraded.
The first phase of catabolism involves the removal of the α-amino groups usually by:
Transamination. Subsequent oxidative deamination.
Forming ammonia and the corresponding α-keto acid the “carbon skeletons” of amino acids.
A portion of the free ammonia is excreted in the urine, but most is used in the synthesis of urea.
The first step in the catabolism of most amino acids is → The transfer of their α-amino group to αketoglutarate.
NOTE
All amino acids are transaminable except lysine and threonine.
The products are → an α-keto acid (derived from the original amino acid) and glutamate.
α-Ketoglutarate plays a key role in amino acid metabolism by accepting the amino groups from most amino acids, thus becoming glutamate.
Glutamate produced by transamination can be oxidatively deaminated or used as an amino group donor in the synthesis of nonessential amino acids.
This transfer of amino groups from one carbon skeleton to another is catalyzed by a family of enzymes called aminotransferases (formerly called transaminases), these enzymes are found in the cytosol and mitochondria of all body cells, especially those of the liver, kidney, intestine, and muscle.
AST
❖ Substrate specificity of aminotransferases:
✓ Each aminotransferase is specific for one or, at most, a few amino group donors.
✓ Aminotransferases are named after the specific amino group donor, because the acceptor of the amino group is almost always α-ketoglutarate.
✓ The two most important aminotransferase reactions are catalyzed by alanine aminotransferase (ALT) and aspartate aminotransferase (AST).
❖ Mechanism of action of aminotransferases:
✓ All aminotransferases require the coenzyme pyridoxal phosphate (a derivative of vitamin B6), which is covalently linked to the ε-amino group of a specific lysine residue at the active site of the enzyme.
✓ Aminotransferases act by transferring the amino group of an amino acid to the pyridoxal part of the coenzyme to generate pyridoxamine phosphate.
✓ The pyridoxamine form of the coenzyme then reacts with an α-keto acid to form an amino acid, at the same time regenerating the original aldehyde form of the coenzyme.
❖ Diagnostic value of plasma aminotransferases: ✓ Amino transferases are normally intracellular enzymes, with the low levels found in the plasma representing the release of cellular contents during normal cell turnover. ✓ The presence of elevated plasma levels of aminotransferases indicates damage to cells rich in these enzymes. For example, physical trauma or a disease process can cause cell lysis, resulting in release of intracellular enzymes into the blood.
1- Liver disease:
- Plasma AST and ALT are elevated in nearly all liver diseases, but are particularly high in conditions that cause extensive cell necrosis, such as severe viral hepatitis, toxic injury, and prolonged circulatory collapse.
- Serial enzyme measurements are often useful in determining the course of liver damage.
Non hepatic disease:
Aminotransferases may be elevated in non-hepatic disease, such as myocardial infarction and muscle disorders. - However, these disorders can usually be distinguished clinically from liver disease.
✓ Two aminotransferases AST and ALT are of particular diagnostic value when they are found in the plasma.
ALT is more specific than AST for liver disease, but the latter is more sensitive because the liver contains larger amounts of AST
(Oxidative
The amino groups of most amino acids are ultimately funneled to glutamate by means of transamination with α-ketoglutarate.
Glutamate is unique in that it is the only amino acid that undergoes rapid oxidative deamination, a reaction catalyzed by glutamate dehydrogenase.
Therefore, the sequential action of transamination (resulting in the collection of amino groups from most amino acids onto α-ketoglutarate to produce glutamate) and the oxidative deamination of that glutamate (regenerating αketoglutarate) provide a pathway where by the amino groups of most amino acids can be released as ammonia.
Two mechanisms are available in humans for the transport of ammonia from the peripheral tissues to the liver for its ultimate conversion to urea.
Found in most tissues, uses glutamine synthetase to combine ammonia (NH3) with glutamate to form glutamine a nontoxic transport form of ammonia.
The glutamine is transported in the blood to the liver where it is cleaved by glutaminase to produce glutamate and free ammonia.
Used primarily by muscle, involves transamination of pyruvate (the end product of aerobic glycolysis) to form alanine.
Alanine is transported by the blood to the liver, where it is converted to pyruvate.
Introduction :
If ammonia was allowed to accumulate in the body, it would be toxic.
Normally the blood contains 10 - 80 μg/dL ammonia that is controlled by the liver.
If the concentration becomes higher, it results in ammonia intoxication (hyperammonemia) that is particularly toxic to the brain by causing coma and may be death if uncontrolled.
Thus, it is rapidly removed from the circulation by the liver (mainly) and converted either to glutamate, glutamine, or urea.
In liver cirrhosis ammonia is not converted into urea. Ammonia passes directly to systemic blood due collaterals and reaches toxic concentration to the brain. The brain detoxifies ammonia into glutamine that depletes α-ketoglutarate of Krebs' cycle. This leads to energy failure in the brain cells. Moreover, abnormal reaction of ammonia with α-ketoglutarate produces the toxic α-ketoglutaramate.
(Main source)
Transamination followed by deamination of amino acids (Main source)
✓ This becomes significant source in constipation, gastrointestinal hemorrhage and high protein diet.
✓ It is very important to be prevented in liver disease, otherwise ammonia intoxication would occur.
✓ Intestinal antiseptic antibiotics such as neomycin are very beneficial in such cases.
✓ Deamination of other nitrogenous base, e.g., purines and pyrimidines and monoamines.
Anabolic fates:
- The free ammonia removed by deamination enters the ammonia pool of the body and may be used for synthesis of:
Catabolic fate (direct excretion):
- Ammonia is excreted directly into the urine as it is.
Synthesis of non-essential amino acids by the reversible transamination of α-keto acids.
- 60% of that ammonia is derived from the glutaminase reaction as the kidney catabolic part of glutamine cycle.
Synthesis of purines, pyrimidines, porphyrins and sugar amines:
N1, N3, N9 of purines are derived from ammonia (from aspartate and glutamine). N1 of pyrimidine is derived from ammonia (as carbamoyl phosphate).
- Porphyrins have glycine incorporated in their structure.
- 40% of that ammonia comes from direct deamination of other amino acids by different deaminases in the kidney.
- Urine contains also other nitrogenous non-protein compounds such as creatinine and uric acid.
It is one of the mechanisms for detoxification of ammonia in extra- renal tissues particularly brain, kidney, retina, muscles and liver as glutamine.
Glutamate reacts with ammonia in presence of ATP, Mg2+ and the mitochondrial glutamine synthetase to produce glutamine. This is particularly important for the brain and nervous tissue.
Glutamine goes via the blood from the various tissues to the kidney (also in liver, brain and retina) where it is hydrolyzed by glutaminase enzyme into glutamate and ammonia.
Normal blood glutamine level is 6 - 12 mg/dl and becomes 30 - 54 mg/dL during hepatic coma.
The ammonia is then excreted in the urine in exchange with sodium and represents 60% of the total urinary ammonia.
Glutamine has several other fates, e.g., transamidination, detoxication by conjugation and purines and pyrimidines synthesis.
It is a five-reaction cyclic cascade that utilizes two amino groups and a CO2 to synthesis urea in mitochondria and cytosol of the liver.
Urea is the major disposal form of amino groups derived from amino acids, and accounts for about 90% of the nitrogen-containing components of urine.
One nitrogen of the urea molecule is supplied by free ammonia, and the other nitrogen by aspartate.
The carbon and oxygen of urea are derived from CO2.
Urea is produced by the liver, and then is transported in the blood to the kidneys for excretion in the urine.
Site: Mitochondria and cytoplasm of the liver cells.
carbamoyl-phosphate:
✓ Condensation of one mole of ammonia, one mole of CO2 and one mole of phosphate derived from ATP to form carbamoyl phosphate is catalyzed by the mitochondrial carbamoyl phosphate synthetase I, an enzyme present in the liver mitochondria of ureotelic organism including man. There is a liver cytosolic carbamoyl phosphate synthetase II that is utilized in pyrimidine biosynthesis.
✓ The reaction requires Mg2+ or Mn2+ as cofactor and N-acetyl glutamate as an allosteric activator (increases the enzyme affinity to ATP).
✓ Transfer of the carbamoyl residue from carbamoyl phosphate into ornithine to form citrulline with liberation of phosphate is catalyzed by the mitochondrial ornithine carbamoyl transferase (ornithine transcarbamoylase).
✓ Citrulline goes to cytosol (facilitated by a specific transporter) to complete the cycle.
✓ Ornithine carbamoyl transferase is used as a test for liver function. The level of this enzyme in blood is increased by many diseases, e.g., liver metastasis in breast cancer and in malignant lymphomas.
✓ Citrulline is joined to the amino group of aspartate to form argininosuccinate, catalyzed by argininosuccinate synthetase that requires ATP and Mg2+ with liberation of H2O.
✓ Citrullyl-AMP is an active intermediate formed during the reaction.
✓ It is catalyzed by the argininosuccinase found in liver and kidney tissues.
✓ Fumarate formed by this cleavage joins Krebs' cycle.
✓ Liberation of urea is brought about by cleavage of arginine catalyzed by arginase.
✓ The formed ornithine returns back to mitochondrial (facilitated by a specific transporter).
✓ This enzyme is present mainly in the liver of all ureotelic organisms. However, smaller quantities also occur in testes, skin and mammary gland.
- Regulation is concentrated on the key regulatory steps of carbamoyl phosphate synthetase I and also on glutamate dehydrogenase as preparatory step that provide substrate (ammonia).
- Thus, glutamate dehydrogenase channels ammonia from all transaminable amino acids to carbamoyl phosphate synthetase I for synthesis of carbamoyl phosphate and urea.
- The major allosteric activator for carbamoyl phosphate synthetase I is the Nacetyl-glutamate.
- Urea formation increases during starvation and lack of energy to cope with the increased rate of protein breakdown.
- High protein diet in non-growing individuals increases urea synthesis.
✔ Understand the definition of decarboxylation.
✔ Know some important examples.
✔ Be able to define phenylalanine and tyrosine metabolism.
It is the removal of carbon dioxide (CO2) from amino acids with formation of amines. Catalyzed by decarboxylase which uses PLP as a coenzyme.
PLP (coenzyme) CO2 (Amine)
These are products of simple decarboxylation of amino acids. Usually amine have high physiological activity like (hormones, neurotransmitters, …etc).
• Tyramine from Tyrosine
• Dopamine from (DOPA)
• Ethanolamine from Serine
• Serotonin from Tryptophan
• SAM amine from SAM
• GABA from Glutamic acid
• Histamine from Histidine
• Arginine-nitric oxide
• Creatine-arginine & glycine
• Carnitine-lysine & methionine
1. Tyramine is a vasoconstrictor and increases the blood pressure. 2. Stimulates uterine contractions.
Tyrosine decarboxylase (tdc) Tyramine Tyrosine
2-Dopamine from DOPA
Dopamine is a hypothalamic neurotransmitter that is derived from tyrosine. It’s a precursor for adrenaline and noradrenaline.
1) the prefix “de” means “removal” 2) co-enzyme: a nonprotein compound that is necessary for the function of an enzyme.
Parkinson’s disease is a neurological disorder associated with underproduction of dopamine.
Ethanolamine enters in the structure of phospholipids; by transmethylation gives choline.
Enter in structure of phospholipids.
form acetylcholine ( neurotransmitter ) when condensed with acetyl-COA
-Serotonin is vasoconstrictor (like Tyramine).
-Smooth muscle contraction e.g bronchoconstriction& diarrhea.
-Excitatory neurotransmitter so drugs blocking serotonin catabolism used in treatment of depression.
5-Hydroxy-tryptamine (serotonin)
• S-adenosyl methionine amine (SAM amine) used for biosynthesis of polyamines (spermidine-spermine).
• S-adenosyl methionine (SAMe) is a major methyl donor in the brain and is also antidepressant .
Remember that:
PLP (pyridoxal phosphate) is a coenzyme and it’s the active form of vit. B6
6-GAPA from Glutamic acid
GABA (gamma-aminob utyric acid) is an inhibitory neurotransmitter, decrease its level in the brain causing convulsions.
Decrease of PLP causing convulsions due to decrease in GABA in the brain.
An inflammatory agent (in allergy).
Blockage of Histamine receptors prevent HCL secretion so used in treatment of gastric hyperacidity & peptic ulcer, e.g. cimetidine is a histamine receptor antagonist.
Now here comes part two of this topic :
Arginine is the precursor for biological synthesis of nitric oxide which is synthesized from arginine in an NADPH-dependent reaction catalyzed by nitric oxide synthase
1. Acts as a biological messenger –how ?- it’s a simple gaseous substance, diffuses readily through membranes.
2. Plays –in humans- a role in a range of physiological processes, including:
• Neurotransmission.
• Vasodilatation.
• Wound healing.
• prevents platelets aggregation.
• smooth muscle relaxation.
• It helps macrophages function.
1. Creatine phosphokinase “CPK” is the enzyme that catalyzes the formation of creatine-p from creatine.
2. Creatinine is an important kidney function test, its normal level 0.7 – 1.4 mg/dL in male & 0.6 –1.2 mg/dL in female.
CPK has 3 iso-enzymes: 1-CPK-MM in skeletal ms. 2-CPK-MB in cardiac ms. 3-CPK-BB in brain cells.
It is important for “fatty acid oxidation” as it transports fatty acids into mitochondria where they are oxidized.
-Essential amino acid.
-Aromatic amino acid.
-Both ketogenic & glucogenic -Converted into tyrosine.
(Degradation
1.Mostly through tyrosine.
2.Is hydroxylated at paraposition by phenylalanine hydroxylase to form tyrosine.
3.This reaction is irreversible and requires specific coenzyme biopterin which is structurally related to folate.
-Non essential amino acid. -Aromatic amino acid.
-Glucogenic & partly ketogenic.
- Synthesis from phenylalanine.
1.Phenylalanine is converted to tyrosine; a single pathway is responsible for degradation of them both.
2.Occurs mostly in liver.
3.Tyrosine first undergoes transamination to parahydroxyphenylpyruvate catalyzed by tyrosine transaminase (PLP-dependent)
This figure shows the conversion of phenylalanine to tyrosine:
Catecholamine
1-Melanin
Synthesis of melanin:
Melanin is a pigment of skin, hair and eye. The synthesis of it occurs in melanosomes present in melanocytes “the pigment producing cells”
Tyrosine is precursor for melanin and only one enzyme namely tyrosinase (a copper containing oxygenase), is involved in its formation.
Tyrosinase hydroxylates tyrosine to form 3,4 dihydroxy-phenylalanine (DOPA). DOPA can act as a cofactor for tyrosinase. This reactioninhibited by tyrosine- regulates the synthesis of melanin.
-Another pathway-
Cysteine condenses with dopamine and in the next series of reactions results the synthesis of red melanins.
The skin color of the individual is determined by the relative concentrations of black & red melanins. this –in turn- is dependent on many factors, both genetic & environmental. These include: [activity of tyrosinase- density of melanocytes -availability of tyrosine ..etc]
The presence of moles on the body represents a localized severe hyperpigmentation due to hyperactivity of melanocytes.
1. Thyroid hormones: tetra-iodothyronine [thyroxine] and triiodithyronine are synthesized from the tyrosine residues of the protein thyroglobulin & activated iodine.
2. Iodination of tyrosine ring occurs to produce mono & di-iodotyrosine from which tri-iodothyronine (T3) and thyroxine (T4 or tetraiodothyronine) are synthesized.
3. The protein thyroglobulin undergoes proteolytic breakdown to release the free hormones T3 & T4.
2- They are derived from tyrosine.
3- Tyrosine is the precursor for the synthesis of catecholamines, namely dopamine, norepinephrine (noradrenaline) and epinephrine (adrenaline).
4- Tyrosine is converted to catecholamines in adrenal medulla and CNS.
-the name catechol refers to the dihydroxylated phenyl ring (it’s nucleus)
-Amines derivatives of it are called catecholamines
1- Tyrosine is hydroxylated to 3,4-dihydroxyphenylalanine (DOPA) by tyrosine hydroxylase which is a rate limiting enzyme requires tetrahydrobiopterin as a coenzyme. In contrast to this enzyme, tyrosinepresent in melanocytes- converts tyrosine to DOPA.
1) A catecholamine.
2) An inhibitor of prolactin secretion.
3) A neurotransmitter in substantia nigra, extrapyramidal tract & striatal tract
4) In Parkinsonism the dopamine in the brain is reduced. ( as dopamine enter the brain cells, the precursor L-DOPA is used as a drug in parkinsonism).
5) Alpha methyl DOPA will inhibit DOPA decarboxylase & prevent production of epinephrine so it’s an antihypertensive drug.
1) Increases blood pressure.
2) Increases the rate & force of myocardial contraction.
3) Causes relaxation of smooth muscle of bronchi.
4) Increases glycogenolysis (anti-insulin in nature) & stimulates lipolysis.
5) Is released from adrenal medulla in response to flight, fight, exercise and hypoglycemia.
it’s half-life of epinephrine is 2-5 minutes. it’s catabolized in tissues, by catechol-O-methyl transferase (COMP) to metanephrine. it’s then acted upon by mono amine oxidase (MAO), which will oxidatively deaminate metanephrine.
the major end product is 3-hydroxy-4-methoxymandelic acid or vanillyl mandelic acid (VMA).
normally VMA is excreted 2-6 mg/24 hrs.
VMA is increased in pheochromocytoma and neuroblastoma.
✔ What is Phenylketonuria
✔ Discuss inborne errors of phenyl alanine and Tyrosine
Most common metabolic disorder in AA metabolism
Autosomal recessive with Incidence of PKU is 1 in 10,000 births.
Due to deficiency of the hepatic enzymes, phenylalanine hydroxylase. Defect in dihydrobiopterin reductase is also reported
PKU primarily causes the accumulation of phenylalanine in tissues and blood & excretion in urine
Disturbance in routine metabolism, phenylalanine is diverted to alternative pathways resulting in the excessive production of phenypyruvate, phenylacetate, phenyllactate and phenylglutamine: excreted in urine.
Alternatice pathway for catabolism of phenylalnine in phenylaketonuria.
• Phenypyruvate
• phenylacetate
• phenyllactate
• phenylglutamine
A. Mental retardation, failure to walk or talk, failure of growth, seizures and tremor
B. Accumulation of phenylalanine in brain impairs the transport & metabolism of other aromatic a.a. (tryptophan & tyrosine)
C. Impaired synthesis of serotonin
D. Defect in myelin formation
Melanin is the pigment synthesized from tyrosine by tyrosinase Accumulation of phenylalanine competitively inhibits tyrosinase and impairs melanin formation The result is hypopigmentation that causes light skin color, fair hair, blue eyes
3. Elevated levels of phenylalnine, phenylpyruvate, phenylactate and phenylacetate are found in plasma & urine giving mousey odor.
Normal level in newborns: 1 -2 mg/dl
PKU: 20 -65 mg/dl Phenylpyruvate in urine can be detected by ferric chloride test (green color) – non specific test
Amino acid measurement using tandem mass spectrometry (MS/MS). Measurements done using MS/MS determine the concentration of Phenylalanine and the ratio of Phenylalanine to tyrosine, the ratio will be elevated in PKU
type II
Characterized by skin and eye lesions and rarely mental retardation
Disturbed self-coordination is seen in these patients Neonatal tyrosinemia
Caused by absence of enzyme p-hydroxyphenyl pyruvate dioxygenase
Mostly temporary condition and usually responds to ascorbic acid
Substrate inhibition of the enzyme is overcome by the presence of ascorbic acid.
Autosomal recessive disorder with 1 in 25,000 births
Defective enzyme: homogentisate oxidase in tyrosine metabolism
Homogentisate accumulates in tissues and blood and is excreted into urine
On standing, Homogentisate gets oxidized to corresponding quinones, which polymerize to give black or brown color
Urine resembles coke in color
Deposition occurs in connective tissue, bones and various organs (nose, ear) resulting in a condition known as ochronosis
Arthritis; due to deposition of pigment alkaptons in the joints
Treatment by consumption of protein diet relatively low phenylalanine content
Chromatography for quantification of Homogentisate
Due to deficiency of the Enzymes fumarylacetoacetate hydroxylase and/or maleylacetoacetate isomerase
Rare but serious disorder
Causes liver failure, rickets, renal tubular dysfunction and polyneuropathy Tyrosine and its metabolites are excreted in urine
In acute tyrosinosis, the infant exhibits diarrhea, vomiting and cabbage-like odor Death may be seen due to liver failure within 1 year
Treatment: diets low in tyrosine, phenylalanine and methionine
Albino – white
Inborn error due to lack of synthesis of the melanin pigment
Defect in tyrosinase enzyme
Autosomal recessive disorder with 1 in 20,000 Biochemical basis:
1. Deficiency or lack of the enzyme tyrosinase 2. Decrease in melanosomes of melanocytes 3. Impairment in melanin polymerization 4. Limitation of substrate (tyrosine) availability 5. Lack of protein matrix in melanosomes
Photophobia with lack of pigment in the eyes
Lack of melanin pigments makes skin sensitive to sunlight
✔ Glycine synthesis , catabolic and anabolic fates .
✔ Serine synthesis ,catabolic and anabolic fates.
✔ Sulfur_containing amino acids (methionine , cystine ).
- Participates in synthesis of several biologically active compounds.
- It is a neutral, aliphatic,glucogenic and non-essential amino acid.
- It is the simplest amino acid (2C).
- It produced denovo (CO2 + NH3), from threonine, serine, glyoxylic acid & choline: Threonine.
Serine.
, transamination catalyzed by glycine transaminase in the cytosol of liver is a salvage process for regenerating glycine on the expense of glutamate or alanine. Failure of this salvage process delivers glyoxylate into oxalate formation leading to primary hyperoxaluria
Choline, see choline cycle in the catabolic fates.
De-novo synthesis , by reversal of glycine cleavage catalyzed by glycine synthase, a liver mitochondrial enzyme,
It is catabolized into CO2 and NH3 through glycine synthase
Into CO2 and formyl-FH4 and/or oxalate through glyoxylate and formate,
Into pyruvate through serine or methylglyoxal.
reversibly transaminated into glyoxylate that gives formate with conversion of alpha-ketoglutarate into glutamate catalyzed by glycine transaminase.
It is conjugated in the liver with benzoic acid (a toxic food additive) giving hippuric acid, which is excreted in the urine. Glycine conjugation is used to detoxify several other drugs. This reaction is used as a liver function test
1. Protein synthesis, e.g., collagen (every third amino acid in the helix is glycine).
2. Synthesis of porphyrins (haem): -
Succinyl-CoA condenses with glycine and the COOH of glycine is liberated as CO2 to give ð-aminolevulinate catalyzed by ð-aminolevulinate synthase, a mitochondrial enzyme, then two molecules of ð-aminolevulinate are condensed together with the releases of two water molecules to form porphobilinogen, the building unite of haem, see metabolism of porphyrins. ð-Aminolevulinate can be oxidatively deaminated into ∑- ketoglutarate and succinate.
3. Synthesis of bile salts: - Glycine conjugates with cholic or chenodeoxycholic acids to form glycocholate or glycochenodeoxycholate (see cholesterol metabolism).
4. Synthesis of purines: - Carbons 4 and 5 and nitrogen 7 of purines are derived from glycine
5. Synthesis of glutathione: - It is a tripeptide formed from 3 amino acids (glutamic acid, cysteine and glycine). It is formed in the cytoplasm of the liver by the help of ATP and - glutamyl-cysteine synthetase and glutathione synthetase. Glutathione exists in 2 states reduced with a free SH group, GSH and oxidized without a free SH group but a disulfide linkage, GSSG.
Reduced glutathione function :
1) Amino acid transport across the cell membrane (including absorption).
2) Coenzyme : It is a coenzyme for maleylacetoacetate isomerase enzyme, see tyrosine. It is also a coenzyme in S-adenosylmethionine and heme synthesis, and for formaldehyde dehydrogenase, glycogen synthase and glyoxylase.
3) Synthesis of leukotrienes (see prostaglandins).
4) Detoxication by conjugation (see metabolism of xenobiotics).
5) As hydrogen donor in oxidation reduction reactions that include: It protects hemoglobin against oxidation by hydrogen peroxide. Keeps the integrity of the cell membrane against damage by oxidants, e.g., H2O2. Thus, it prevents lipid peroxidation of the membrane lipids of RBCs and thence its hemolysis by various oxidants because it is the most liable among cells, sees Favism.
6) It maintains the SH group(s) at the active site of several enzymes that is essential for their activity, e.g., glyceraldehyde dehydrogenase.
7) It inactivates insulin in the liver by reductive cleaving of the 2 disulfide linkages of insulin into separate polypeptide chains catalyzed by insulinglutathione transhydrogenase.
8) It protects the R-cells of pancreas from the degenerative action of alloxan or dehydroascorbic acid. This protective action is due to antioxidant properties of glutathione, which reduces alloxan to inactive substance and reduces dehydroascorbic acid to ascorbic acid.
- Creatine is formed from glycine and parts of arginine and methionine. Creatine presents in muscle (98%), brain (1.3%), liver, testes, whereas, phosphocreatine is intracellular only.
- It is synthesized in two steps, the first in the mitochondria of kidney, pancreas and to a limited extent in liver, and the second (transmethylation) in the liver and pancreas, and goes to blood to be extracted by tissues particularly the muscles and nerves. Synthesis is regulated by growth hormone and creatine level.
- Creatine phosphate (phosphagen) is the main store of high energy in muscles. During muscular contraction, creatine phosphate regenerates ATP from ADP catalyzed by creatine kinase and during rest ATP regenerates creatine phosphate catalyzed by ATP-creatine transphosphorylase and the kinase,
- 1 - 2% of the total body creatine phosphate is spontaneously dephosphorylated dehydrated daily into creatinine that diffuses to blood and then through the kidney to urine. Creatinine clearance rate from blood into urine is a kidney function test. Males excrete 1.5 - 2 gm and females 0.8 - 1.5/daily, but no creatine normally except in children, pregnancy and after parturition, fevers, thyrotoxicosis, muscle damage, low carbohydrate diet, diabetes mellitus, wasting diseases and starvation. Blood level of creatine is 0.2 - 0.9 mg/dL and creatinine is 0.8 - 2.0 mg/dL.
- Choline is synthesized from glycine through serine and is then catabolized again into glycine, forming what is called choline cycle. It is used for lecithin, plasmalogens, sphingomyelin and acetyl-choline synthesis. It is a lipotropic factor that prevents fatty liver.
Primary hyperoxaluria: It is due to failure of catabolism and/or transamination of glyoxylate either to formate or glycine, respectively. The accumulated glyoxylate is oxidized to oxalate that causes bilateral calcium oxalate stones, nephrocalcinosis, recurrent urinary infection, and early mortality from renal failure or hypertension
. Glycinuria: It is due to defective renal reabsorption of glycine leading to excretion of 0.6 - 1.0 gm glycine/day with normal blood glycine level. The patient has tendency to precipitate renal oxalate stones.
- It is a hydroxyl containing, glucogenic and non-essential, amino acid that can be synthesized in the body.
From glycine
From 3-phosphoglycerate
By hydroxymethyl transferase .
By two mechanisms as follows
- Serine is converted to pyruvate by serine dehydratase and pyridoxal phosphate as a coenzyme. It is also converted through glycine into other products of glycine catabolism.
1) It participates in protein synthesis. It is especially important as a part of proteins because it is a site for covalent modification of its OH group by phosphorylation and dephosphorylation regulatory mechanisms
2) Glycine synthesis.
3) Ethanolamine and choline synthesis.
4) Sphingosine synthesis: sphingosine enters in structure of sphingolipids.
5) Cephalins synthesis: They contain serine as a nitrogenous base.
6) Thymine synthesis: Serine last carbon (as methylene-FH4) may be used as a source of methyl group for conversion of UMP into TMP, see synthesis of pyrimidines.
7) Synthesis of cysteine: Homocysteine formed during transmethylation reaction is regenerated into methionine by methionine synthase, methyl-FH4 and methylcobalamin, see transmethylation. The majority of homocysteine, however, is converted into cysteine on the expense of serine that is converted into homoserine, then alpha-ketobutyrate that is converted into propionylCoA then succinate.
- It is a glucogenic (gives propionate), essential, and sulfur-containing amino acid.
-Its major role is being a methyl donor giving its methyl group after activation by ATP into S-adenosyl-methionine, see transmethylation.
- Methionine is a lipotropic factor preventing fatty liver by being a methyl donor.
- It is a transaminable amino acid that gives methyl-thio-butyrate that is hydrolyzed into alpha-keto-c-butyrate and methylmercaptan in the liver.
- It is also catabolized after giving its methyl group into homocysteine, then cysteine and homoserine that gives propionyl-CoA that give succinate, see serine metabolism. Thus, high dietary methionine content spares cysteine and vice versa.
It is mainly being a methyl donor, see transmethylation and regeneration of cysteine from it
(with >16.3 - 100 μM/L homocysteinemia, in blood) are genetic defects in methionine metabolism. They are four types depending on site of the defect. Cystathionine synthase deficiency, defective B12 absorption and activation, and deficiency of methylene-FH4 reductase are all causes. They are due to accumulation of homocysteine produced from methionine after transmethylation. Homocysteine is converted into homocystine, which appears in urine at up to 300 mg/day, a condition called overflow aminoaciduria. S-adenosyl-methionine may appear in urine. There is mental retardation, hepatomegaly, dislocated eye lenses, skeletal deformities, premature osteoporosis, and intravascular thrombosis. Low-methionine highcysteine diet and large doses of folate, pyridoxine and B12 may improve certain subtypes of this case.
High blood homocysteine: Metabolic failure to convert homocysteine into cysteine or methionine leads to its accumulation in the blood. This is connected to long list of diseases including coronary artery disease, cerebrovascular disease, peripheral vascular disease and oxidative stress. It is lowered by dietary folate (provides methyl-FH4), B12 (provides methylcobalamin) and B6 (provides pyridoxal phosphate for cystathionine synthase and cystathionase), all activates homocysteine conversion into methionine and cysteine, respectively.
Hypermethioninemia: is due to deficiency of methionine-Sadenosyltransferase in the liver.
- Cysteine and cystine (dicysteine) are inter-convertible in the body by removal/addition of two hydrogens catalyzed by cystine reductase. They are glucogenic, sulfur-containing and non-essential amino acids synthesized in the body from methionine (as homocysteine) coupled with serine.
The major source of inorganic sulfate in the body is cysteine. Sulfate is used for sulfation reactions after activation into 3-phosphoadenosine-5- phosphosulfate (active sulfate or PAPS, 2 ATP + SO4 2- → PAPS + ADP + PPi) catalyzed by sulfotransferases. Sulfate detoxifies phenol or indole into phenyl sulfate or indoxyl sulfate (indican), respectively, that go to urine. Sulfated steroids and sulfated sugar derivatives are also produced by sulfation with PAPS.
Cysteine is transaminated (in presence B6) into R-mercaptopyruvate that loses sulfur as H2S catalyzed by transulfurase to give pyruvate. Or, cysteine is oxidized into cysteine-sulfinic acid in the liver and then transaminated to give 3-sulfinylpyruvate that is desulfinylated into sulfite (SO2 - ) and pyruvate. SO2 - is oxidized into SO4 2- by sulfite oxidase with lipoic acid and hypoxanthine, then sulfates go to urine or activated for sulfation. Or, cysteine is oxidized into
cysteine-sulfinic acid in the liver that is oxidized again into cysteic acid that is then transaminated into sulfonylpyruvate to be desulfonated into pyruvate and sulfate (SO4 2-). This cysteic acid is decarboxylated into taurine in presence of pyridoxal phosphate and GSH.
Desulfhydrase converts cysteine into pyruvate, H2S and ammonia, see nonoxidative deamination. It is decarboxylated into mercaptoethanolamine (that is a part coenzyme A structure) by decarboxylase and PLP. Thiosulfate produced from cysteine is used for detoxication of cyanide into thiocyanate and sulfite, (HCN + Na2S2O3 → HCNS + Na2SO3).
1. Synthesis of proteins: particularly scleroproteins and certain hormones, e.g., insulin and vasopressin.
2. Synthesis glutathione.
3. Synthesis of cystine: 2 molecules of cysteine give cystine.
4. Detoxication: Cysteine, as it is, reacts with aromatic compound, e.g., benzene, polycyclic hydrocarbons, e.g., naphthalene and ring halogenated hydrocarbons, e.g., bromobenzene that are then acetylated and go into urine.
Cysteine + Bromobenzene + acetic acid → Bromophenylmercapturic acid
Cysteine + naphthalene + acetic acid → Naphthaylmercapturic acid
5- Selenocysteine.
While not normally considered an amino acid present in proteins, selenocysteine occurs at the active sites of several enzymes.
Examples include the human enzymes thioredoxin reductase, glutathione peroxidase, and the deiodinase that converts thyroxine to triiodothyronine.
Unlike hydroxyproline or hydroxylysine, selenocysteine arises cotranslationally during its incorporation into peptides
. The UGA anticodon of the unusual tRNA designated tRNASec normally signals STOP. The ability of the protein synthetic apparatus to identify a selenocysteinespecific UGA codon involves the selenocysteine insertion element, a stem-loop structure in the untranslated region of the mRNA. Selenocysteine-tRNASec is first charged with serine by the ligase that charges tRNASer. Subsequent replacement of the serine oxygen by selenium involves selenophosphate formed by selenophosphate synthase.
Cystinosis (cystine storage disease):
-It is a lysosomal genetic disease characterized by the deposition of cystine crystals in many tissues especially reticuloendothelial system due to defective carrier-mediated transport of cystine. It is accompanied by impaired renal function and generalized aminoaciduria. The patient dies at young age from acute renal failure.
Cystinuria (cystine-lysinuria):
-It is a genetic disease characterized by marked increase in urinary cystine (2030 times normal) together with basic amino acids (lysine, arginine and ornithine). It is due to defective renal tubular reabsorption of these 4 amino acids with possible formation of cystine stones
✓ Key junction points ✓ Organ specialization and metabolic integration in a well fed state ✓ Metabolism in starvation
Definition: it is the coordination between metabolites (carbohydrates, lipids and proteins). ⚫ Insulin and glucagon are primary responsible for homeostasis of blood glucose level. ⚫ There is an additional regulation by thyroid, glucocorticoids and epinephrine hormones.
Significance of integration 1. Fuel supply for all tissues at all times. 2. under positive caloric balance, food energy intake is stored as glycogen or fat. 3. Under negative caloric balance, fatty acids glucose mainly for brain and RBCS that need glucose under all the conditions.
2) Fatty acid oxidation: fatty acid Acetyl CoA and energy is trapped in the form of NADH and FADH2 .
3) Amino acid degradation: Amino acid consumption, they are degraded to meet the fuel demands of the body. glucogenic aa glucose via pyruvate or TCA cycle intermediates. Ketogenic aa
oxidized synthesis synthesis acetyl coA
4) Citric acid cycle: acetyl CoA is the commonest metabolite it enters citric acid cycle and oxidized to CO2. Most of the energy is trapped as NADH and FADH2.
5) Oxidative phosphorylation: NADH and FADH2 are oxidized in electron transport chain, which is coupled with oxidative phosphorylation to generate ATP.
6) Gluconeogenesis: non-carbohydrates are the precursor.
7) Glycogen metabolism: glucose stored as glycogen in liver and muscles.
8) Hexose monophosphate shunt: liberation of NADPH utilized in biosynthesis of several compounds like fatty acids and ribose sugar (essential for nucleotides)
Key junction points phosphorylated Glucose-6-phosphate pyruvate
1. Glucose-6-phosphate: When glucose is transported into the cell glucose glucose-6-phosphate.
catabolized stored glycogen
Pentose phosphate pathway Ripose-5phosphate
NB: glucose-6-phosphate can be generated from glycogen or by gluconeogenesis.
2. Pyruvate :
transaminated Alanine
Glucose-6-phosphate glycolysis
pyruvate
reduced Lactate (anaerobic)
oxidixed
Carboxylated in mitochondria
Oxaloacetate (The first step of gluconeogenesis) oxidized
NB: Pyruvate acetyl CoA (irreversible step)
3. Acetyl CoA: the third junction point. it is activated 2 carbon unit produced by 3 ways;
-Oxidative decarboxylation of pyruvate.
-B-oxidation of fatty acids.
-Degradation of ketogenic amino acids.
⚫ Acetyl CoA is completely oxidized into CO2 in citric acid cycle, converted into HMG-CoA ketone bodies or cholesterol .
⚫
Pyruvate dehydrogenase complex in cytosol
Acetyl CoA fatty acids.
⚫ Tissues and organs work to meet the metabolic demand (usually 2-4 hours after food consumption)
- Liver is the metabolic hub of the body. It makes the fuel that supply the brain, muscles and other organs.
- Liver plays a central role in regulation of carbohydrate, lipid and amino acid metabolism.
1. Carbohydrate metabolism
- Liver removes about two third of glucose absorbed by intestine and converts it to glucose-6-phosphate.
glycolysis glycogen Ribose-5-phosphate
- Decreased gluconeogenesis.
2. Lipid metabolism: increased fatty acids and triacylglycerol synthesis.
3. Protein metabolism: increased catabolism of amino acids and increased protein synthesis.
Adipose tissue
⚫ Normally, free fatty acids are obtained from the liver for fat synthesis.
Because adipocytes lack glycerol kinase, they cannot recycle the glycerol from fat breakdown but must obtain glycerol-3-phosphate by reducing the DHAP (Dihydroxyacetone Phosphate) produced by glycolysis.
⚫ Adipocytes also need glucose to feed the pentose phosphate pathway for NADPH production.
⚫ Insulin is required for glucose uptake.
a. Increased glucose transport: stimulated by insulin (glucose transport)
b. Increased glycolysis: to provide glycerol phosphate for triacylglycerol synthesis
c. Increased activity in the HMP: To supply NADPH (essential for fat synthesis).
a. Increased synthesis of fatty acids: De novo synthesis of fatty acids from acetyl CoA
b. Increased triacylglycerol synthesis: Fatty acid + glycerol →triacylglycerol Adipocytes lack glycerol kinase, so that glycerol 3-phosphate used in triacylglycerol synthesis must come from the metabolism of glucose. Thus, in the well-fed state, elevated levels of glucose and insulin favor storage of TG.
c. Decreased triacylglycerol degradation: Insulin inhibits the hormonesensitive lipase (dephosphorylated form) and thus inhibits triacylglycerol degradation in the well-fed state.
a. Increased glucose transport: due to increase insulin. Glucose is phosphorylated to glucose 6-phosphate and metabolized to produce the energy needs of the muscle.
b. Increased glycogen synthesis: The increased insulin to glucagon ratio and the availability of glucose 6-phosphate stimulates glycogenesis.
Fatty acids are of secondary importance as a fuel for muscle in the well-fed state in which glucose is the primary source of energy.
a. Increased protein synthesis: An increase in amino acid uptake and protein synthesis occurs in the absorptive period after ingestion of a meal containing protein (stimulated by insulin).
b. Increased uptake of branched-chain amino acids: Muscle is the principal site for degradation of branched-chain amino acids. Leucine, isoleucine, and valine are taken up by muscle, where they are used for protein synthesis and as a source of energy.
Heart
Heart muscle differs from skeletal muscles in 4 ways:
1- The heart is continuously active, whereas skeletal muscle contracts intermittent on demand.
2- The heart has a completely aerobic metabolism.
3- The heart contains negligible energy stores such as glycogen or lipid. Thus, any interruption of the vascular supply results in rapid death of myocardial cells.
4- Heart muscle uses glucose, free fatty acid and ketone bodies as fuels
1. Carbohydrate metabolism: Glucose is the only source of fuel in an absorptive state. About 120 g of glucose is utilized per day. The brain contains no stores of glycogen, and is therefore completely dependent on the availability of blood glucose. If the blood glucose levels fall below approximately 30 mg /dl (normal blood glucose is 70-90 mg/dl) cerebral function is impaired.
2. Lipid metabolism: Free fatty acids cannot cross the blood-brain barrier; hence their contribution for the supply of energy to the brain is insignificant.
a. Increased glycogenolysis: several hours after a meal, blood glucose levels decrease stimulating the secretion of glucagon and inhibiting insulin secretion. The increased glucagon to insulin ratio stimulates glycogenolysis. Liver glycogen is nearly depleted after 10 – 18 hours of fasting. Thus hepatic glycogenolysis is a transient response to early fasting. Adult's liver contains 100 g of glycogen in the well -fed state.
b. Increased Gluconeogenesis: gluconeogenesis begins 4 –6 hours after the last meal and becomes fully active as liver glycogen stores are depleted. Gluconeogenesis plays an essential role in maintaining blood glucose during both overnight and prolonged fasting. The main sources for gluconeogenesis are amino acids, glycerol and lactate.
a. Increased fatty acid oxidation: The oxidation of fatty acids derived from adipose tissue is the major source of energy in hepatic tissue in the post absorptive state.
b. Increased Synthesis of Ketone bodies: The availability of circulating ketone bodies is important in fasting because they can be used as fuel by most tissues including brain, once their level in blood is sufficiently high. This reduces the need for gluconeogenesis from amino acids, thus slowing the loss of essential protein. Ketone body synthesize is favored when the concentration of acetyl CoA, produced from fatty acid oxidation exceeds the oxidative capacity of the tricarboxylic acid (TCA) cycle. Unlike fatty acids Ketone bodies are water –soluble, and appear in the blood and urine by the second day of a fast.
1. Carbohydrate metabolism: Glucose transport into the adipocyte and its metabolism is depressed due to low levels of blood insulin. This leads to a decrease in fatty acid and triacyl- glycerol synthesis.
a.Increased degradation of triacylglycerol's: The activation of hormone –sensitive lipase and subsequent hydrolysis of stored triacylglycerol are stimulated by high levels of epinephrine.
b.Increased release of fatty acids: Fatty acids resulting from the hydrolysis of stored triacylglycerol are released into the blood. Bound to albumin, they are transported to other tissues for use as fuel. Part of the fatty acids is oxidized in the adipose tissue to produce energy. The glycerol produced from triacylglycerol degradation is used by the liver for gluconeogenesis.
Exercising muscle initially uses its glycogen stores as a source of energy. During intense exercise, glucose -6-phosphate derived from glycogen is converted to lactate by anaerobic glycolysis. As these glycogen reserves are depleted, free fatty acids provided by the mobilization of triacylglycerol from adipose tissue become the major sources.
1. Carbohydrate Metabolism: Glucose transport and subsequent glucose metabolism is depressed because of low blood insulin.
2.Fat Metabolism: During the first 2 weeks of fasting, muscle uses fatty acids from adipose tissue and ketone bodies from the liver as fuels. After about 3 weeks of fasting, muscle decreases its utilization of ketone bodies and oxidize only fatty acids. This leads to a further increase in the already elevated levels of blood ketone bodies.
3.Protein Metabolism: During the first few days of starvation there is rapid breakdown of muscle protein, giving amino acids that are used by the liver for gluconeogenesis. Alanine and glutamine are quantitatively the most important glucogenic amino acids released from muscle. After several weeks of fasting, the rate of muscle proteolysis decreases due to a decline in the need for glucose as a fuel for brain
During the first two weeks of starvation, the brain mostly dependent on glucose, supplied by liver gluconeogenesis, depending on the amino acids released from the muscle protein breakdown. Starvation beyond three weeks results in increased plasma ketone bodies and the brain adopts itself to depend on ketone bodies for the energy. This decreases the need for protein catabolism for gluconeogenesis.
Adipose tissue
1. Depressed glucose transport to adipocytes (low insulin)
2. Decreased synthesis of fatty acids and triacylglycerol
1) Increased degradation of triacylglycerol
2) Increased release of fatty acid.
Skeletal muscle Brain
. Depressed glucose transport and metabolism (low insulin).
First 2 weeks: muscle uses fatty acid from adipose and ketone bodies from liver.
3 weeks: oxidize only fatty acids decreased ketone so the level of ketone is elevated.
First few days: rapid muscle protein breakdown so increased amino acids to go to liver for gluconeogenesis (alanine, glutamine)
Several weeks: decrease rate of muscle proteolysis.
First 2 weeks: dependent on glucose by liver gluconeogenesis
3 weeks: increased ketone bodies so the brain uses them for energy.
3 weeks: decreased the need of protein catabolism for gluconeogenesis.
✔ Pyrimidines and Purines
✔ Disorders of purine metabolism.
✔ DNA structure & organization.
Pyrimidine and purine are the names of the parent compounds of two types of nitrogen- containing heterocyclic aromatic compounds.
In DNA ⇾ Cytosine and Thymine.
In RNA ⇾ Cytosine and Uracil.
✓ Gout.
✓ Lesch Nyhan syndrome.
✓ Immunodeficiency associated with purine metabolism
✓ Infantile autism.
Metabolic disorders associated with overproduction of uric acid.
At physiological form, uric acid is found in more soluble form as sodium urate.
Normal serum uric acid range is 3-7 mg/dl.
In severe hyperuricemia, crystal of sodium urate gets deposited in the soft tissues, particularly in joints. Such deposits are commonly known as tophi.
This causes inflammation of joints resulting in gouty arthritis.
The prevalence of gout is about 3 per 1000 persons, mostly affecting males.
Post-menopausal women, however are as susceptible as men for this disease
Historically, gout was found to be associated with high living, over eating and alcohol consumption.
Lead poisoning also causes gout by decreasing uric acid excretion.
Types of Gout:
◖
Primary metabolic gout◗
◖
Primary renal gout◗
It is an inborn error of purine metabolism due to overproduction of uric acid.
Causes:
- Increased activity of PRPP synthetase
- Overactivity of PRPP amidotransferase.
- HGPRT deficiency.
- Glucose 6-phosphatase deficiency.
- Elevation of glutathione reductase
It is due to failure of uric acid excretion from the body so that uric acid level in the body gets increased.
◖
Secondary metabolic gout◗
Secondary gout is due to secondary to certain diseases like leukemia, polycythemia, lymphoma, psoriasis and increased tissue breakdown like in trauma, starvation etc.
◖
Secondary renal gout◗
It is due to secondary to defective glomerular filtration of urate due to generalized renal failure.
When uric acid is present in high concentrations in the blood, it may precipitate as a salt in the kidneys.
The salt can form stones, which can in turn cause pain, infection, and kidney damage.
Use of Colchicine & Uricosuric drugs.
✓ To remove urates from the joint, is the colchicine drug of choice.
✓ To remove the urates from the body, uricosuric drugs such as probenecid, sulfinpyrazone, salicylates etc. are used.
Use of Allopurinol.
✓ Inhibits the activity of enzyme xanthine oxidase as a result of which uric acid is not produced.
It is X linked metabolic disorder since the structural genes for HGPRT is located on the X chromosome.
It affects only males and is characterized by excessive uric acid production and neurological abnormalities such as mental retardation, aggressive behavior, learning disability etc.
✓ Allopurinol is used to treat hyperuricemia but it has no effect on the neurological manifestation in these patients.
✓ Treatment for the neuro-behavioral features is limited to behavioral therapy and providing protective physical device to prevent self-mutilation.
✓ Orotic aciduria
✓ Reye’s syndrome
✓ Primary structure.
✓ Secondary structure.
• DNA is a polynucleotide i.e., contains millions of nucleotides.
• Each nucleotide is formed of: (base, sugar and phosphate group).
• They are covalently linked to each other by 3`,5` phosphodiester bonds
The nitrogenous bases:
- They are heterocyclic compounds i.e.; the cycle consists of two or more different atoms (not only carbon).
- The nitrogenous bases that enter in RNA and DNA structure are either purines or pyrimidines.
Purine bases: a) Adenine b) Guanine c) Hypoxanthine d) Xanthine
e) Uric acid.
Pyrimidines bases: a) Uracil. b) Cytosine. c) Thymine.
Hypoxanthine, xanthine and uric acid are metabolic end products from adenine and guanine.
✓ Note that:
1. Bases with long name (pyrimidines) have small structure while those with short name (purines) have large structure.
2. Bases exist in two forms:
a. Ionized form that is called the keto (lactam) form
b. Unionized and called enol (lactim) form
Sugars:
- Sugars enter in the structure of nucleotides are either Ribose (in RNA) or Deoxyribose (DNA).
Nucleoside Base - Pentose
▪
They are formed by the attachment of C₁ of the sugar to the nitrogen 1 of pyrimidine and nitrogen 9 of purine
Nucleotide Base - Pentose - Phosphoric Acid
▪
They are formed by the attachment of -OH of phosphate to one of the -OH groups of the sugar.
➢ DNA exists as a double stranded molecule forming a double helix:
- The 2 strands of DNA molecule are antiparallel i.e., one strand runs in the 5` to 3` direction and the other strand runs in the 3` to 5` direction
➢ The double strands of DNA molecules are joined together according to pairing rule:
- Pairing rule: is the rule by which the bases of one strand of DNA are paired with the bases of the second strand so that an adenine (A) is always paired with thymine (T) (by two hydrogen bonds), while a cytosine (C) is always paired with guanine (G) (by three hydrogen bonds). Therefore, one strand of DNA double helix is always complementary and not identical to the other strand.
➢ The hydrophilic ribose phosphate molecules form the backbone outside the DNA molecule and the hydrophobic bases are arranged inside.
➢ Nucleotides of each of the two helical strands are bound to each other by covalent 3’-5’ phosphodiester linkage.
- C – 3’ –OH group of the ribose sugar of one nucleotide with the C – 5’ –OH group of ribose of the next nucleotide. This kind of bonding gives rise to a linear polydeoxyribonucleotide strand with 2 free ends on both sides.
➢ That end of the strand which bears a free 5’phosphate group without phosphodiester linkage is called the 5’-end.
➢ The opposite end bears a free 3’ – hydroxyl or 3’ phosphate group and is called the 3’ end.
Chromosomes:
- These are nucleoproteins, formed mainly of DNA and basic proteins.
- In man they are 46 in number.
Chromatin
It is the chromosomal material that is formed of a condensed DNA-protein complex.
The DNA found in a single human cell has a total length of 1 meter, and a typical human cell is only 20 micrometers long.
For DNA to be packed into such a small space, it must be condensed into a compact structure.
A number of proteins carry out this condensation and the condensed DNAprotein complex is called (chromatin).
Chromatin is consisted of:
✓ Very long double stranded DNA molecule.
✓ Histones: which are basic proteins.
✓ Protamines: which are also basic proteins.
✓ Small quantity of RNA.
- Histones are small proteins and are positively charged at physiological pH due to their high content of histidine, lysine and arginine.
- Because of their positive charges, the histones form ionic bonds with the negatively charged DNA.
Nucleosomes:
- The packaging unit of the chromatin is called nucleosome.
- Histones are arranged in structure units called nucleosome which resemble beads on string formed of DNA.
Genome
It is the total number of genes within one mature cell of an organism.
Genes are pieces of DNA, which occupy a specific position (locus) on it.
Each gene is a part of DNA sequences that contains genetic information coding for synthesis of one polypeptide (protein).
It's semi-conservative process involving synthesis of DNA from DNA
1)separation of the parental double stranded DNA helix into two single DNA strands occurs
2)each of them acts as a template upon which a new strand will be synthesized
3)each of the resulting two daughter double stranded DNA will contains old strand (one parent strand remained) and a new strand (synthesized from the free nucleotides present in the nucleus).
1. Helix unwinding protein “DNA helicase” : unwinds the double stranded DNA helix into two single stranded DNA through breakdown of hydrogen bonds between the nitrogenous bases (It utilizes ATP for each base pair break) forming replication fork “a V-shaped region” .
2. Helix destabilizing proteins “single stranded DNA binding proteins” :
1. bind to the two single stranded DNA (created by DNA helicase) keeping them separated & prevent their reannealing at the area of replication origin “Oric”, which is the site at which the DNA replication begins. 2. protect the DNA from nuclease which attacks the single stranded DNA.
C. Topoisomerases enzymes:
Two enzymes: Topoisomerase-I &Topoisomerase II. Function: prevent positive supercoiling which make further separation more difficult.
RNA Primer
D. Primase enzyme:
The DNA polymerase-III unable to assemble the first few nucleotides of the new strand using the parental DNA strand as a template & this assembly requires RNA primer. In eukaryotes, the RNA primer is synthesized by DNA polymerase α.
Function : joins the free 3’OH of C3 of one end of the chain made by the DNA polymerase-I to 5’OH of phosphate attached to OH of C5 of another end of the chain made by the DNA polymerase-III forming the phosphodiester linkage. It requires ATP.
Which when they enter in the formation of the new strand, they will loss 2 Pi & become dAMP, dGMP , dTMP , dCMP.
It include synthesis of:
Leading strand
Lagging strand
leading strand: lagging strand:
built in 5’-3’ direction towards the replication fork.
built in a continuous manner.
built in 3’-5’ direction away from the replication fork.
built in a discontinuous manner forming okazaki fragments which are small fragments of DNA.
contains few RNA primers.
contains many RNA primers.
RNA is found mainly in cytoplasm and is a single stranded polymer of ribonucleotides of adenine, guanine, cytosine and uracil instead of thymine. It contains ribose instead of deoxyribose. It is found in nucleus, nucleolus, cytoplasm and mitochondria. It is mainly of three types (beside the small nuclear RNA, snRNA, used in mRNA splicing) that differ in: gene of origin, function, size and structural modification.
- The initial single-stranded blue script of mRNA is called heterogeneous nuclear RNA (hnRNA) that after processing varies in size from 400 – 4000 nucleotides. - It is characterized in eukaryotes by having extensive post-transcriptional Modifications :
Firstly, the introns (the non-functional untranslatable internal sequences) are removed.
then a polyadenylate tail (20 – 250 base) is added at the 3'-end. Function: marks the mRNA for transportation to the cytoplasm
protects the mRNA from degradation by 3'-exonuclease.
7-methyl-guanosine triphosphate cap is added at the 5'-end of mRNA.
Function: directs the initiation of translation protects the mRNA from degradation by 5'-exonuclease.
- It also contains 5' and 3' terminal untranslated regions containing regulatory sequence for translation and mRNA half life.
- It is copied in the nucleus by transcription of DNA sequence of gene proper of specific gene on the base complementarity rule using the antisense strand of DNA as a template.
- It carries the genetic information (code, codons) for protein synthesis. Each amino acid is represented by three consecutive nucleotides sequence, i.e., the codon that is recognized by the anticodon of the tRNA.
The simplest and smallest RNA.
having less than 100 nucleotides length.
transcribed from specific genes that need to be post-transcriptionally processed to remove introns and its bases undergo extensive amount of modifications
characterized by the presence of several inter-chain hydrogen bonding that gives it a clover leaf like shape with three loops, an extra loop and free 3'-end and 5'-start.
carries the amino acids connected to the 3'-end and transfers them to the ribosomes for protein synthesis.
NB:
1- The 3'-ACC amino acid acceptor sequence is added after transcription.
2- each amino acid has its own tRNA, i.e., at least 20 types of tRNA exist.
It is the process of production of RNA copy from a specific region along the length of DNA, i.e., the gene proper.
The transcription unit is a sequence (stretch) of DNA which is formed of:
The gene enhancer/silencer: the regulatory gene response sequences that control the regulated rate of gene expression (more than the basal level).
The gene promoter region (transcription initiator sequence) : basal rate controlling sequence & a 5'3' regulatory DNA sequence up-stream the gene proper.
Transcribed region (gene proper) : the DNA sequence that is copied as hnRNA or other types of RNAs.
Termination region: a regulatory DNA sequence down-stream the gene proper of some genes, at which the RNA polymerase disassembles from the DNA template.
Between genes or islands of related genes there are insulator sequences that separate the coordinately regulated gene(s) so as regulatory sequences would work only on these isolated gene(s).
works in the same orientation of the gene reading frame (3'5').
located directly up-stream of the gene proper.
RNA polymerase binds DNA template at the gene promoter to initiates the basal rate of transcription.
Some promoters are weak and other strong (at which rate of transcription is much faster), nevertheless there are certain promoterless genes.
Prokaryotic promoter:
The region recognized by RNA polymerases.
Simpler than eukaryotic promoter
Formed of three parts:
i. Pribnow box: - It is a stretch of 6 nucleotides: 5'-TATAAT-3' - located 10 nucleotide up-stream the site of beginning of transcription.
ii. The -35 region: - It is a stretch of 8 nucleotides: 5'-TGTTGACA-3' - located about 35 nucleotide up-stream the site of beginning of transcription.
iii. The spacer stretches of nucleotides of about 10 nucleotides in between the transcrition start and Pribnow box & about 19 nucleotides in between the Pribnow box and the -35 region.
Both Pribnow box and the -35 region are recognized by RNA polymerases.
Eukaryotic Promoter:
More complex than the prokaryotic one
Formed of at least three parts:
i. Hogness or TATA box:
- It is a 6 stretch of nucleotides: 5'-TATAAA-3'. - located 25 nucleotide up-streams the site for beginning of transcription.
ii. CAAT box: - it is a 9 stretch of nucleotides; 5'-GGNCAATCT-3' - located about 70 to 80 nucleotide up-streams the site for start of transcription.
iii. The spacer stretches of DNA about 25 stretch of nucleotides between the transcrition start and TATA box and about 40 nucleotides between the CAAT box and TATA box.
They are transcription regulatory sequences.
May be remote from the gene.
By thousands of nucleotides up- or down-stream the gene proper or even within the gene proper.
Work in any orientation, i.e., 5'3' or 3'5'.
May activate transcription above the basal rate (Enhancer elements).
May suppress transcription below the basal rate (Silencer elements).
Examples for these elements are the hormone-response elements for steroid and thyroid hormones, vitamin D and vitamin A and other effectors. Activator or suppressor proteins that bind at these elements are called transcription factors and interact with RNA polymerases directly or indirectly through coactivator or corepressor proteins to increase or decrease rate of transcription.
Genes usually have several regulatory elements but some genes have none, since constitutionally transcribed genes have a constant rate of basal transcription.
A single type that is responsible for synthesis of all types of RNA
Products of RNA polymerase require slight or no modification after transcription.
The holoenzyme is formed of five subunits (α2ββ'σ) :
- Two identical α subunits
- Two similar but not identical ββ' subunits
- regulatory σ subunit
- The core part of the enzyme is formed of the four α2ββ' subunits.
There are three types; each is specific for synthesis of a specific type of RNA.
Mostly require extensive post-transcriptional modifications particularly mRNA.
They are much complex in structure and are formed of up to 16 subunits.
- RNA polymerase I: Responsible for synthesis of the large RNA molecules (rRNA) that is insensitive to α-amanitin (a mushroom toxin).
- RNA polymerase II: Responsible for synthesis of mRNA that is sensitive to low concentration of α-amanitin.
- RNA polymerase III: Responsible for synthesis of small RNA molecules, i.e., tRNA and 5S rRNA that is sensitive to high concentration of α-amanitin.
Initiation occurs on the template (non-coding) strand and it never occurs in the other strand, coding strand.
NB:
1- Template strand is single strand of a transcription unit (a gene) that is complementary to the RNA & is read in 3'5' so that the synthesized RNA will be formed in 5'3'.
2- Coding strand is similar to mRNA sequence except for U/T.
3- Which DNA strand is the template and which is the coding?
That differs from one gene to the other but is always the strand that contain the prompter sequence read in 3'5' direction.
RNA polymerase:
- recognizes the promoter region by the help of the σ (sigma) factor.
- Then, the core enzyme binds tightly to the DNA.
- Once the core molecule binds to the DNA, it unwinds 17 nucleotides to separate the two strands.
- The binding of the enzyme to DNA occurs in a sequential process, i.e., σ factor then core enzyme (α 2ββ') binds and it searches for the transcription initiation site (an open reading frame starting at TAC).
The synthesized RNA always starts with a purine that enters at the initiation site of the enzyme.
- This purine ribonucleotide: stays in prokaryotic mature mRNA removed in mature eukaryotic mRNA due to the post-transcriptional processing before capping.
When the second nucleotide enters at the elongation site of the enzyme, it forms a phosphodiester bond between 3'-OH group of ribose of the first nucleotide and the 5'-OH of the phosphate group on C5 of ribose of the second nucleotide.
- σ Factor is released before elongation starts.
- The four ribonucleotides triphosphate (ATP, GTP, CTP and UTP) continue to enter into the polymerization (or elongation) site of β unit with the release of a pyrophosphate (PPi) each time a new nucleotide is added to the growing RNA chain.
- The RNA polymerase continues transcription from 3' towards 5' end of the template strand according to the base pairing role in an anti-parallel manner so that A-U, G-C, T-A and C-G.
- Elongation continues till the termination point is reached.
- Termination may be rho (ρ) factor-dependent or independent.
In rho-dependent termination:
Rho factor recognizes and binds the termination sequence in the template DNA. Then, it disassembles the enzyme/RNA/DNA complex to release RNA polymerase and the synthesized RNA molecule from DNA template strand.
In rho-independent termination: RNA polymerase stops transcription when; the synthesized complete RNA molecule takes its three-dimensional form with the formation of specific secondary structures such as a hairpin followed by an oligo-U sequence
All this leads to pausing of RNA polymerase and thence disassembly of the transcription machinery.
Eukaryotic termination: may be more complex involves the polyadenylate tail addition signaled by specific AU sequence.
Rifamycin: Binds to the core enzyme occupying substrate binding site and inhibiting the incoming nucleotides from binding to the initiation site.
Actinomycin D: Binds DNA template inhibiting its transcription by preventing movement of RNA polymerase along DNA.
1
Eukaryotic crude transcript of mRNA produced in the nucleus is called heterogeneous nuclear RNA (hnRNA), i.e., the blue script.
Post-transcriptional Processing of mRNA include decreasing its size, 5'-capping and 3'-tailing along with the post-maturation mRNA editing.
A. Intron removal (Decrease in size):
It is due to the splicing or removal of internal non-translatable sequences (Introns) from the translatable sequences (Exons) by splicosome.
Introns separate functional domains of the gene and allow for genetic rearrangement.
Splicosome is an aggregation formed in the nucleus of hnRNA (the blue script of mRNA, tRNA or rRNA) + 4 small nuclear RNA (snRNAs; U1, U2, U5 and U4/U6) + more than 50 proteins.
The role of snRNAs is
1. bind each end of the intron by base pairing with each other
2. splice and remove the intron
3. rejoins ends of exons.
The enzymatic activity of the splicosome complex resides in the snRNAs that are hence called ribozymes (enzymes with RNA structure).
Removal of introns facilitates the transport of mature mRNA from the nucleus to the cytoplasm otherwise it will be degraded in the nucleus.
As an example, the gene for the endothelial NO synthase is 22kbp and has 26 exons and 25 introns. Exons give rise to a protein of 130 KDa and 1200 amino acid residues.
Alternative splicing gives rise to different mRNA and hence proteins from same initial transcript.
Inherited defect in splicing may lead to a disease, e.g., at least one form of β-thalassemia (with absent β-chain of hemoglobin) appears to result from a nucleotide change at an exon-intron junction that prevents proper splicing.
It is the addition of 7-methyl guanosine triphosphate to the 5'-end of mRNA by guanylate transferase (specific enzyme).
The cap is attached by 5' to 5' triphosphate linkage.
It enhances the translation of mRNA.
It protects mRNA from the action of 5'3' exonucleases and phosphatases.
Addition of 20 - 250 polyadenylate tail at the 3'-end by the action of poly-A-polymerase enzyme.
It is specified by a special sequence near the 3'-end of the mRNA (AAUAAA).
The tail protects 3'-end of mRNA from 3'5' exonuclease.
It facilitates mRNA transport into cytoplasm.
It is the modification of the mature mRNA sequence after its complete processing and transfer into cytoplasm leading to a change or truncation in the protein molecule.
Example is the apolipoprotein B100 (i.e., 1- 4536 amino acid residue, 100 KDa) that is the liver form of the complete mRNA and the B48 (i.e., 1 - 2152 amino acid residue, 48 KDa) that is the truncated intestinal form of the protein. The intestinal form of mRNA is produced due to deamination of cytosine at CAA codon of the amino acid residue number 2153 into uracil by intestine-specific cytosine deaminase. The UAA formed is a stop codon leading to truncation of the B100 into B48 size.
not only controlled by the above-mentioned strategies.
Also controlled by specific sequences in 5' and 3' untranslated regions of mRNA.
Regulatory proteins bind at these sequences.
Example is the control of iron transport and storage by regulating the half-life of the mRNAs of iron transporters and binding proteins by the iron-regulated proteins.
tRNA transcript is a large precursor containing more than one tRNA. It is processed by the action of specific class of ribonucleases that also include removal of introns and cleavage of a 5'-leader sequence.
• Proteins are amino acid polymers which are synthesized in cytoplasm, endoplasmic reticulum and/or mitochondria. • There are various types of proteins with different function and structure due to the amino acid sequence in their constituting polypeptides. Each type of protein is produced by certain cell utilizing one gene or more.
• It is catalyzed by Amino-acyl-tRNA synthetase enzyme by attaching a specific amino acid to a specific tRNA.
• This activation requires ATP which is hydrolyzed into AMP+PPi
• elF-1 dissociates the complete 80S ribosome into 60S & 40S ribosomal subunits.
• elF-3 prevents their reassociation.
• 40S ribosomal subunit then bind to elF-1&-3.
• elF-2 bounding to GTP will binds and activates Met-tRNA to binds to (40SelF-1-elF-3) complex forming 43S pre-initiation complex.
• So, 43S pre-initiation complex is formed of: elF-1+ elF-2+ elF-3 + 40S ribosomal subunit + GTP + Met-tRNA
• elF-4 binds to mRNA activating it to binds to 43S pre-initiation complex with hydrolysis of ATP into ADP + Pi to forms the 48S pre-initiation complex which is formed of : elF-1-elF-2-elF-3-elF-4 + 40S subunit + GTP + Met-tRNA + mRNA
• The 48S pre-initiation complex scans the mRNA till reach translation initiation codon for methionine which is AUG, so protein synthesis always starts with methionine as N-terminal amino acid.
• elF-5 activates the binding of 60S ribosomal subunit to 48S pre-initiation complex with hydrolysis of the GTP bounding to elF-2 into GDP + Pi and release of all elFs forming the complete 80S initiation complex.
Requirements:
• New amino acyl-tRNA
• GTP as a source of energy
• Peptidyl transferase which is needed for peptide bond formation.
• Translocase which moves the ribosome three nucleotide bases on the mRNA towards its 3’end.
• eEF-1α & eEF-2
Steps:
• The eEF-1α binds with a new amino-acyl-tRNA and enable it to enter the ribosome according to codon anticodon recognition with hydrolysis of GTP into GDP + Pi and release of eEF-1α which recycles.
• The free NH2 group of the new amino acid binds with the free COOH group of the first amino acid (Met) catalyzed by peptidyl transferase then release of uncharged tRNA (free tRNA without amino acid).
• Then the ribosome moves three nucleotide bases on the mRNA towards the 3’end (translocation) with the help of translocase enzyme, GTP & eEF-2.
• It occurs when the ribosome moves to bring one of the three termination codons (UAA or UAG or UGA) as no tRNA can recognizes these codons but eukaryote releasing factors (eRF_1 -2 -3) can recognize all the three termination codons.
• Releasing factors activate the peptidyl transferase to hydrolyze and release the peptide chain on tRNA, then release of mRNA from 80S ribosome, this requires hydrolysis of GTP into GDP + Pi.
Point Mutations
These are a single base changes where there is substitution (replacement) of one base for another and it may be transitions or transversion
➢ Transition mutations: One pyrimidine is changed to the other pyrimidine or one purine is changed to the other purine.
➢ Transversion mutation: One purine is changed to either of the 2 pyrimidines or one pyrimidine is changed to either of the 2 purines.
1- Silent mutation: the changed base leads to a codon, which produces the same amino acid. In this case, the change lies in the third base of the codon, which has several alternative names for same amino acid.
2-Missense mutation: the change occurred either in first or second base of the codon producing a different amino acid.
3-Non-sense mutation: the altered base results in a non-sense termination codon. This leads to pre -mature stopping of protein synthesis leading to truncated protein.
This type of mutation results from either deletion or addition of one or more nucleotide(s) in DNA that generates altered mRNA.
1-Garbled translation of the mRNA distal to a single nucleotide deletion
2-Premature termination of polypeptide due to appearance of nonsense codon near the -COOH terminus.
3-Addition or deletion of amino acid to the Protein. If 3 nucleotides were inserted or deleted, this results in a protein in which one amino acid was missed or added.
- Used to correct many types of DNA damage that affects only one strand. It has three mechanisms as follows: A. Nucleotide excision repair of thymine-thymine(or pyrimidine) dimer: Thymine-thymine dimer is covalent linkage of two adjacent thymine bases in a single strand and occurs spontaneously, or due to chemical, radiation, or ultraviolet (UV) light damage to DNA segment. These dimers prevent DNA polymerase from replicating DNA strand beyond the site of the dimer.
➢ This enzyme recognizes the dimer and makes a nick in the affected DNA strand 8 nucleotides away from the dimer site to the 5'-side.
➢ DNA polymerase I fills the gap with new nucleotides using the healthy strand as a template in the 5' 3' direction by nick translation.
➢ Then, UV- endonuclease cut the free damaged specific sequence 4 nucleotides from the dimer site away to the 3'-side.
➢ Then, the damaged piece of DNA diffuses away.
➢ Then, DNA ligase joins the 3' end of the new DNA and the 5' end of the original DNA.
B. Mismatch Repair: Mismatch, e.g., G-T, is due to copying errors during replication.
➢ The mismatch is recognized by a group of proteins called MutS, MutL and MutH that identify the parent DNA strand by its methylation. Once identified the mismatch, they cut the defective DNA strand.
➢ A special exonuclease (exonuclease 1) hydrolyzes DNA in 3' 5' direction to a few nucleotides 5' the mismatch and releases free DNA nucleotides.
➢ A new DNA is synthesized to fill the gap by DNA polymerase III and DNA ligase joins the 3'-end of the new DNA and the 5'-end of the DNA ahead of it.
➢ Oxidative deamination of cytosine into uracil, adenine into hypoxanthine and guanine into xanthine occurs spontaneous, or due to chemical or radiation damage to a single base.
➢ Since uracil, hypoxanthine and xanthine are foreign to DNA; they are recognized and removed from the DNA strand by specific-DNA glycosylase that cuts the bond between the deoxyribose and the base leaving apyrimidinic or apurinic site, i.e., AP.
➢ An AP-endonuclease cuts the deoxyribose-phosphate backbone of the affected strand at 5' end of the AP site and removes a few nucleotides.
➢ Then, DNA polymerase I excise the residual deoxyribose phosphate unit and inserts cytosine, as dictated by the presence of guanine on the undamaged complementary strand.
➢ Then, the deoxyribose-phosphate backbone is rejoined by a Ligase
The figure illustrates the mechanism of repair of double DNA strand breaks that are due to ionizing radiation, chemotherapy and oxidative free radicals.
Two proteins are involved; Ku and DNA-dependent protein kinase. Both of them attach to each end of the double strand break, activate one another other, unwind the duplex (Ku has helicase activity) and search for the closest DNA sequence of minimum complementarity in the opposite strands. The extranucleotide tails are degraded into free nucleotides by exonuclease and the gaps are filled by DNA polymerase III and DNA ligase seals the free ends.
It is an autosomal recessive disease. It is an example of a defective mechanism for the repair of pyrimidine dimers in DNA. It is due to absence of the UV-specific endonuclease enzyme and defective correction of thymine dimer. Patients are highly sensitive to sunlight or UV light and their skin cells contain multiple thymine dimer. They develop skin cancer.
It is an autosomal recessive disease. Ataxia and malignant neoplasms of the reticuloendothelial system are the major symptoms. There is impaired repair of DNA strand breaks leading to increased sensitivity to X-rayinduced damage.
It is an autosomal recessive anemia. It is associated with increased susceptibility to malignant neoplasms. It is due to failure of correction of cross-linkage damage, e.g., thymine-thymine dimer.
It is Lynch syndrome in which patients develop cancer in the colon with cancer also in stomach and/or uterus. It is due to defective DNA mismatch repair due to mutations in two genes, hMSH2 and hMLH1 ( human analogs of MutS and L ).
✔ Transcriptional control of gene expression
✔ Post-Transcriptional control of gene expression
✔ Epigenetic control of gene expression
✔ Gene rearrangement
Gene expression is controlled at four levels:
At transcription level.
B. At post transcription level.
C. At epigenetic level.
D. Gene rearrangement.
• Each differentiated cell has the capacity to synthesize certain types of protein in suitable amounts through differential regulation of gene expression.
• Regulation of gene expression can be illustrated by the Lac-Operon model in E. Coli as the simplest model for regulation of gene expression since regulation of other genes in E. Coli or eukaryotes are far more complex.
• Operon is a coordinated unit of gene expression (Lac = lactose- metabolizing enzymes, Oper = operation, and On = is on).
• Lac-Operon is formed of two genes with their regulatory sequences. One gene is constitutively regulated regulatory gene, the LacI-gene, i.e., Lac-gene inhibitory gene that gives a monocistronic mRNA translated into the repressor
protein.The other gene is structural, Lac-gene formed of three units (Z, Y and A) and gives a polycistronic mRNA translated into three proteins (β-galactosidase, lactose permease and acetylase).
• E. Coli can metabolize glucose, glycerol, lactose or galactose as a source of energy with preference of glucose.
• For the integrated metabolism of lactose the bacterium requires lactose permease that permits entry of lactose into the cells and β-galactosidase that hydrolyzes lactose into glucose and galactose. They are co-regulated since they are produced as one polycistronic mRNA along with a third enzyme of unknown function, i.e., acetylase (it may acetylates specific proteins and facilitate gene induction).
• Since Lac-gene is an inducible gene, in presence of glucose the bacterium contains a few molecules of these enzymes per cell. But when the media lacks glucose, the bacterium expresses this gene at a very high rate (as much as 100-fold the uninduced rate) producing large amount of these enzymes per cell to increase lactose metabolism.
• Jacob and Monod elucidated the mechanism of the coordinated regulation of the expression (induction/repression) of that Operon in 1961.
• As above, the Lac-gene has a promoter sequence downstream of which there is the Operator sequence at which Lac repressor binds to hinder the binding of RNA polymerase to the promoter, and upstream of which there is the CAP sequence at which the catabolite-activated DNA-binding protein (CAP, cAMP-dependent) binds to activate binding of RNA polymerase.
• The regulatory Lac repressor DNA-binding protein is the product of Lac repressor gene (Lac-I gene) which has the promoter as the only regulatory sequence and is a regulatory gene with a constitutive constant rate of transcription and hence protein synthesis.
The two states of Lac-Operon (Repression and induction)
• When E. Coli is grown in presence of glucose, transcription of the Lac-gene is repressed. Repression is mediated by Lac repressor, which binds as a tetramer to the operator sequence preventing the binding of RNA polymerase to the promoter and prevents the transcription of the Lac-gene. The repressor is a negative regulator, and its sequence is a silencer on expression of the Operon, see the following figure.
• When glucose is absent, the Lac-gene is induced, i.e., expression rate of the Operon is increased. Starvation of the bacterium of glucose leads to increase of cAMP that binds and activates the DNA binding of the Catabolite gene Activator Protein (CAP). The CAP binds to the CAP binding sequence of DNA up-stream the promoter to facilitate binding of RNA polymerase to the promoter to induce transcription of the Lac-gene at a low level. Therefore, cAMP-activated CAP is a positive regulator, and its sequence is an enhancer.
• The produced small amount of permease facilitates entrance of lactose into the cell. Lactose (an inducer) or its non-metabolizible analogs (e.g., isopropylthiogalacoside, a gratuitous inducer) have high affinity to bind the repressor causing a change in its conformation. This change prevents the repressor from binding to the operator. Therefore, lactose acts as an inducer and the operator becomes free for higher rate of transcription by RNA polymerase.
of gene expression
Gene expression is also regulated at the levels of RNA processing (e.g., capping, tailing, alternative splicing and editing), RNA transport, mRNA half-life and rate of translation (e.g., proteins utilized in iron absorption, transport and storage).
• Gene expression is also regulated by epigenetic factors such as the spatial cell-cell and cell-matrix contact through mechanisms that include methylation at cytosine of specific CpG islands near the promoter region of genes (hyperand hypo-methylation) and chromatin remodeling. Environmental agents, e.g., alkylating pollutants may work by modulation of such methylation and
Post-Transcriptional control of gene expression:
Epigenetic control of gene expression
remodeling. An example: is the inactivation of most of the gene contained in the inactive female X chromosome as a form of genomic imprinting.
• It is due to one of the following events :
1. Cross-over is a recombination between homologous chromosomes during meiosis with equal or unequal reciprocal exchange of genetic material. The later results in diseases due deletion/insertion mutation.
2 . Sister chromatid exchange.
3. Differential rearrangement, i.e., for the VL and CL genes for a single immunoglobulin molecule that are widely separated in the germ line DNA and upon maturation of plasma cell they move and become closer together.
4. Transposition, transposons are small DNA elements that are capable of transposing themselves in and out of the host genome and may affect the function of the neighboring sequences. This occurs through an RNA intermediate utilizing reverse transcriptase.
5. Gene conversion by exchange of DNA sequences between homologous or non-homologous chromosomes after being duplicated and become similar in these chromosomes.
6. Integration of oncogenic viruses.
7. Gene amplification, due to repeated initiation during DNA replication that increases the gene copy number and dosage, e.g., genes for eggshell formation during oogenesis are amplified and also in cancer cells (See cancer biology).